Summary
Despite considerable advances in pharmacological, surgical and technology-based cardiovascular therapy, left ventricular dysfunction and heart failure are increasingly prevalent health problems. Recent studies suggest that angiogenic gene therapy can restore perfusion in ischaemic myocardial tissue, and that the transfer of nonangiogenic genes may correct defects in calcium handling that contribute to abnormal contractile function in patients with heart failure; however, large clinical trials of gene therapy for treatment of left ventricular dysfunction and heart failure have yet to be completed, and only a small number of genes have been evaluated in patients. Researchers continue to investigate new genes, combinations of genes and approaches that combine gene and cell therapy, and to develop novel expression vectors and delivery systems; collectively, these refinements promise to improve both patient response and safety.
Résumé
Malgré les considérables avancées dans les traitements médicamenteux, interventionnels et chirurgicaux, la dysfunction ventriculaire gauche et l’insuffisance cardiaque restent d’importants problèmes de santé publique. De récentes études suggèrent que la thérapie génique angiogénique peut restorer une perfusion du tissu myocardique ischémique et que le transfert de gènes non angiogéniques peut corriger les anomalies du transport calcique qui contribuent aux défauts de contraction myocardique chez les patients avec insuffisance cardiaque. Cependant, de grandes études cliniques de thérapie génique pour le traitement de la dysfunction ventriculaire gauche et de l’insuffisance cardiaque sont en attente. Seul un petit nombre de gènes a été étudié à ce jour chez l’homme. Les chercheurs poursuivent les travaux avec de nouveaux gènes, des combinaisons de gènes, des approches qui associent thérapies cellulaires et géniques, ainsi qu’avec le développement de nouveaux vecteurs et systèmes de délivrance. Le but est d’améliorer le bénéfice pour les patients en s’assurant de la bonne tolérance de ces procédures.
Background
LVD and heart failure are increasingly important health problems despite considerable advances in pharmacological, surgical and technological approaches to treatment. Heart failure is the leading medical cause of hospitalization and is expected to cost the USA health care system $37.2 billion in direct and indirect expenses in 2009 . The most common cause of LVD is coronary artery disease, followed by hypertension and valvular disease. Heart failure, which often develops in patients who survive myocardial infarction, is a debilitating disease with high morbidity and can lead to frequent hospital re-admissions. The prognosis for patients with chronic heart failure remains poor. Nonpharmacological therapies, such as heart transplantation and the use of implantable left ventricular assist devices, are considered appropriate only in later stages of disease progression and, consequently, are considered for a very small fraction of younger patients.
The impaired cardiac contractile function associated with heart failure can be attributed to declines in perfusion and to unfavourable cardiac remodelling. Because gene therapy is designed to restore perfusion, and newer treatments may also target abnormalities in the contractile function of viable cardiomyocytes, it may be considered a promising alternative for the treatment of LVD and heart failure. Here, we describe several genes that are currently under investigation, the advantages and disadvantages of the vectors and delivery routes that are used most frequently, and the implications of findings from preclinical and early clinical trials of cardiovascular gene therapy.
Gene candidates
Numerous proteins, growth factors, cytokines and calcium-transport regulators have been the focus of angiogenic and myogenic research. Several genes have been investigated in vitro and in animal models, and the promising results of these studies have justified the first clinical evaluations. A number of these genes are described below, but only a few have been used clinically.
Vascular endothelial growth factor
VEGF, which could be considered the prototype for angiogenic gene therapy , has been shown to improve LVD in animal models of both ischaemic and pacing-induced heart failure . VEGF-A (also called VEGF-1) was first discovered in 1989 and is the founding member of the VEGF protein family. VEGF-A is upregulated during pathological vessel growth , but it possesses a diverse array of pro-angiogenic properties and is the isoform used most frequently in gene therapy. Several VEGF-A derivatives, each containing a different number of amino acids (e.g., VEGF-121, VEGF-165, VEGF-189, and VEGF-206), can induce angiogenesis in animal models ; their solubilities and binding characteristics (e.g., to heparin and the extracellular matrix) differ, which influences their ability to interact with target cells and, presumably, alters their angiogenic potency. VEGF-165 is the most promising for use in angiogenic therapy, because it is the most potent (100-fold more potent than VEGF-121) and can induce the developmental gradients required to pattern vessel growth .
