Ultrasound Evaluation of the Portal and Hepatic Veins
Leslie M. Scoutt
Margarita V. Revzin
Hjalti Thorisson
Ulrike M. Hamper
Portal hypertension (PHT) is an extremely common medical problem worldwide. In Western countries, PHT most commonly occurs secondary to underlying liver cirrhosis, either viral or alcohol induced. Morbidity is primarily related to bleeding from gastroesophageal (GE) varices and liver failure. However, these patients are also at increased risk for developing hepatocellular carcinoma (HCC). Although ultrasound cannot directly assess portosystemic pressure gradients, ultrasound remains the most readily accessible, least invasive, and least expensive imaging modality available for screening patients for signs of PHT and HCC. Furthermore, there are no known risks associated with ultrasound examination of the liver. Ultrasound is also an excellent means of evaluating patients for portal vein (PV) thrombosis and Budd-Chiari syndrome (BCS), which may precipitate acute deterioration of liver function in patients with cirrhosis and HCC. In addition, duplex Doppler ultrasound has an important clinical role in monitoring patients following placement of transjugular intrahepatic portosystemic shunts (TIPS), which may serve as an adjunct to medical therapy for the treatment of GE varices or refractory ascites.
PATHOPHYSIOLOGY OF PORTAL HYPERTENSION
PHT is generally defined as an increase in the portosystemic pressure gradient of more than 10 mm Hg. In most cases, the increased portosystemic gradient is largely due to an increase in resistance to portal venous flow or increased peripheral vascular resistance secondary to a combination of morphologic abnormalities as well as circulating vasoconstrictors. The body compensates for the decrease in portal flow by increasing splanchnic blood flow through volume retention, circulating vasodilators, and increased sympathetic tone. Unfortunately, these physiologic adaptations, described as the hyperdynamic circulation, increase congestion in the liver and worsen the portosystemic gradient. The ultimate result of the increased portosystemic gradient is reversal of flow in the PV and the development of portosystemic collaterals. Portosystemic collaterals or varices may arise from dilatation of preexisting portosystemic vascular channels or from development of new blood vessels (angiogenesis). Splenomegaly and ascites are also common findings in patients with PHT. Hepatic encephalopathy may develop as liver function deteriorates and the liver is no longer able to metabolize the ammonia and manganese absorbed by the blood draining the bowel.
Patients with cirrhosis are at increased risk for developing HCC. The incidence of HCC in patients with cirrhosis is estimated at 3% per year, and patients with cirrhosis due to hepatitis B, hepatitis C, and hemochromatosis seem to have the highest risk for developing HCC. On the other hand, patients with cirrhosis secondary to autoimmune hepatitis, nonalcoholic steatohepatitis, and Wilson’s disease are at less risk.1,2 Hence, patients with cirrhosis are often screened at yearly intervals with ultrasound for HCC and signs of PHT. In addition, if a patient with previously compensated cirrhosis presents with acute decompensation of liver function, workup to exclude HCC or portal/hepatic vein (HV) thrombosis should be considered.2
PV pressure is optimally compared to right atrial pressure. A normal gradient is less than 7 mm Hg; a gradient between 7 and 10 mm Hg is consistent with mild PHT; GE varices typically do not form until the portosystemic gradient is greater than 10 to 12 mm Hg. Once the portosystemic gradient exceeds 12 to 15 mm Hg, the risk of variceal bleeding is substantially increased.3,4 As the morphologic features obstructing portal venous outflow may not be evenly distributed throughout the liver parenchyma, the portosystemic gradient may vary in different hepatic segments.5
Many classification systems have been proposed for describing PHT. The most common classification subdivides PHT into prehepatic, intrahepatic, and posthepatic causes (Table 22.1) based on the primary site of increased resistance to portal blood flow. Intrahepatic PHT is often further subdivided into presinusoidal, sinusoidal, and postsinusoidal causes.6 However, in many cases, increased resistance to flow may occur at more than one site. Hence, there is some overlap within this classification system. Worldwide, the most common cause of PHT is believed to be schistosomiasis.7 In the United States, alcohol-induced cirrhosis and viral-induced cirrhosis are the most common causes of PHT.7 Hyperdynamic PHT is the least common type.
