Understanding Technology and Equipment
S. Suave Lobodzinski
KEY POINTS
Electrocardiogram (ECG) leads are the electrocardiographer’s electric windows into the heart’s electric activity, not wire-electrode assemblies.
Torso modifications of limb leads are nonstandard and can affect the ECG signal. Such changes may obscure or create changes of myocardial infarction and right axis deviation.
Patch monitors appear to be best suited for detecting and cataloging atrial fibrillation (AF) episodes, although they may produce false positives if P-waves are not detected because of small signal amplitudes.
Modern Holter devices now have the ability to record and document continuous 12-lead ECG signal simultaneously with a multitude of other biologic signals during normal daily activities.
Ambulatory ECG (AECG) recorded in free-living subjects has issues with signal quality because of skin stretch artifacts, wire entanglements, and occasional skin dermatitis caused by electrode gels.
Mobile cardiac telemetry devices provide near-real-time information on detected arrhythmic events; however, a single-lead ECG limits their diagnostic capabilities.
Patch ECG monitors should not be used in lieu of multilead Holter monitoring.
Patch ECG monitors are not recommended for use in children or in adults with cardiac pacemakers or other implanted electronic devices.
Insertable loop recorders are the only presently available devices that allow continuous ECG monitoring for up to 3 years and that can document arrhythmia burden over time (including AF) without the need for active intervention by the patient.
Rapidly advancing AECG technology blurs the established monitoring categories such as Holter and loop recorders, event monitors, and mobile cardiac telemetry.
Multifunction hybrid cardiac monitors with AECG functionality are expected to gradually replace older single-function devices.
INTRODUCTION
The purposes of this chapter are (1) to examine the relation of the ambulatory electrocardiogram (AECG) to its technology, (2) to further the understanding of how the modern AECG is derived and recorded, and (3) to provide a systematic review of modern AECG devices in the context of the ECG monitoring practice. Special emphasis will be placed on the rapidly evolving new technologies such as systems on chip electronics, cloud-connected devices, and emerging multiparameter hybrid monitors. The evolution and application of AECG technologies have profound clinical implications, as exemplified by the very long-term monitoring periods approaching implantable devices. We recognize that the technical nuances of modern AECG may be unfamiliar to many clinicians, hence the goal of this chapter is to provide more insight into the generally missing link between the selection of monitoring technology and its consequences for clinical AECG interpretation.
Measurement of Bioelectromagnetic Field of the Human Heart on the Body Surface
To better understand the ECG measurement problem, let us reexamine the heart-originated bioelectric potentials on the body surface. The discovery of the existence of the bioelectric three-dimensional field generated by the heart dates back to Augustus Waller1 who recorded the distribution of the electric potentials on the surface of the thorax as shown in Figure 10.1.
Using Lippmann’s capillary electrometer to record the body surface potentials, he explored five locations to produce 10 leads. Out of these, he selected five cardinal leads. Einthoven’s contribution to ECG2 was the creation of the first ECG apparatus
that used string galvanometer to record the first practical ECG. He further simplified Waller’s lead system to record ECG from extremities, because he was able to minimize the skin-electrode impedance by submerging arms and feet in the buckets of salty water. This so-called Einthoven lead system (Einthoven triangle) is still in use today. In 1942, Goldberger developed the concept of a “zero potential electrode” (known today as central Wilson terminal) and added the augmented leads to standard ECG. Subsequent advancements in ECG instrumentation technology finally allowed for practical recording of the biopotentials from the thorax surface. In 1944, Wilson introduced the precordial leads (chest leads), and the standard 12-lead system representing the heart’s electrical activity recorded from electrodes on the body surface as shown in Figure 10.2 was established.3
that used string galvanometer to record the first practical ECG. He further simplified Waller’s lead system to record ECG from extremities, because he was able to minimize the skin-electrode impedance by submerging arms and feet in the buckets of salty water. This so-called Einthoven lead system (Einthoven triangle) is still in use today. In 1942, Goldberger developed the concept of a “zero potential electrode” (known today as central Wilson terminal) and added the augmented leads to standard ECG. Subsequent advancements in ECG instrumentation technology finally allowed for practical recording of the biopotentials from the thorax surface. In 1944, Wilson introduced the precordial leads (chest leads), and the standard 12-lead system representing the heart’s electrical activity recorded from electrodes on the body surface as shown in Figure 10.2 was established.3
Standard 12-lead ECG (stdECG) is shown in Figure 10.2. It is important to note that the stdECG system records the biopotentials in three dimensions as shown in Figures 10.3 and 10.4.