Fibroblast growth factor
The FGF family includes 23 members, of which acidic FGF (FGF-1) and bFGF or FGF-2 are the best characterized. The biological activity of FGF is not well understood. FGF proteins are potent mitogens for a variety of cell types, including endothelial cells, vascular smooth muscle cells and fibroblasts, and FGF secretion stimulates the synthesis of proteases that contribute to angiogenesis by digesting the extracellular matrix. Unlike VEGF, FGF-1 and FGF-2 are not crucial for embryogenesis, and because they lack a secretory signal sequence, they enter the extracellular space only passively after cell damage. Nevertheless, both have been used successfully to induce angiogenesis , and the secreted FGF isoforms, such as FGF-4 and FGF-5, may have even more therapeutic potential.
Hypoxia-inducible factor
The metabolic stimuli associated with hypoxia and ischaemia induce expression of a variety of transcription factors that stimulate angiogenesis, and researchers have begun to investigate the genetic transfer of these factors as an alternative to growth-factor-based gene therapy. Because interactions between HIFs and hypoxia response elements trigger an “angiogenesis programme” by upregulating the expression of a number of growth factors and cytokines simultaneously, the therapeutic administration of HIF could enhance vascular growth by mimicking the natural angiogenic response. HIF1-alpha regulates the expression of SDF-1, which is critically involved both in the mobilization of angiogenic progenitor cells from the bone marrow to the peripheral circulation and in the recruitment of mobilized cells to ischaemic tissue . However, HIF1-alpha can also induce cell death and may be significantly less potent than VEGF .
Sonic hedgehog
Recent experiments performed in our laboratory indicate that the embryonic hedgehog signalling pathway can be reactivated to combat ischaemia in adult mammals. Recombinant Shh protein induces a robust angiogenic effect by upregulating multiple angiogenic factors, including VEGF, in interstitial mesenchymal cells , and genetic transfer of Shh enhances the regeneration of ischaemic myocardium by inducing the expression of trophic factors, such as SDF-1, which increases the recruitment and incorporation of bone marrow-derived progenitor cells into the growing vasculature . We have also shown that Gli3, a transcription factor targeted by Shh during hedgehog signalling, is strongly upregulated in the ischaemic tissue of adult mammals and may have a favourable effect on myogenesis and angiogenesis after an ischaemic insult . Because Shh appears to trigger a cascade of pro-angiogenic factors, it may be particularly effective for angiogenic gene therapy.
Stromal cell-derived factor-1
SDF-1 (also called CXC CXCL12) is a 68-amino-acid protein of the CXC chemokine family. Two isoforms, SDF-1-alpha and SDF-1-beta, are encoded as splice variants of a single gene and are expressed by both endothelial cells and stem cells. The growth factor activity of SDF-1 has most often been linked to lymphopoiesis and myelopoiesis , but interactions between SDF-1 and its receptor CXCR4 also regulate progenitor cell trafficking, and SDF-1-alpha is essential for the recruitment of stem and progenitor cells to ischaemic tissue .
Factors that regulate calcium transport
Abnormalities in the function of molecules responsible for the rhythmic release and uptake of Ca 2+ ions in myocytes contribute to impaired cardiac contractility in patients with heart failure , and because myocardial contractility is dependent on ventricular Ca 2+ handling, genetic modification of these molecules could be a viable approach for treatment of heart failure. One of the key Ca 2+ handling abnormalities in both humans and experimental models of heart failure is caused by a defect in sarcoplasmic reticulum function, and a large body of experimental evidence indicates that SERCA2a plays an important role in the progression of dilated cardiomyopathy. SERCA2a activity is known to decline in late-stage heart failure, and SERCA2a protein and messenger RNA levels are reduced in cardiac tissue isolated from the failing hearts of patients and animals with heart failure . Furthermore, gene therapy with a pseudophosphorylated mutant of phospholamban, the principal regulator of SERCA2a, treated cardiomyopathy in hamsters and infarction-induced heart failure in rats successfully for 6 months or more . Preclinical studies also indicate that increases in cardiac adenylyl cyclase content improve left ventricular function, attenuate deleterious remodelling and reduce mortality in both heart failure and acute-infarction models. Investigations of adenylyl cyclase type 6 gene transfer have progressed from studies in cultured cardiac myocytes to animal models of heart failure .