TABLE 22.1 CLASSIFICATION OF PORTAL HYPERTENSION | |||||
---|---|---|---|---|---|
|
SCANNING TECHNIQUE AND NORMAL ANATOMY
Duplex ultrasound (DUS) evaluation of the hepatic vasculature is best performed using a 2- to 5-MHz curved array transducer in a fasting (8 to 12 hours) patient. Gray-scale imaging of the liver is initially performed and is optimized by using harmonic imaging, spatial compounding, and multiple acoustic windows. The size, echotexture, and surface contour of the liver should be noted and focal liver lesions excluded. The use of a high-frequency (5 to 10 MHz) linear array transducer may aid in evaluating the liver surface for nodularity suggestive of cirrhosis. The spleen is evaluated for size and focal lesions, and the upper abdomen is screened for ascites and varices. Oblique imaging, aiming toward the right shoulder, is particularly useful for visualization of the main PV. The porta hepatis is often best visualized in the right decubitus position, as artifact from bowel gas will be eliminated or reduced when the patient turns on the right side, allowing gas in the bowel to rise into the gastric fundus in the left upper quadrant and fluid in the bowel to collect in the gastric antrum and duodenum providing a good acoustic window. When evaluating the hepatic vasculature with pulsed Doppler, spectral waveforms should be obtained from the following vessels: upper abdominal aorta; main, right, and left hepatic arteries; main, right, and left PVs; splenic vein (SV) at the splenic hilum and under the pancreas; superior mesenteric vein (SMV) at the portal confluence; right, middle, and left HVs; and inferior vena cava (IVC). Peak systolic velocity (PSV) is measured in the hepatic arteries as well as in the aorta, and peak velocity is measured in the PVs. Optimally, at least three Doppler spectral waveforms should be obtained in the main PV and main hepatic artery (HA). The highest velocity is recorded. Machine settings will vary depending upon the vessel examined. When evaluating the PVs, settings should be optimized for the detection of slow flow. The color box should be small; the pulse repetition frequency (PRF) or scale should be set as low as possible before aliasing occurs; the wall filter should be as low as possible until motion artifact occurs; and the gain should be maximized until artifact from noise obscures the image. Spectral Doppler waveforms from the portal and HVs should be obtained during quiet respiration, as deep inspiration will flatten venous waveforms and decrease PV velocity. However, when evaluating the HA, the PRF and wall filter will need to be increased to avoid aliasing and motion artifact. When measuring PSV with spectral Doppler, the angle of insonation should be 60 degrees or less and the sample volume should encompass the width of the HA. The PRF should be adjusted such that the Doppler waveform is as large as possible before aliasing occurs. The PV contributes approximately 75% of the blood supply to the liver and is formed by the confluence of the splenic and SMVs. The Doppler spectral waveform from a normal PV demonstrates low-velocity continuous hepatopetal flow. Peak velocity ranges from 10 to 40 cm/s, and normal PV flow demonstrates mild respiratory variation (Fig. 22.1A). During quiet respiration, the PV is normally less than 13 mm in diameter.8,9 However, diameter and velocity are inversely related. Hence, due to increased intra-abdominal pressure with deep inspiration, PV diameter increases, reaching a maximum of 16 mm, and velocity decreases slightly.8,9,10,11 Occasionally, PV flow may be more pulsatile in the normal patient (see Fig. 22.1B) as cardiac pulsatility is transmitted through the HVs and hepatic sinusoids to the PVs. Contraction of the right atrium at end diastole transmits back pressure to the portal circulation, decreasing forward portal venous flow. Thus, the trough in a pulsatile portal venous waveform corresponds to end diastole.11 Flow in the SV and SMV is hepatopetal in direction and also demonstrates mild respiratory variation, as well as an increase in diameter on deep inspiration.10 In most patients, the celiac artery bifurcates into the common HA and the splenic artery (see Fig. 22.2A). The common HA courses in the hepatoduodenal ligament toward the liver, lying anterior and medial to the PV and inferior to the common bile duct (see Fig. 22.2B). However, in approximately 11% of patients, the right HA arises from the superior mesenteric artery (SMA), and in 4% of patients, the common HA will arise from the SMA. Replaced right or common hepatic arteries course inferior and lateral to the PV. The spectral waveform from the HA demonstrates a sharp systolic upstroke with continuous forward diastolic flow (see Fig. 22.2B). PSV is related to systemic blood pressure and is typically around 100 cm/s, and the resistive index (RI) of the HA normally ranges between 0.55 and 0.7.11 The right, middle, and left HVs typically join the IVC near the dome of the liver; however, congenital variations of HV anatomy are common. Normal HV and IVC spectral waveforms are pulsatile, reflecting the variations in right atrial pressure during the cardiac cycle. Flow is primarily directed away from the liver capsule (Fig. 22.3A). The initial S wave is due to filling of the right atrium in early to mid systole. This is followed by the V wave due to overfilling of the right atrium at the end of systole just before the tricuspid valve opens. The subsequent D wave reflects filling of the right atrium in diastole. Lastly, there is a brief reversal of flow heading back toward the liver, the A wave, which corresponds to the right atrial “kick” or contraction at end diastole (see Fig. 22.3B). These changes in HV velocity are extremely sensitive to intra-abdominal pressure. Therefore, if a spectral waveform is obtained in deep inspiration, or if the IVC is compressed by an enlarged liver, ascites, or a large amount of fat in the anterior abdominal wall, the HV and IVC waveforms in may appear artifactually flattened.
