Cardiologists and oncologists today face the daunting challenge of identifying patients at risk for late-onset left ventricular (LV) systolic dysfunction from the use of various chemotherapeutic agents. Currently, the most widely used method in clinical practice for monitoring the potential of chemotherapy-induced cardiotoxicity is calculation of LV ejection fraction. The use of LV ejection fraction to determine whether to continue or discontinue the use of chemotherapeutic agents is limited, because decreases in LV ejection fraction frequently occur late and can be irreversible. These limitations have led to the exploration of diastolic function and newer modalities that assess myocardial mechanics to identify sensitive and specific variables that can predict the occurrence of late systolic function. The cancer therapies associated with cardiotoxicity are reviewed in this report. Additionally, the authors evaluate the role of present-day echocardiographic parameters, complementary noninvasive imaging modalities, and biomarkers in the prediction of cardiotoxicity. The authors address the evolving role of cardioprotective agents and potential therapies to prevent or reverse the progression of LV systolic dysfunction. Finally, they provide some ideas regarding future directions to enhance the knowledge of predicting late-onset LV systolic dysfunction secondary to cancer therapy.
Attention ASE Members:
ASE has gone green! Visit www.aseuniversity.org to earn free CME through an online activity related to this article. Certificates are available for immediate access upon successful completion of the activity. Non-members will need to join ASE to access this great member benefit!
Cancer Therapies and Cardiotoxicity
Systemic anticancer therapies include different classes of drugs with variable mechanisms and targets of action. Conventional chemotherapy is classically represented by antiproliferative actions and includes alkylating agents (cyclophosphamide), platinum-based drugs (cisplatin), antimetabolites (methotrexate, 5-fluorouracil, capecitabine), microtubule agents (vinca alkaloids, taxanes), antibiotics (anthracycline, actinomycin D, bleomycin). Alternative anticancer strategies are available, including hormone therapy (tamoxifen) and immunotherapy (most commonly monoclonal antibodies). Also, recent molecularly targeted agents, principally protein tyrosine kinase inhibitors, are available. The classification of chemotherapy-induced cardiotoxicity is not defined in a universally accepted schema. The classification of the type 1 and type 2 toxicities has been proposed and is the classification we will define and use.
Many chemotherapeutic agents are associated with cardiotoxicity that manifests as LV systolic dysfunction. In particular, anthracycline and trastuzumab-induced cardiotoxicities are well described.
Type 1 Chemotherapy-Mediated Cardiotoxicity
Anthracycline-induced cardiotoxicity, type 1 chemotherapy-related cardiac dysfunction, is typically dose related and irreversible, associated with microscopic ultrastructural changes, and frequently results in myocardial cell death. It is due, at least in part, to oxidative stress on cardiac myocytes resulting in free radical formation and cell death. Chemotherapeutic agents classified as causing type 1 chemotherapy-related LV systolic dysfunction include doxorubicin, epirubicin, idarubicin, liposomal anthracyclines, cyclophosphamide, and docetaxel (see Table 1 ). In patients with cancer who develop asymptomatic or symptomatic anthracycline-induced cardiotoxicity, LV ejection fraction (LVEF) recovery and cardiac event reduction can occur if there is early detection and treatment with modern HF therapy.
Drug | Cardiotoxicity |
---|---|
Mitoxantrone | Increased risk if current or prior heart disease |
Increased risk if prior treatment with anthracycline | |
Increased risk if prior treatment with radiation therapy | |
Contraindication to use if LVEF < 50% | |
Cyclophosphamide (>150 mg/kg) | 7%–28% risk for HF 1–10 days after first dose |
Ifosfamide | 10%–30% risk for HF |
Mitomycin | Risk for hemorrhagic pericarditis, tamponade, myocarditis, myocardial infarction, and cardiomyopathy |
Docetaxel | 2%–8% risk for HF |
Paclitaxel | Risk for hypotension, bradycardia |
Synergistic effect with anthracycline metabolites yielding HF |
Type 2 Chemotherapy-Mediated Cardiotoxicity
Alternatively, trastuzumab-induced cardiotoxicity, type 2 chemotherapy-related cardiac dysfunction, typically is not dose related and can be associated with reversible myocardial dysfunction rather than structural damage. Reversibility is defined as recovery of LVEF to the normal range. A small study revealed that 60% of patients (25 of 42) who developed trastuzumab-induced cardiotoxicity recovered to normal LVEFs after discontinuation of trastuzumab and initiation of HF therapy. Chemotherapeutic agents classified as causing type 2 chemotherapy-related LV systolic dysfunction include trastuzumab, lapatinib, sunitinib, imatinib, and bevacizumab (see Table 2 ). These differences are fundamental to the dilemma the medical community faces of whether the benefits of chemotherapies outweigh the associated risks for the treatment of life-threatening cancers. However, type 1 and 2 cardiac dysfunctions can coexist in the same patient.