Gene delivery
Vectors
Unlike protein administration, gene therapy can lead to high, sustained protein levels; however, the effectiveness of gene transfer depends on the transfection efficiency – the amount of the gene internalized by cells in the target tissue – and the magnitude and endurance of subsequent expression. The cellular insertion and intracellular trafficking of the transgene is facilitated by vectors, which can be categorized as viral or nonviral. Nonviral plasmid vectors were used in early investigations because they are inexpensive, easily constructed, and generally considered safe; plasmid vectors do not initiate inflammation or an immune response and incur no risk of insertional mutagenesis. Plasmid DNA is taken up effectively and expressed by all mammalian cell types, including cardiomyocytes, in vivo ; however, the transfection efficiency of plasmid vectors was low in randomized, controlled trials . Nevertheless, plasmid vectors could be useful when short-term modification of gene expression may be beneficial, such as immediately after an acute cardiac event, or for initiating mechanisms that lead to progenitor cell recruitment and to the activation of resident stem cells. More recently, small interfering RNAs have become popular for inhibition studies and could provide a new option for nonviral gene manipulation; very high transfection efficiency can be achieved by administering decoy receptors or antibodies that circumvent blocking factors in cardiovascular tissue.
The viral vectors used most frequently for cardiovascular gene therapy are adenoviruses and AAVs; retroviral vectors were used in early studies, but their popularity has declined because of safety concerns. Compared with plasmid transfection, viral transfection into vessel walls and heart muscle is much more efficient , and virally transfected genes are typically expressed for a longer period of time – AAV-transfected genes can be expressed for months – although the duration varies depending on the virus used. In addition, viral vectors typically infect only a limited number of cell types, and this specificity (i.e., tropism) can be advantageous for cardiovascular therapy. Adenoviruses seem particularly effective for transfecting cardiomyocytes , and naturally occurring tropisms for vascular smooth muscle cells and cardiomyocytes are among the more useful characteristics of AAVs. However, viral vectors can generate an inflammatory or immunogenic response and may integrate into the cellular genome, which could increase cancer risk. Nevertheless, adenoviruses have a good safety record in cardiovascular clinical trials , and the likelihood of inflammation, immunogenicity and host-genome integration varies depending on the vector used.
Routes of administration
Clinical acceptance of gene therapy as a routine treatment option will require the development of standardized, practical delivery systems and techniques for delivering the gene to the tissues targeted most frequently, such as the myocardium and blood vessels. Genes can be injected directly into the targeted tissue for treatment of peripheral disease, but local delivery to the heart used to require open-chest surgery or thoracoscopy. Intracoronary administration or catheter-based delivery systems (e.g., navigation and catheter mapping technology) for transendocardial gene delivery to the myocardium are much less invasive and more feasible for patients with LVD and heart failure .