ULTRASOUND FINDINGS IN PORTAL HYPERTENSION
Patients with PHT frequently develop splenomegaly (>13 cm in maximal diameter) and ascites (Fig. 22.4). Gamna-Gandy bodies (siderotic nodules containing fibrous tissue, hemosiderin, and calcium salts) may be identified in the spleen
as multiple punctate echogenic foci (Fig. 22.5).12 In the liver, the stigmata of cirrhosis, namely volume redistribution with a decrease in size of the right lobe and relative increase in size of the caudate lobe and lateral segment of the left lobe, coarse echotexture, surface nodularity, and focal masses may be noted (Fig. 22.6). Any focal mass noted on DUS in a patient with cirrhosis should be presumed to be HCC, irrespective of size and echogenicity, until proven otherwise (see Fig. 22.6E and F). Although regenerative nodules are the most common cause of focal liver lesions in patients with cirrhosis, they are uncommonly visualized on ultrasound examination. While cirrhosis is the most common cause of PHT in the United States, it should be remembered that cirrhosis can be present in the absence of PHT, and PHT can occur without cirrhosis.
as multiple punctate echogenic foci (Fig. 22.5).12 In the liver, the stigmata of cirrhosis, namely volume redistribution with a decrease in size of the right lobe and relative increase in size of the caudate lobe and lateral segment of the left lobe, coarse echotexture, surface nodularity, and focal masses may be noted (Fig. 22.6). Any focal mass noted on DUS in a patient with cirrhosis should be presumed to be HCC, irrespective of size and echogenicity, until proven otherwise (see Fig. 22.6E and F). Although regenerative nodules are the most common cause of focal liver lesions in patients with cirrhosis, they are uncommonly visualized on ultrasound examination. While cirrhosis is the most common cause of PHT in the United States, it should be remembered that cirrhosis can be present in the absence of PHT, and PHT can occur without cirrhosis.
Ultrasound findings of PHT progress as the portosystemic gradient increases. Initially, the diameter of the PV increases: greater than 13 mm in quiet respiration and greater than 16 mm in deep inspiration (Fig. 22.7A). This change in PV diameter has been reported to have a very high specificity (near 100%) though a low sensitivity (˜45% to 50%) for PHT.13,14 In addition, the PV, SV, and SMV will not increase in diameter with deep inspiration in patients with PHT. A less than 20% increase in the diameter of the SV and SMV following deep inspiration has been reported to have a sensitivity of 81% and specificity of 100% for the diagnosis of PHT.10,11,12,13,14 On spectral Doppler interrogation, loss of respiratory variation occurs in the PV, and the waveform will flatten. As PV diameter increases, velocity decreases (see Fig. 22.7B). Eventually, bidirectional flow (above and below the baseline) will be observed (see Fig. 22.7C), and ultimately flow in the PV will reverse, becoming hepatofugal (see Fig. 22.7D).15,16,17,18 As flow in the PV decreases, flow in the HA typically increases, and the HA becomes dilated and tortuous with a so-called corkscrew appearance (Fig. 22.8). Waveforms in the HVs may also flatten (Fig. 22.9).19
However, none of these progressive findings in the PV, HA, or HVs directly correlate with specific measurements of the portosystemic gradient, and not all of the above findings are specific for PHT. The most common pitfall in DUS evaluation of the PV is poor visibility due to increased depth secondary to a large liver or ascites. In some patients, flow in the PV may be of such low velocity and volume that it cannot be detected by pulsed Doppler despite optimization of imaging parameters, thereby mimicking PV thrombosis. Dilatation of the PV is a nonspecific finding and can be seen with congestive heart failure, although in patients with heart failure the waveform in the PV is typically pulsatile, and dilatation of the IVC and HVs is common with prominence of the A wave. Furthermore, the absence of the findings described above cannot be used to exclude the possibility of PHT. Therefore, Doppler ultrasound cannot be used to assess the severity or progression of PHT.
