Ultrasound Propagation Through Tissue
Molly Flannagan
1. Which of the following is the most significant cause of ultrasound attenuation in diagnostic ultrasonography?
A. Thermal absorption
B. Specular reflection
C. Scattering
D. Diffuse reflection
View Answer
1. Correct Answer: A. Thermal absorption
Rationale: Ultrasound attenuation is the decrease in amplitude and intensity of ultrasound waves as they travel through tissue, which occurs as a result of the interaction of the sound waves with the tissue. It is predominantly affected by three factors: thermal absorption, reflection, and scattering. Of these three, thermal absorption is the most significant cause of attenuation.
Thermal absorption is the conversion of ultrasound wave energy into heat as a beam travels through and interacts with tissue boundaries. It occurs in a predictable fashion, and the amount of heat generation can be calculated by using characteristics including wave frequency, ultrasound pressure, tissue density, and the speed of sound. This is the principle behind thermal cellular destruction in ultrasound ablation of lesions such as malignancies.
Specular and diffuse reflection cause attenuation of an ultrasound beam because only a portion of the transmitted wave bounces off the tissue interface and returns to the ultrasound transducer. The point of reflection can be smooth, as in specular reflection, and cause the sound wave to be reflected in a singular direction. In contrast, diffuse reflection results when a structure has an uneven surface and ultrasound waves are reflected back at various angles, resulting in a more unpredictable surface pattern. Regardless of the pattern, both specular and diffuse reflection partially contribute to ultrasound wave attenuation. (See Figure 3.1.)
The cumulative effect of these phenomena is diminished, returning echoes and poorer image intensity of deeper structures. One way to compensate for this effect is by altering the transmission frequency. High-frequency ultrasound waves generate images with higher axial resolution, but because of the increased number of points of interaction with the tissue, these waves are subject to more attenuation. Low-frequency waves, while providing lower resolution, can visualize deeper structures because they undergo less attenuation. Time gain compensation (TGC) is another method to compensate for attenuation, by progressively increasing the signal gain for later returning echoes, making deeper structures appear more bright on the resulting display. (See Figure 3.2.)
Selected Reference
1. O’Brien, W. Ultrasound—Biophysics mechanisms. Prog Biophys Mol Biol. 2007;93(1-3):212-255.
2. What nonthermal effect of ultrasound is most responsible for the ability of shock wave lithotripsy to treat nephrolithiasis?
A. Ionizing radiation
B. Acoustic radiation force
C. Acoustic streaming
D. Cavitation
View Answer
2. Correct Answer: D. Cavitation
Rationale: As ultrasound waves propagate, they produce both thermal and nonthermal effects on the surrounding tissues. The safety profile of ultrasound, with its lack of ionizing radiation, has allowed for its use both diagnostically and therapeutically. Thermal effects are exploited in high-intensity focused ultrasound (HIFU) by focusing ultrasound waves to selected areas causing a predictable degree of tissue heating, resulting in coagulation necrosis and cell death. Clinical applicability of this technology has been used in catheter-based ablation of both benign and malignant lesions.
The nonthermal effects of ultrasound energy include acoustic radiation force, acoustic streaming, and cavitation. Acoustic radiation force and acoustic streaming refer to the transfer of momentum from an ultrasound wave onto an external medium such as tissue- or fluid-filled structures, causing observable displacement of the tissues adjacent to the ultrasound wave. The degree of displacement is dependent upon the intrinsic stiffness of the surrounding tissue, thus the viscoelastic properties of a given tissue can be calculated noninvasively with ultrasonography.
Cavitation is the process by which fluctuations in pressure cause the formation of gas bubbles often from existing gas nuclei within the tissue. Continuous exposure to ultrasound waves causes the microbubbles to grow to a maximal size, then collapse on itself to create a shock wave. This mechanism is the principle behind which ultrasound lithotripsy of kidney stones is achieved.
Selected References
1. American Institute of Ultrasound in Medicine, Official Statement, ALARA principle; 2014.
2. Izadifar Z, Babyn P, Chapman D. Mechanical and biological effects of ultrasound: a review of present knowledge. Ultrasound Med Biol. 2017 Jun;43(6):1085-1104.