We observe that stdECG bioelectric field is three dimensional and the electrode placements reflect that. It is important to note that the ECG leads refer to the electric views of the heart and not physical wire-electrode “leads.”
All leads used in ECG measurements are bipolar. The term “unipolar lead” is a misnomer and should be avoided. One should be careful to clarify in which context (medical or engineering) the term “lead” is used.
RECORDING THE AECG
The patient’s skin, along with the monitor, cable, lead wire, connector, and ECG electrode all play distinct roles in recording an ECG. The recording of AECG signal starts with the electrodes, which translate ionic charges within the body into skin potentials. An instrumentation amplifier at the input of AECG measures the difference between the right arm (RA) and left arm (LA) potentials as shown in Figure 10.5.
There is, however, an additive omnipresent noise on the body surface because of radiofrequency interference, which obstructs the true ECG signals and must be eliminated. These noise signals that are present at different body locations are referred to as “common mode,” meaning that they are common to all ECG measurement sites.
Often, these signals are much higher in amplitude than the ECG itself; therefore, the task of the instrumentation amplifier is to reject them by subtracting the voltage potentials from each other. This feature of the amplifier is known as “common mode rejection ratio” and is measured in decibels (dB). Because the amplifiers are not perfect, designers developed an active circuit that measures the common noise, reverse its phase, and inject it into the body surface, thus further canceling the common mode interference as shown in Figure 10.5.
Often, these signals are much higher in amplitude than the ECG itself; therefore, the task of the instrumentation amplifier is to reject them by subtracting the voltage potentials from each other. This feature of the amplifier is known as “common mode rejection ratio” and is measured in decibels (dB). Because the amplifiers are not perfect, designers developed an active circuit that measures the common noise, reverse its phase, and inject it into the body surface, thus further canceling the common mode interference as shown in Figure 10.5.
Electrodes and the Skin-Electrode Interface
Virtually all disposable and embedded electrodes used by AECG monitoring devices in use today utilize wet-gel electrodes. These are nonpolarizable electrode types, with a silver-silver chloride (AgCl) element (snap) coated with an ionically active gel,4 with exception of some polarizable silver textrodes (textile electrodes) embedded in the shirt/vest carrier.5 All ECG monitoring electrodes must comply with ANSI/AAMI EC12:2000 (R 2010) standard and are subject to regulatory oversight.6 When the electrodes are placed on the body surface, the skin becomes an integral part of the interface. Should this interface be compromised because of high patient skin impedance,7 the signal integrity will be adversely affected, causing the baseline to wander, substantial noise (artifact), and even loss of the ECG signal. Other factors beyond high patient skin impedance such as motion artifacts (electrode pull, skin stretch), a strong electrical field (60 Hz), humidity, temperature, static electricity, or the patient’s
skin condition such as diaphoresis may also influence the ECG signal quality. Often most clinicians do not realize that the skin is a major source of ECG artifact. Artifacts can lead to unnecessary electrode changes, higher costs, false alarms, and frustration for staff and the patients. It is therefore important to understand how the body biopotentials are transferred into electrical signals presenting as inputs to the AECG monitor. A simplified skin-electrode interface model is shown in Figure 10.6. The model7 is a combination of a resistor in series with a paralleled resistor and capacitor. Ehc is the voltage between the skin and electrolytes, such as a gel. This model is applicable to wet electrodes used in AECG applications. The capacitance Cd represents the electrical charge between the electrode and skin, whereas Rd stands for the resistance that occurs between the skin and electrode during charge transfer.7 Rs represents the resistance of the electrolyte gel (if any), sweat, and the underlying skin tissue7 (Figure 10.6).