The most specific finding of PHT is the visualization of portosystemic shunts or varices, although occasionally collateral vessels may develop secondary to mesenteric or SV thrombosis. Varices may develop secondary to reversal of flow in existing veins or recanalization of embryonic channels. Up to 65% to 90% of varices can be visualized on DUS, provided one looks systematically throughout the abdomen and retroperitoneum (Fig. 22.10).15,20,21,22
Identification of GE varices is critical, as rupture of GE varices may result in death if bleeding cannot be controlled. It is estimated that 30% to 40% of patients with Child-Pugh class A compensated cirrhosis have GE varices, and up to 60% to 65% of patients with Child-Pugh classes B and C.23,24 Hence, the incidence of varices appears to be related to the severity of the liver disease. Additionally, the risk of bleeding from GE varices is directly related to the portosystemic pressure gradient. Once the portosystemic gradient exceeds 12 to 15 mm Hg, the risk of variceal bleeding is substantially increased.3,4 Endoscopic screening is the most sensitive way of identifying GE varices, and some groups recommend that patients with established cirrhosis be routinely screened with endoscopy.25,26 However, such screening is invasive and costly. On ultrasound, GE varices are most easily found by locating the echogenic diaphragm near the aorta in a sagittal plane and imaging with color Doppler (Fig. 22.11). Alternatively, one may follow the coronary vein cephalad toward the GE junction from the portal confluence. The coronary vein runs craniocaudally in a plane parallel to the SMV or aorta and joins the SV from above, usually just medial and superior to the confluence of the SV with the SMV. The coronary vein is considered abnormal if flow is heading toward the head or if the diameter is greater than 4 mm. The risk of bleeding from GE varices increases if the coronary vein is greater than 7 mm in diameter.15,27
The paraumbilical vein runs in the ligamentum teres between the medial and lateral segments of the left lobe of the liver (Fig. 22.12). It extends from the left PV toward the capsule of the liver, continues anteriorly and superficially coursing under the anterior abdominal wall, and drains into the inferior epigastric veins. Blood returns to the systemic circulation via the external iliac vein. A paraumbilical vein is considered abnormal
if it is greater than 3 mm in diameter or if flow extends beyond the liver capsule. A patent vein in the ligamentum teres less than 2 mm in diameter can be seen in some normal patients and can even demonstrate low-velocity (<5 cm/s) flow away from the liver capsule.28,29,30 If a patient with PHT develops a patent paraumbilical vein, flow often remains hepatopetal in the main PV despite elevation of the portosystemic gradient. However, a patent paraumbilical vein does not significantly decompress the liver or reduce the portosystemic gradient. Hence, patients with patent paraumbilical veins remain at risk for developing GE varices.
if it is greater than 3 mm in diameter or if flow extends beyond the liver capsule. A patent vein in the ligamentum teres less than 2 mm in diameter can be seen in some normal patients and can even demonstrate low-velocity (<5 cm/s) flow away from the liver capsule.28,29,30 If a patient with PHT develops a patent paraumbilical vein, flow often remains hepatopetal in the main PV despite elevation of the portosystemic gradient. However, a patent paraumbilical vein does not significantly decompress the liver or reduce the portosystemic gradient. Hence, patients with patent paraumbilical veins remain at risk for developing GE varices.
Short gastric or left gastric varices are found below the left lobe of the liver (Fig. 22.13). In patients with spontaneous splenorenal shunts, a direct communication, often “U-shaped,” will be observed between the splenic and left renal veins (Fig. 22.14). The left renal vein is typically dilated and flow will be reversed in the main PV. Spontaneous portocaval shunts are less common, but if large are associated with focal dilatation of the IVC at the level of the shunt as well as hepatic encephalopathy, since a large amount of blood draining from the bowel will be shunted into the systemic circulation without detoxification by the liver. Surgical shunts appear similar to spontaneous shunts on DUS examination. Although these are the most readily ultrasound-detected variceal patterns, varices can occur virtually anywhere in the retroperitoneum or abdomen (Figs. 22.15 and 22.16).