3. Which ultrasound wave array is correctly matched with the field of view or beam structure it produces?
A. Linear sequential array—ultrasound waves exiting transducer at 90°, but splay out as they move away from the transducer resulting in a fan-shaped field of view
B. Curvilinear sequential array—radially increasing rings which create a long focal ultrasound beam
C. Phased array—pulsed beams emanating from a single point of origin
D. Annular array—parallel arranged breams created in sequence resulting in a rectangular field of view
View Answer
3. Correct Answer: C. Phased array—pulsed beams emanating from a single point of origin
Rationale: An ultrasound wave array refers to both the activation and organization of the piezoelectric crystals within the actual ultrasound transducer. Depending on the position and function of each of the crystals, the resulting ultrasound image on the screen will vary. Ultrasound arrays are broadly grouped into three functional categories—sequential, phased, and annular.
In sequential arrays, multiple crystals are aligned side by side and fire in sequence. A single scan line will run from one crystal, reflect off the tissue, and return back to the probe. The resulting images are built from numerous ultrasound scan lines next to each other, running in straight lines the length of the tissue and then returning to the receiver. Sequential arrays can be linear or curvilinear, depending on the organization of the piezoelectric crystals within the transducer footplate. In linear sequential arrays (Figure 3.3), also known as switched arrays, crystals are parallel to one another and produce waves 90° from the transducer head traveling in a straight line through the tissue. Consequently, the beams stay parallel to one another throughout the entire tissue and the image corresponds to the width of the ultrasound transducer with uniform beam density throughout. This creates a rectangular field of view.
Curvilinear sequential arrays (Figure 3.4) have the crystals aligned along a curved transducer head. The beams are again emitted at a 90° angle from the transducer head, traveling in straight lines through the tissue. But because of the initial positioning of the crystals in the head, the beams diverge from one another as they move away from the transducer, resulting in decreased beam density farther from the probe head. While curvilinear probes allow for a wider field of view, they sacrifice resolution at increasing depths.
Phased arrays (Figure 3.5) have crystals tightly aligned together, but instead of emitting sequential perpendicular waves, the crystals are activated in pulses at various angles. There are fewer crystals in the transducer, but since they are activated in an oscillating fashion, each crystal can create multiple nonparallel ultrasound scan lines per image. Sound waves are generated from a single point on the transducer and then fan out as they move through the tissue. With each pulse from a single piezoelectric crystal, the resulting ultrasound wave is steered in a slightly different degree. This generates a sector- or wedge-shaped image with a narrow field of view closer to the probe and a wider field of view farther away. The receiver is then able to spatially sort the returning waves based upon time to return and wave amplitude, allowing for image construction with the interrogation of areas from multiple angles. Phased array probes are useful for evaluation of areas with small footplate contact, but a large underlying area of interest, such as cardiac ultrasonography between intervening rib spaces.
Annular arrays (Figure 3.6) are constructed from piezoelectric crystals cut into rings with larger diameter crystals on the outer edge and smaller diameter crystals internally. Each ring has a different acoustic property and will produce different frequency waves, resulting in various depths of natural focus. This creates a long focal zone within the ultrasound beam. This type of array is used predominantly in ophthalmologic evaluations.
Selected References
1. Markowitz J. Probe selection, machine controls, and equipment. In: Carmody KA, Moore CL, Feller-Kopman D, eds. Handbook of Critical Care and Emergency Ultrasound. McGraw-Hill Education; 2011:25-39.
2. Whittingham R, Martin K. Transducers and beam-forming. In: Hoskins P, Martin K, Thrush A, eds. Diagnostic Ultrasound: Physics and Equipment. Cambridge University Press; 2010:23-46.
4. The acoustic impedance of various tissues affects the reflection and transmission of an ultrasound beam as it travels through tissues. Which tissue has the lowest acoustic impedance?