skin condition such as diaphoresis may also influence the ECG signal quality. Often most clinicians do not realize that the skin is a major source of ECG artifact. Artifacts can lead to unnecessary electrode changes, higher costs, false alarms, and frustration for staff and the patients. It is therefore important to understand how the body biopotentials are transferred into electrical signals presenting as inputs to the AECG monitor. A simplified skin-electrode interface model is shown in Figure 10.6. The model7 is a combination of a resistor in series with a paralleled resistor and capacitor. Ehc is the voltage between the skin and electrolytes, such as a gel. This model is applicable to wet electrodes used in AECG applications. The capacitance Cd represents the electrical charge between the electrode and skin, whereas Rd stands for the resistance that occurs between the skin and electrode during charge transfer.7 Rs represents the resistance of the electrolyte gel (if any), sweat, and the underlying skin tissue7 (Figure 10.6).
![]() FIGURE 10.5 The formation of a directly measured electrocardiogram lead together with the adaptive noise reduction circuit. LA, left arm; RA, right arm; RL, right leg. |
Some electrode gels may cause skin adverse reactions such as dermatitis, itching, and redness, prompting patients to scratch or outright remove them. Hence, a selection of good quality monitoring electrodes is critical to patient comfort.
Lead Systems Used in AECG Applications
Because it is not practical to use stdECG as shown in Figure 10.2 in AECG applications because of limb and wrist electrode placements, a number of substandard, modified lead configurations have been developed to reduce wire clutter, cost, measurement artifacts and increase patient comfort.8,9 The most widely used “stdECG-like” lead systems in ambulatory monitoring are Mason-Likar (ML)9 and EASI10,11 lead systems. Other reconstructed 12-lead systems include TruSt and 12RL. The ML configuration features 10 electrodes with extremity locations moved to the torso, as shown in Figure 10.7.
The arm electrodes are placed in the infraclavicular fossae medial to the deltoid insertions and the left leg (LL) electrode is placed midway between the costal margin and iliac crest in the left anterior axillary line. More recent applications of the ML monitoring position place the arm electrodes over the outer clavicles and the right leg (RL) electrode (body potential reference) at the sternum. The precordial electrodes are placed in the standard positions.12,13,14,15
EASI—Phillips Medical Systems (Andover, Massachusetts)
The EASI lead system is a reduced 5-electrode lead system that uses the E, A, and I locations derived from the Frank lead system15 and adds “S” electrode, at the top of the midsternum, along with the body reference electrode to provide orthogonally oriented signals. This eliminates the need to determine intercostal spaces and avoids the breasts. EASI lead system makes use of the transformation coefficient matrix that produces synthesized quasi-stdECG. EASI is well suited for AECG applications because of the reduction of a number of electrodes, reducing signal artifacts in free-living subjects.16
12RL ECG—GE Healthcare (Milwaukee, Wisconsin)
12RL is marketed as a “12-lead view” in ST monitoring applications, useful for QT/QTc interval measurement using a reduced lead set. It uses six electrodes in standard placement (RA, RL, LA, LL, V1, and V5) as shown in Figure 10.7. Little is known about 12RL outside these two references.17,18
TruST—Dräger (Huston, Texas)
The modified lead system marketed by Dräger, Hospital System division (Telford, Pennsylvania) is very similar to 12RL, but instead of V1, lead V2 is used. It is marketed as the “12-lead equivalent” for ST-segment monitoring applications.