Drug | Cardiotoxicity |
---|---|
Lapatinib | 1% risk for asymptomatic cardiac events |
Reversible decrease in LVEF | |
Cardiac events not influenced by prior treatment with anthracycline or trastuzumab | |
Sunitanib | Risk for hypotension |
10%–30% LVEF decrease | |
Increased risk if history of CAD or CV risk factors | |
Bevacizumap | 2%–3% risk for LV systolic dysfunction, especially in elderly with CV risk factors |
Anthracyclines and Trastuzumab: Two Widely Used Chemotherapeutic Agents
Anthracycline-induced cardiotoxicity is classified on the basis of clinical findings as (1) acute, (2) early-onset chronic progressive, or (3) late-onset chronic progressive. Acute anthracycline-induced cardiotoxicity represents 1% of cases; it can occur hours or days after infusion of the drug, is not dose related, and is usually reversible. Acute anthracycline-induced cardiotoxicity is not a predictor of the future development of HF. Early-onset chronic progressive anthracycline-induced cardiotoxicity occurs during therapy or within 1 year after therapy and, generally, is not reversible. Late-onset chronic progressive anthracycline-induced cardiotoxicity manifests ≥1 year after therapy. The two chronic forms of anthracycline-induced cardiotoxicity are dose related and present as dilated cardiomyopathy and, frequently, HF. The limiting dose of anthracycline for each patient is determined by age, cardiovascular risk factors, prior radiation dose, coexisting drug therapy, type of drug, drug schedule, and, most importantly, cumulative dose (see Table 3 ).
Cumulative dose (mg/m 2 ) | Incidence |
---|---|
300 | <3% |
400 | 3%–5% |
550 | 7%–26% |
700 | 18%–48% |
Trastuzumab is a widely used chemotherapeutic agent that causes cardiotoxicity. It is a monoclonal antibody binding erythroblastic leukemia viral oncogene homolog 2 gene and human epidermal growth factor receptors 2, and widely used in erythroblastic leukemia viral oncogene homolog 2–positive breast cancer. This agent, in contrast to anthracyclines, does not provoke myocardial necrosis but causes myocardial cell dysfunction that is frequently reversible. The incidence of trastuzumab-induced cardiac dysfunction varies from 2% to 10% but can be up to 27% when used in combination with anthracycline and cyclophosphamide. The cumulative effect of these chemotherapeutic agents on cardiac function is critical to treatment decisions.
The challenge the cardiology community faces is the development of parameters to identify early changes in myocardial function that will predict future cardiotoxicity. One of the most important tools for defining myocardial function is echocardiography.
Cancer Therapies and Cardiotoxicity
Systemic anticancer therapies include different classes of drugs with variable mechanisms and targets of action. Conventional chemotherapy is classically represented by antiproliferative actions and includes alkylating agents (cyclophosphamide), platinum-based drugs (cisplatin), antimetabolites (methotrexate, 5-fluorouracil, capecitabine), microtubule agents (vinca alkaloids, taxanes), antibiotics (anthracycline, actinomycin D, bleomycin). Alternative anticancer strategies are available, including hormone therapy (tamoxifen) and immunotherapy (most commonly monoclonal antibodies). Also, recent molecularly targeted agents, principally protein tyrosine kinase inhibitors, are available. The classification of chemotherapy-induced cardiotoxicity is not defined in a universally accepted schema. The classification of the type 1 and type 2 toxicities has been proposed and is the classification we will define and use.
Many chemotherapeutic agents are associated with cardiotoxicity that manifests as LV systolic dysfunction. In particular, anthracycline and trastuzumab-induced cardiotoxicities are well described.