A. Air
B. Fat
C. Skin
D. Bone
View Answer
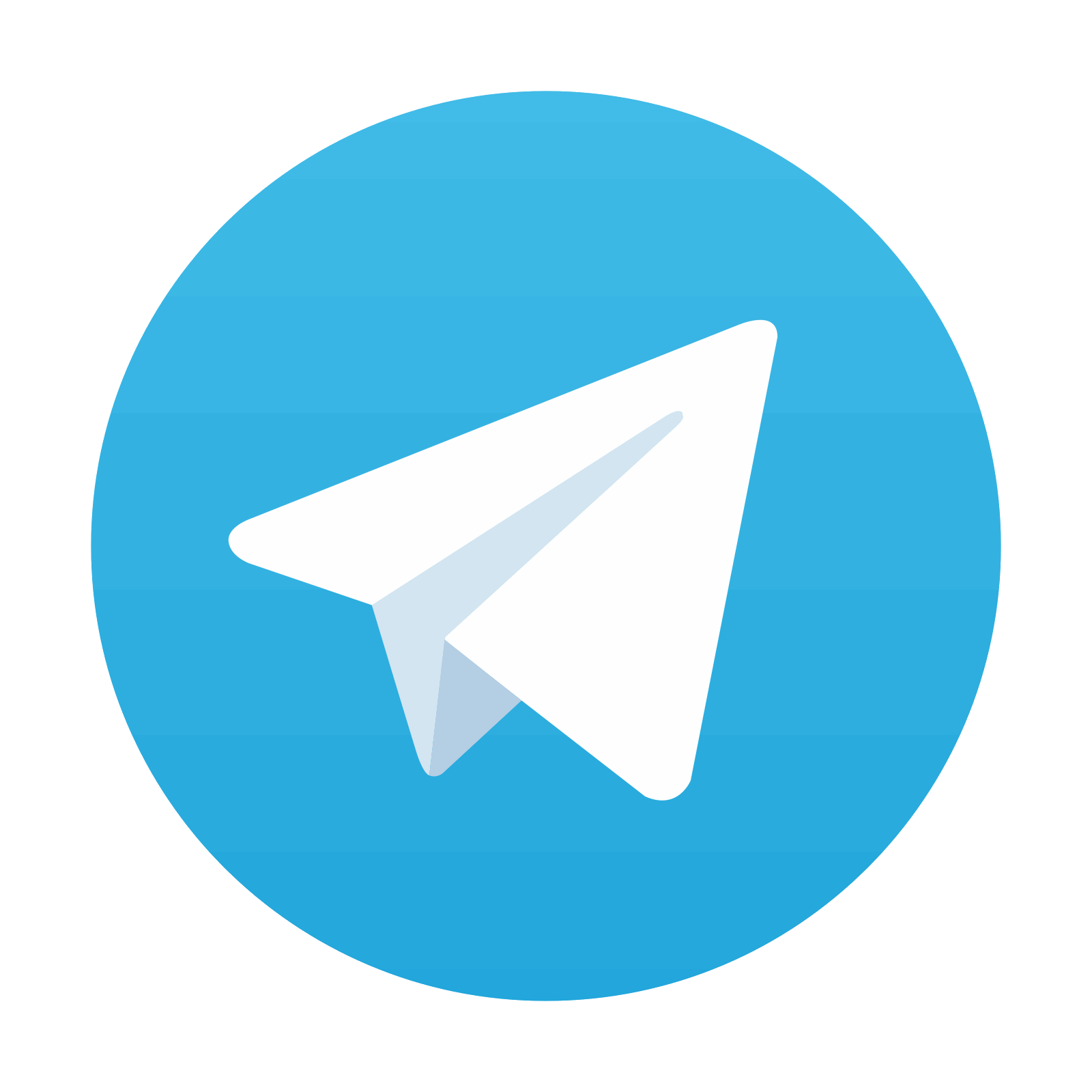
4. Correct Answer: A. Air
Rationale: Acoustic impedance is a physical property of a tissue that describes the amount of resistance an ultrasound beam encounters as it moves through the tissue. Impedance is proportional to the density of the material:
Z = ρ×c
where impedance is Z (kg/(m2s)), r is the density of the tissue (kg/m3), and c is the speed of the sound wave in the tissue (m/s).
