Abstract
Imaging artifacts encompass patterns in the image that seem to suggest the presence of structures that are in reality not present. They may in fact relate to both the appearance of nonexistent structures, as well as the concealing of existing structures. Artifacts are mostly caused by physical interactions between the imaged tissue and ultrasound itself that are more complex than assumed by the ultrasound system; however, they can also result from malfunctioning ultrasound equipment or their inadequate settings, as well as from interference caused by other electronic equipment. Imaging artifacts can occasionally encumber an echocardiographic examination (particularly for novices), and the knowledge of these occurrences should thus be used to minimize their effect.
Keywords
attenuation artifacts, refraction artifacts, reverberation artifacts, side-lobe artifacts, ultrasound artifacts
Introduction
Imaging artifacts encompass patterns in the image that seem to suggest the presence of structures that are in reality not present. They may in fact relate to both the appearance of nonexistent structures, as well as the concealing of existing structures. Artifacts are mostly caused by physical interactions between the imaged tissue and ultrasound itself that are more complex than assumed by the ultrasound system; however, they can also result from malfunctioning ultrasound equipment or their inadequate settings, as well as from interference caused by other electronic equipment. Imaging artifacts can occasionally encumber an echocardiographic examination (particularly for novices), and the knowledge of these occurrences should thus be used to minimize their effect. In this chapter, the most common artifacts have been categorized according to the authors’ discretion, although they recognize that alternative ways of classifying these artifacts are possible, as no standard nomenclature exists and reference to certain artifacts in the literature is limited ( Table 7.1 ).
B-Mode Artifacts
Shadowing (Attenuation) and Dropout Artifacts
Shadowing (i.e., attenuation) artifacts obscure certain (segments of) underlying structures. When imaging structures with mechanical properties (i.e., mass density and/or compressibility) substantially differ from soft tissue (e.g., metals such as used in prosthetic valves or air/contrast bubbles), very strong reflections will occur, resulting in little or no transfer of ultrasound energy to more distal regions, as (almost) all energy will have been reflected. This will manifest as a strong reflection in the area of the reflector, followed by an “acoustic shadow” that represents a signal void ( Fig. 7.1 , yellow dashed arrows, ).


This shadowing will affect not only the two-dimensional (2D) image but also the color Doppler signal, which could be, for example, highly relevant in the assessment of regurgitant jets in the setting of prosthetic heart valves. Heavily calcified tissue is a similarly strong reflector; resorting to alternative scanning windows can mostly circumvent such artifacts. For more information on negative shadows, refer to Box 7.1 and Fig. 7.2 .
Although shadowing is typically seen as a darker zone (i.e., signal void) distal to a highly reflecting (most common) or absorbing (less common) structure, the opposite can also occur in that a structure is attenuating less than what is assumed by the scanner’s automatic time gain compensation (refer to Chapter 1 ). As a result, the distal echo signals are overamplified by the scanner, resulting in a brighter zone distal to the low-attenuating structure, which is referred to as a “negative” shadow. A typical example is that of a cyst, as the liquid in the cyst is attenuating the ultrasound wave less than soft tissue, while the scanner automatically corrects for attenuation, assuming it is imaging soft tissue only. An example of such a negative shadow following a cyst in the liver is given in Fig. 7.2 . In cardiac imaging, negative shadows do not normally occur.

Similarly, superficial structures leading to notable attenuation of ultrasound may significantly impair its penetration. As a result, the ribs or lung tissue can diminish the ability to image underlying structures, giving rise to “dropout” artifacts that typically occur at some phases of the respiratory cycle. Such artifacts can be reduced or avoided by scanning at different intervals of the respiratory cycle (i.e., breath hold), and occasionally only by choosing another transducer position ( Fig. 7.3 , ). A dropout artifact is thus similar to a shadowing artifact that occurs very near the transducer, thereby causing part of the image to become invisible.




Reverberation Artifacts
During image generation, the signal of the reflected wave arriving at the transducer is transformed to electrical energy (i.e., the radiofrequency signal) to be used in the image reconstruction process (for more details, see Chapter 1 ). However, a certain portion of the reflected wave is not converted to electrical energy and is merely reflected on the transducer surface to start repropagating through the tissue as if it were another ultrasound transmission. This secondary “transmission” is yet again reflected by the tissue and ultimately detected by the transducer ( Fig. 7.4 , upper panel ). Since the ultrasound system assumes all echo signals are resulting from the original transmission, these secondary reflections that arrive late (as they have to travel back and forth twice) will thus be depicted at a greater depth, mimicking a reflecting structure at a greater distance (see Fig. 7.4 , lower panel ).

In fact, the secondary reflections will also be partially reflected at the transducer surface, resulting in an apparent third transmit; this process can be repeated multiple times. Although these second- and/or higher-order reflections always occur, they are normally negligible in amplitude with respect to the primary echo amplitudes and can therefore simply be ignored. However, when strong reflections of the primary wave occur, the reverberated signal (i.e., the signal caused by two or more round-trips) can be substantial and give rise to image artifacts (i.e., reverberation artifacts) . This type of artifact can typically be recognized as the repetition of a strongly reflecting structure at integer multiple distances from the transducer. When the reflecting structure causing the reverberation is moving, so will the induced reverberation (with an amplified motion amplitude; ). If, on the other hand, this structure is not moving (e.g., a rib), the induced artifact will be stationary as well (i.e., a stationary reverberation artifact ;
).
Similarly, reverberations can occur within structures that are being visualized. Indeed, on their way back to the transducer, reflections from the primary wave can meet strong reflectors that cause these reflections to start propagating in the direction away from the transducer again ( Fig. 7.5 ). As such, these waves are propagating with some time lag with respect to the primary wave and create secondary reflections. Obviously, similar as for the transducer-related reverberations described previously, higher-order reflections can arise by multiple round-trips between both boundaries of the object. Structures with well-aligned, flat, strong reflecting boundaries (due to a strong mismatch in mechanical properties), such as catheters or the metallic leaflets of a prosthetic valve, are most likely to give rise to this acoustic “ping-pong.” When the reverberating structure is substantially larger than the length of the ultrasonic pulse, individual “ping-pong” reflections can be detected ( Fig. 7.6 , blue arrows; Fig. 7.7 , yellow arrows; and corresponding ), in which case the name “ step ladder ” artifact is used. On the other hand, if this structure is small compared with the pulse length, all “ping-pong” reflections start to interfere, giving a more hazy appearance in the image referred to as a “ comet tail ” artifact ( Fig. 7.8 ). Depending on the attenuation the reverberating wave experiences, the “step ladder” or “comet tail” fades out faster (i.e., higher attenuation; see Fig. 7.6 ) or slower (i.e., lower attenuation; see Fig. 7.1 , blue arrow; see
and Fig. 7.8 ).