Other most popular reduced lead configurations19,20,21,22 used primarily in Holter studies include 2-channel (V6 and V1 like) or 3-lead, 5 electrodes and 3-channel 7-electrode systems as shown in Figure 10.8.
a. 2-Channel (V6 and V1 like), 5 electrodes. The neutral RL lead may be positioned in any location least subject to motion artifact (shown in midsternum position). The V lead can be positioned in any of the precordial (V1-V6) positions according to clinician preference (shown in V1 location). The LL lead positioned on the lower left rib cage may ensure the least amount of artifact; however, to be comparable with a stdECG Lead II, the LL lead should be placed on the lower left side of the body, as close to the hip as possible. The electrode cups of these configurations are color-coded as per AHA and IEC standards and are shown in Table 10.1.
b. 3-Channel 7-electrode Channel 1: (1+) 5th rib, (1-) left anterior axillary line-right manubrium, Channel 2: (2+) 2 cm, the right of the xiphoid process, (2-) left manubrium and Channel 3: (3+) 5th rib, left of midclavicular line, (3-) centered on manubrium. Reference RL: any location least subject to motion artifact, shown in central sternum position.
The new electrode locations have emerged to accommodate single-lead patch monitors as shown in Figure 10.9. Biopotential difference measured by a single channel at these locations (ZIO and CAM) are nonstandard and are best characterized as body surface electrograms, rather than ECG. Furthermore, ZIO locations are far away from the atria and P-waves may not be well visible in low-voltage ECGs, thus contributing to many inaccuracies in detecting supraventricular events. The signal from CAM locations will show higher voltage amplitudes because of its atria proximity and is believed to be better suited for atrial arrhythmia monitoring. However, it
may not have enough signal information to detect ST-changes in myocardial infarction (MI) applications.
may not have enough signal information to detect ST-changes in myocardial infarction (MI) applications.