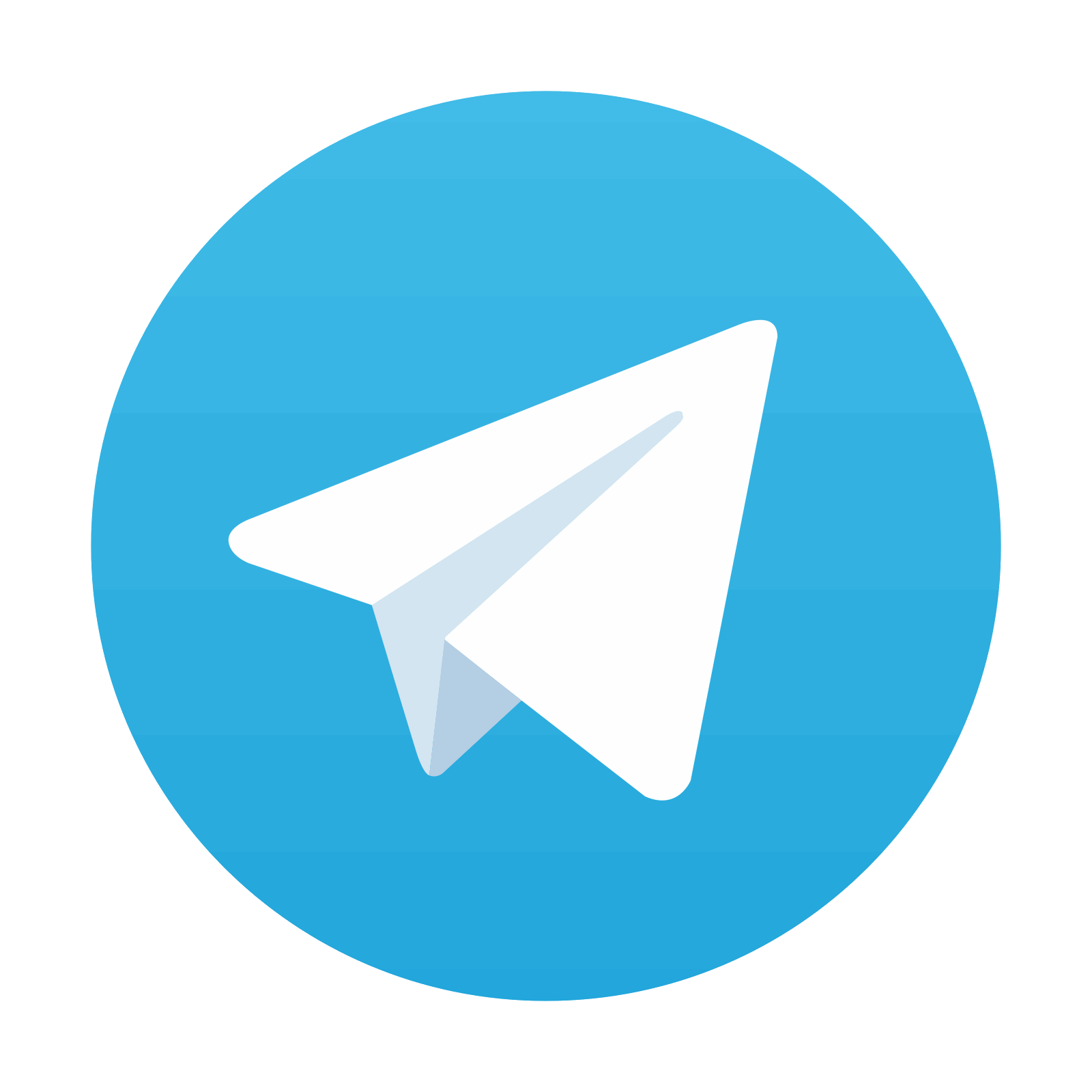
Stay updated, free articles. Join our Telegram channel

Full access? Get Clinical Tree
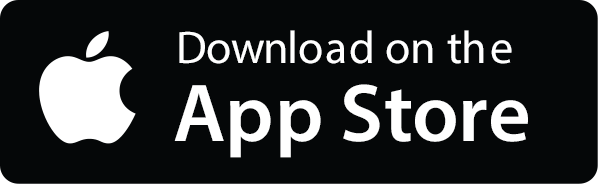
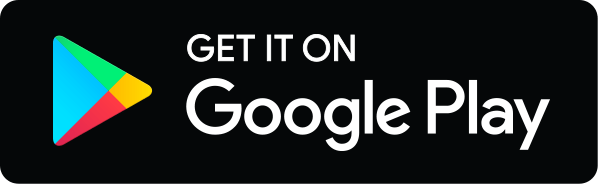