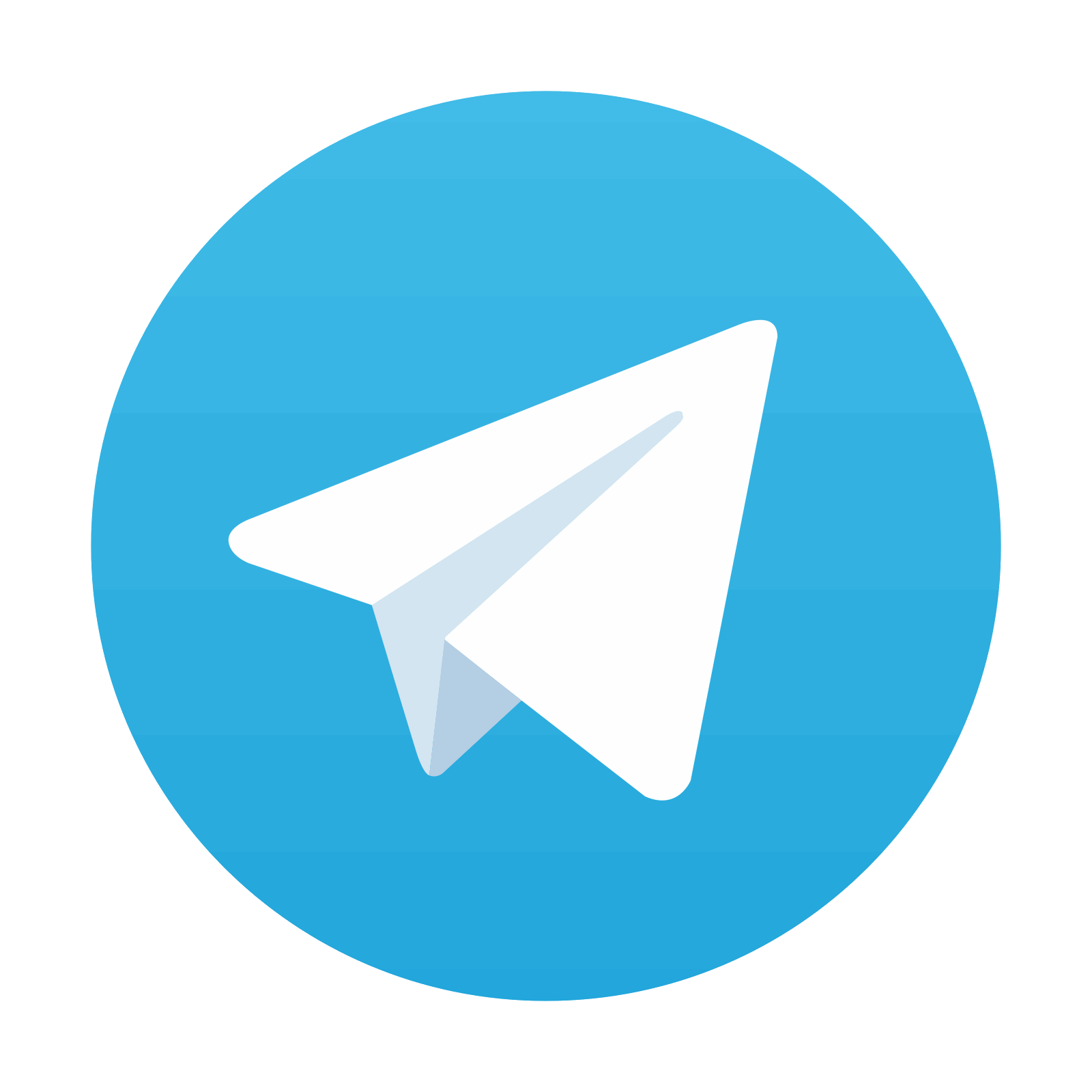
Stay updated, free articles. Join our Telegram channel

Full access? Get Clinical Tree
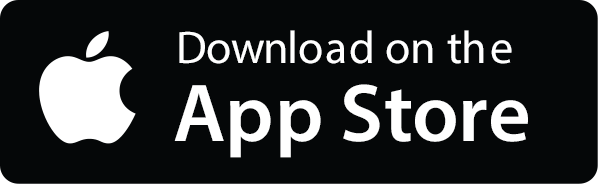
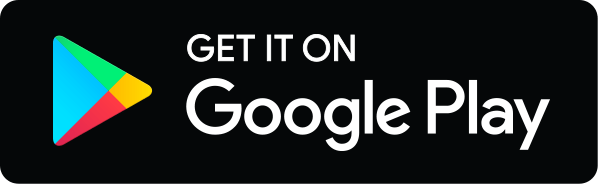