TABLE 10.1 Summary of the 5-Electrode Lead System | |||||||||||||||||||||||||||||||||||
---|---|---|---|---|---|---|---|---|---|---|---|---|---|---|---|---|---|---|---|---|---|---|---|---|---|---|---|---|---|---|---|---|---|---|---|
|
There are many single-lead event monitors in use today that use two or three electrodes to capture 1-channel ECG data. The placement recommendations vary widely and therefore will not be discussed here.
In summary, ML or proprietary reduced lead system (RLS) configurations are used for continuous 12-lead monitoring. Because each RLS set has a different electrode configuration and derivation than stdECG, they are inherently different and should not be compared with each other or with the ML or stdECG to determine changes in an individual over time.23
ML modifications, for example, will produce important amplitude and waveform changes associated with a more vertical and rightward shift of the QRS frontal axis, particularly in those with abnormal standard ECGs. Such changes generate important ECG abnormalities in patients with normal standard ECGs, suggesting “heart disease of ECG origin.” In those with abnormal standard ECGs, moving the limb leads to the torso may result in possible myocardial infarcts to appear and five inferior infarcts to disappear. In addition, ML may introduce clinically important T-wave or QRS frontal axis changes.24
In case of patch monitors, the signal amplitude acquired from the patch monitor locations is always lower,25 hence it may be masquerading as low-voltage ECG indicative of chronic obstructive pulmonary disease, pericardial effusion, severe hypothyroidism, subcutaneous emphysema, massive myocardial damage/infarction, or infiltrative/restrictive diseases such as amyloid cardiomyopathy.26
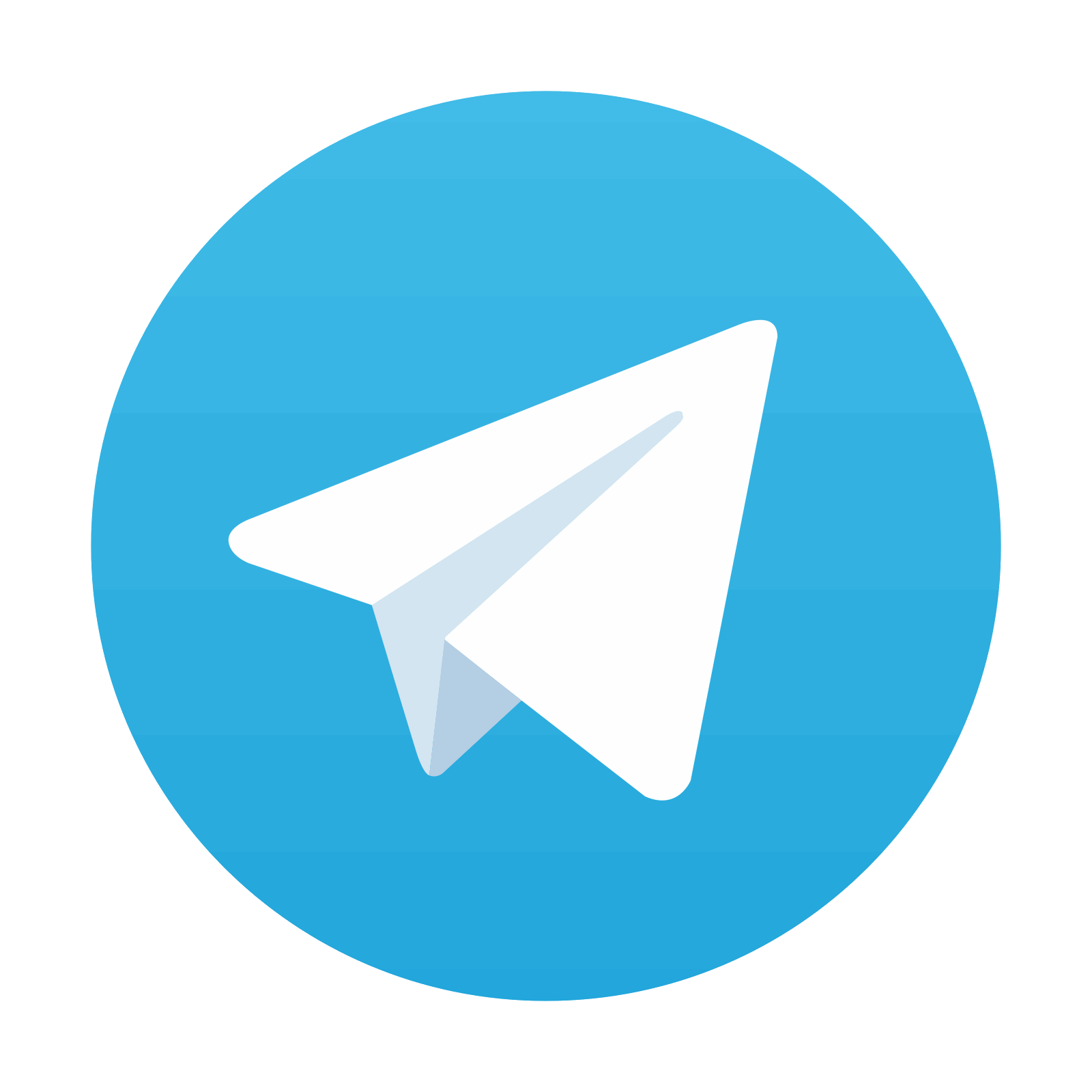
Stay updated, free articles. Join our Telegram channel

Full access? Get Clinical Tree
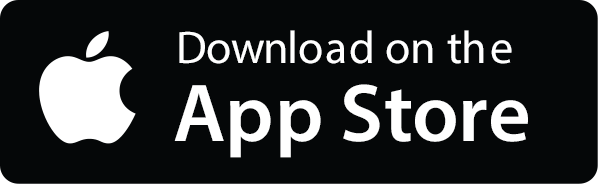
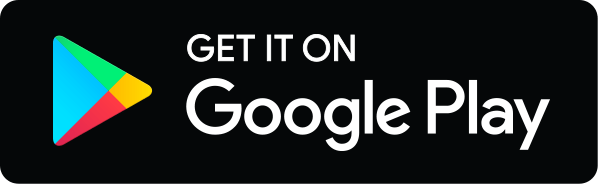