Type 1 Chemotherapy-Mediated Cardiotoxicity
Anthracycline-induced cardiotoxicity, type 1 chemotherapy-related cardiac dysfunction, is typically dose related and irreversible, associated with microscopic ultrastructural changes, and frequently results in myocardial cell death. It is due, at least in part, to oxidative stress on cardiac myocytes resulting in free radical formation and cell death. Chemotherapeutic agents classified as causing type 1 chemotherapy-related LV systolic dysfunction include doxorubicin, epirubicin, idarubicin, liposomal anthracyclines, cyclophosphamide, and docetaxel (see Table 1 ). In patients with cancer who develop asymptomatic or symptomatic anthracycline-induced cardiotoxicity, LV ejection fraction (LVEF) recovery and cardiac event reduction can occur if there is early detection and treatment with modern HF therapy.
Drug | Cardiotoxicity |
---|---|
Mitoxantrone | Increased risk if current or prior heart disease |
Increased risk if prior treatment with anthracycline | |
Increased risk if prior treatment with radiation therapy | |
Contraindication to use if LVEF < 50% | |
Cyclophosphamide (>150 mg/kg) | 7%–28% risk for HF 1–10 days after first dose |
Ifosfamide | 10%–30% risk for HF |
Mitomycin | Risk for hemorrhagic pericarditis, tamponade, myocarditis, myocardial infarction, and cardiomyopathy |
Docetaxel | 2%–8% risk for HF |
Paclitaxel | Risk for hypotension, bradycardia |
Synergistic effect with anthracycline metabolites yielding HF |
Type 2 Chemotherapy-Mediated Cardiotoxicity
Alternatively, trastuzumab-induced cardiotoxicity, type 2 chemotherapy-related cardiac dysfunction, typically is not dose related and can be associated with reversible myocardial dysfunction rather than structural damage. Reversibility is defined as recovery of LVEF to the normal range. A small study revealed that 60% of patients (25 of 42) who developed trastuzumab-induced cardiotoxicity recovered to normal LVEFs after discontinuation of trastuzumab and initiation of HF therapy. Chemotherapeutic agents classified as causing type 2 chemotherapy-related LV systolic dysfunction include trastuzumab, lapatinib, sunitinib, imatinib, and bevacizumab (see Table 2 ). These differences are fundamental to the dilemma the medical community faces of whether the benefits of chemotherapies outweigh the associated risks for the treatment of life-threatening cancers. However, type 1 and 2 cardiac dysfunctions can coexist in the same patient.
Drug | Cardiotoxicity |
---|---|
Lapatinib | 1% risk for asymptomatic cardiac events |
Reversible decrease in LVEF | |
Cardiac events not influenced by prior treatment with anthracycline or trastuzumab | |
Sunitanib | Risk for hypotension |
10%–30% LVEF decrease | |
Increased risk if history of CAD or CV risk factors | |
Bevacizumap | 2%–3% risk for LV systolic dysfunction, especially in elderly with CV risk factors |
Anthracyclines and Trastuzumab: Two Widely Used Chemotherapeutic Agents
Anthracycline-induced cardiotoxicity is classified on the basis of clinical findings as (1) acute, (2) early-onset chronic progressive, or (3) late-onset chronic progressive. Acute anthracycline-induced cardiotoxicity represents 1% of cases; it can occur hours or days after infusion of the drug, is not dose related, and is usually reversible. Acute anthracycline-induced cardiotoxicity is not a predictor of the future development of HF. Early-onset chronic progressive anthracycline-induced cardiotoxicity occurs during therapy or within 1 year after therapy and, generally, is not reversible. Late-onset chronic progressive anthracycline-induced cardiotoxicity manifests ≥1 year after therapy. The two chronic forms of anthracycline-induced cardiotoxicity are dose related and present as dilated cardiomyopathy and, frequently, HF. The limiting dose of anthracycline for each patient is determined by age, cardiovascular risk factors, prior radiation dose, coexisting drug therapy, type of drug, drug schedule, and, most importantly, cumulative dose (see Table 3 ).