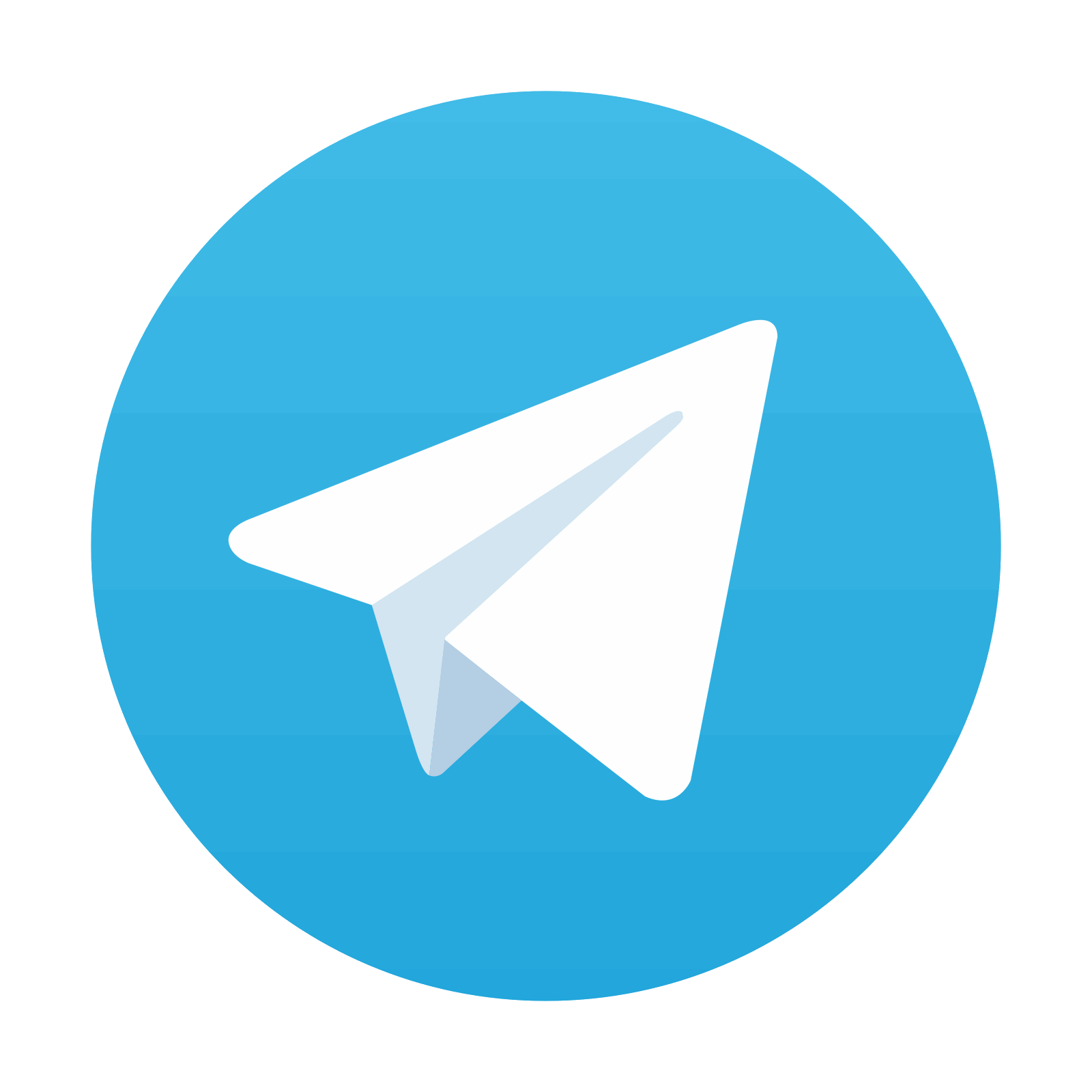
Stay updated, free articles. Join our Telegram channel

Full access? Get Clinical Tree
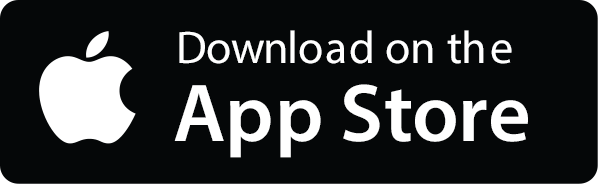
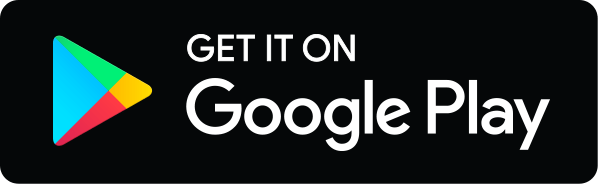