Cumulative dose (mg/m 2 ) | Incidence |
---|---|
300 | <3% |
400 | 3%–5% |
550 | 7%–26% |
700 | 18%–48% |
Trastuzumab is a widely used chemotherapeutic agent that causes cardiotoxicity. It is a monoclonal antibody binding erythroblastic leukemia viral oncogene homolog 2 gene and human epidermal growth factor receptors 2, and widely used in erythroblastic leukemia viral oncogene homolog 2–positive breast cancer. This agent, in contrast to anthracyclines, does not provoke myocardial necrosis but causes myocardial cell dysfunction that is frequently reversible. The incidence of trastuzumab-induced cardiac dysfunction varies from 2% to 10% but can be up to 27% when used in combination with anthracycline and cyclophosphamide. The cumulative effect of these chemotherapeutic agents on cardiac function is critical to treatment decisions.
The challenge the cardiology community faces is the development of parameters to identify early changes in myocardial function that will predict future cardiotoxicity. One of the most important tools for defining myocardial function is echocardiography.
Role of Echocardiography
The role of echocardiography in the detection and prediction of chemotherapy-induced cardiotoxicity is evolving. References to standard measures of ventricular systolic and diastolic functions as well as newer modalities of assessing myocardial mechanics are summarized in Table 4 and discussed in detail below.
Study | Patients ∗ | Drug | Follow-up echocardiography | Parameters | Early/late change | LVEF ↓ | Predictive value |
---|---|---|---|---|---|---|---|
Stoddard et al. (1992) | 26 | Dox > 200 mg (+ others) | Pre; each dose (3 wk) up to 3 mo after last dose | Volumes, IVRT, E, E/A, DT | Early: 3 mo ↑ IVRT and DT | 9/26 (35%) | IVRT (↑37%); sens, 78%; spec, 88% |
Tassan-Mangina et al. (2006) | 20 | Dox 211 mg/m 2 | Pre; 1–3 mo; 3, 5 y | E, A, E/A, DT, IVRT, DTI S′, e′, a′, IVRT | Early: 3 mo ↓ DTI IVRT, E, e’, E/A Late: 3, 5 y both systolic and diastolic parameters (↓S′, LVEF) | 4/16 (25%) | IVRT (↓); sens, 100%; spec, 91% |
Ganame et al. (2007) | 13 children | Dox, dauno, ida; 3 doses, 30–75 mg/m 2 (low to moderate) | Pre; <2 h after each dose | LV mass, FS, LVEF, MAPSE, MPI, E, A, E/A, DT, IVRT, E/e′, IVA, S, D, A pulm, A dur, DTI S′, e′, a′, DTI long and rad strain, SR, global, regional | Early: after 1st dose, ↑MPI, E, A, E/A, IVRT, ↓e′ rad and long, ↓rad and long strain, SR After 2nd and 3rd doses, ↓S′ rad and long; ↓↓rad strain, SR; ↓FS, LVEF (still normal) | — | — |
Ganame et al. (2007) | 56 children | Dox, dauno, ida 240 mg/m 2 | 5 y after last dose | LV mass, FS, LVEF, MAPSE, TAPSE, MPI, E, A, E/A, IVRT, S, D, A dur, DTI S′, e′, a′, e′/a′, IVRT, IVA, strain, SR each wall segment | Late: ↑MPI; ↓MAPSE, IVA basal lateral ↓S, D; ↑IVRT; ↓rad and long strain, SR | — | — |
Dodos et al. (2008) | 100 | Dox, dauno, ida, epi, mitoxantrone 226 mg/m 2 mean dose | Pre; 24–72 h; 1, 6, 12 mo after last dose | FS, LVEF, MPI, E, A, E/A, A dur, DT, IVRT, IVCT, S, D, A, A dur | Early: 24–72 h after, ↓FS, LVEF (still normal) 1 mo after, ↑MPI (67% of pts) Late: 6 mo, ↓E/A; 12 mo after, ↓↓FS, LVEF | 15/100 (15%) | — |
Stapleton et al. (2007) | 151 | Anthra 200 ± 100 mg/m 2 | 8 mo after therapy | FS, MPI, IVRT, IVCT, E, A, S′, e′, a′, e′/a′, E/e′ | Late: 8 mo ↓E/A, e′/a′, ↑a′, ↓e′ (only septal) For doses >200 mg/m 2 , ↓FS | ||
Jurcut et al. (2008) | 16 | PL-dox (30 mg/m 2 ) + cyclophosp every 3 wk | Pre; after 3 and 6 cycles | LVEF, E, A, IVRT, DT, MAPSE; S, D, A pulm, DTI strain, SR, long and rad V | Early: 3 cycles ↓S rad Late: 6 cycles ↓S long, SR long, SR rad (not V) | — | — |
Hare et al. (2009) | 35 | TZM after others | Pre; 3, 6, 9, 12 mo | 2D, 3D LVEF; DTI e′, long strain, SR; STE long and rad strain, SR | 3–6 mo ↓DTI long SR ↓DTI rad SR 6–9 mo ↓STE long SR, ↓STE rad SR (long earlier than rad) | 9/35 (26%); only 1 LVEF <50% | Long SR (in 51% of pts) identified 3 pts with ↓LVEF after 1 y |
Cadeddu et al. (2010) | 49 | Epi 400 mg with placebo vs telmisartan | Pre; 1 wk after each 100-mg dose (T1–T4) | E, A, E/A, DT, DTI S′, e′, a′, long strain, SR | 200 mg ↓DTI peak SR in placebo group | — | — |
Cheung et al. (2010) | 45 children | Dox, dauno 240 mg max (off 1 y) | 6 y | Volumes, 3D LVEF, E, A, DT, DTI S′, e′, a′, STE strain, SR dyssynchrony | Late: 6 y ↓STE circ SR, ↓↓STE circ strain, ↓↓↓ STE rad strain, ↓STE long strain (16% dyssynchrony) | — | — |
Ho et al. (2010) | 70 | Anthra + TZM (after 6 y) | 6 y | Volumes, E, A, E/A, IVRT, DT, MPI, DTI S′, e′, a′, e′/a′, E/e′, STE long and rad strain | Late: 6 y ↓S’, E, E/A, ↓long strain (26% of pts) | — | — |
Appel et al. (2011) | 80 | Epi 270 mg (low dose) + cyclophosp | Pre; after 3 cycles (9 wk each cycle) | E, A, E/A, DT, MPI, DTI S′, e′, a′ | Mild ↓E/A | — | — |
Fallah-Rad et al. (2011) | 42 | Anthra + TZM | Pre-anthra; pre-TZM; 3, 6, 9, 12 mo during TZM | DTI S′, e′, a′, STE long and rad strain, SR | Early: 3 mo ↓S′, ↓global long, rad strain | 10/42 (24%) at 6–9 mo | ↓S′: sens, 93%; spec, 99%; long strain 79%–82%; rad strain 86%–81% |
Sawaya et al. (2011) | 43 | Dox/epi (240/300 mg/m 2 ) + TZM | Pre-anthra; 3, 6 mo during TZM | E, A, E/A, DTI e′, a′, e′/a′, E/e′, strain peak systolic long, rad, circ | Early: 3 mo ↓long strain (by 11%) 6 mo ↓circ strain (by 15%) | 9/43 (21%) at 6 mo (1 pt at 3 mo) | ↓Long strain in 14 pts; sens, 78%; spec, 79% |
Stoodley et al. (2011) | 52 | Dox/epi (12–18 wk) | 1 wk before; 1wk after | Volumes, STE strain, SR | ↓Global long strain (48%), ↓global rad strain (59%) | — | — |
Poterucha et al. (2012) | 19 | Anthra 296 ± 103 mg/m 2 | Pre, 4, 8 mo | LVEF, long peak systolic strain | ↓Long peak systolic strain at 4, 8 mo | ↓Long peak systolic strain compared with controls at 4, 8 mo | ↓LVEF at 8 mo |
Sawaya et al. (2012) | 81 | Anthra + taxanes +TZM | 3 mo (15 mo) | LVEF, peak long, rad, circ strain | ↓Global long strain | 26/81 (32%) at 15 mo | 60% symptoms of HF |
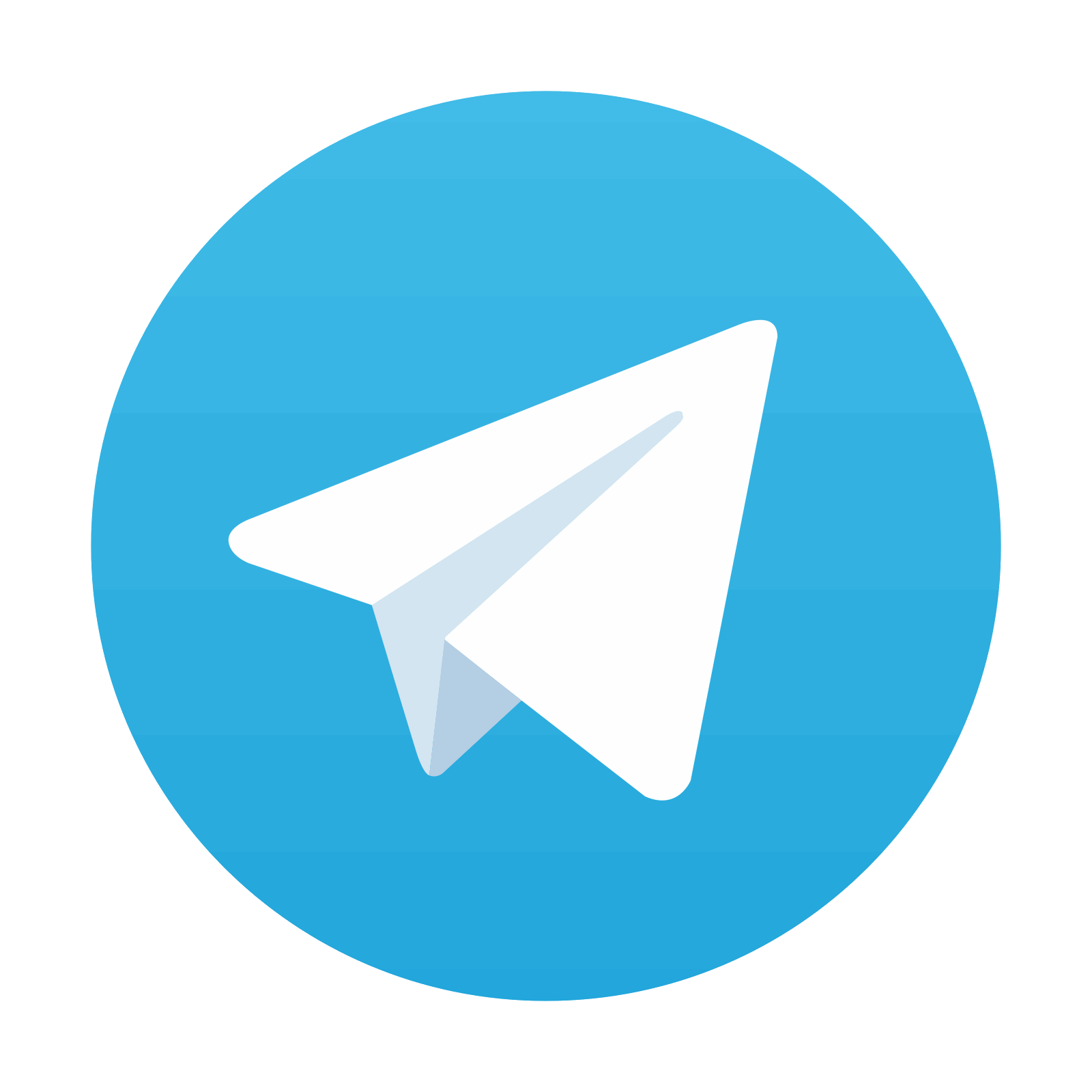
Stay updated, free articles. Join our Telegram channel

Full access? Get Clinical Tree
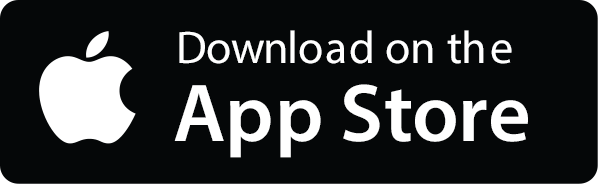
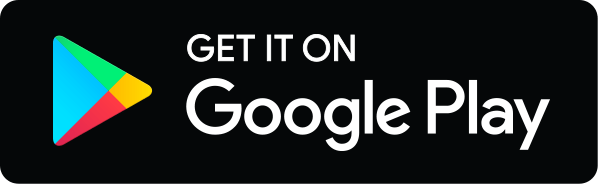