It is estimated that approximately one-third of patients with GE varices will present with significant variceal bleeding.24,31,32 Risk factors for hemorrhage include large varices, a portosystemic gradient greater than 12 to 15 mm Hg, a coronary vein greater than 7 mm in diameter, ascites, acute alcohol binge, and exercise.3,4,15,33 Medical management of significant bleeding from GE varices includes endoscopic sclerotherapy, variceal band ligation, vasoconstrictors (vasopressin, nitroglycerin, somatostatin, and octreotide), and balloon tamponade. Bleeding is controlled by a combination of endoscopic and pharmacologic treatment in up to 80% to 90% of cases.24,32,34,35,36 However, approximately 70% of patients will rebleed,35,36,37 and the mortality rate in such patients is extremely high (30% to 40%) despite aggressive medical management including prophylactic treatment with β-blockers.35,36,37,38
For patients with persistent uncontrolled bleeding from GE varices or recurrent bleeding following medical management, the next step is placement of a TIPS. The role of TIPS placement in managing patients with cirrhosis has evolved over the past few decades, and currently, TIPS placement is preferred over creation of a surgical shunt as it avoids general anesthesia and major abdominal surgery. In addition, TIPS placement does not alter the extrahepatic vascular anatomy, allowing a patient to remain on the liver transplantation list for definitive treatment of liver disease. The most common current indications for TIPS placement are secondary prevention of variceal bleeding, variceal bleeding refractory to medical and endoscopic therapy, and ascites refractory to medical management.39 Less frequent but also commonly accepted indications for TIPS placement include hepatorenal syndrome, BCS, hepatic venoocclusive disease, and hepatic hydrothorax.39 Generally accepted absolute contraindications to TIPS placement include severe right heart failure (as shunting of significant blood volume into the IVC through the TIPS may produce volume overload and precipitate cardiac decompensation), severe tricuspid regurgitation (TR), severe pulmonary hypertension (mean pulmonary pressure >45 mm Hg), and uncontrolled sepsis.39 Other often accepted contraindications to TIPS placement include preexisting severe hepatic encephalopathy (as creation of a TIPS may worsen the encephalopathy), inadequate liver reserve (as TIPS placement may result in fulminant liver failure on the basis of ischemia in such patients), sepsis, and multiple hepatic cysts (due to lack of adequate surrounding liver parenchyma that is necessary to keep the TIPS stable in location).39 Relative contraindications, while dependent upon the aggressiveness of the interventionist and the expected length of patient survival, include HCC (especially in the right lobe or central in location), biliary obstruction (due to risk of bile leak), and moderate pulmonary hypertension.39 The presence of PV thrombosis makes TIPS placement difficult although not impossible.40
Most centers report close to a 90% technical success rate for TIPS placement,41,42,43 with bleeding controlled in up to 90% of patients43,44,45,46 and an approximately 2% mortality rate.47 The complication rate ranges in most series from approximately 10% to 16%.45,46 Factors that increase post-TIPS mortality include Childs-Pugh class C, emergent placement for acute uncontrolled variceal hemorrhage, serum bilirubin greater than 3 mg/dL, alanine aminotransferase levels greater than 100 IU/L, and pre-TIPS encephalopathy.48 More recently, the Model for End-Stage Liver Disease (MELD) score, which is based on serum creatinine, INR, and bilirubin levels, has become a widely used standard to predict 3-month post-TIPS mortality. A score greater than 18 is associated with significantly increased mortality.49,50,51 Causes of acute morbidity include hemorrhage from transcapsular puncture, which may occur in up to 33% of cases,39 stent migration,
cardiopulmonary failure (due to volume overload), infection, and hepatic encephalopathy. Hepatic encephalopathy is most likely caused by shunting of ammonia and other potentially toxic metabolites directly into the systemic circulation without being detoxified by the liver. The larger the TIPS diameter, the more blood is shunted through the liver and the higher the risk of hepatic encephalopathy. Shunt reduction or occlusion may be required if the patient does not respond to protein restriction, lactulose, and vancomycin therapy.
cardiopulmonary failure (due to volume overload), infection, and hepatic encephalopathy. Hepatic encephalopathy is most likely caused by shunting of ammonia and other potentially toxic metabolites directly into the systemic circulation without being detoxified by the liver. The larger the TIPS diameter, the more blood is shunted through the liver and the higher the risk of hepatic encephalopathy. Shunt reduction or occlusion may be required if the patient does not respond to protein restriction, lactulose, and vancomycin therapy.
Recurrent variceal bleeding following successful TIPS placement is usually due to TIPS malfunction from stenosis, thrombosis, or stent retraction. Stenoses within a TIPS usually occur at the hepatic end. Causes of early TIPS stenoses have been postulated to include biliary-TIPS fistulae due to thrombogenic and inflammatory factors in the bile, kinking, or other technical factors.52 Later TIPS stenoses or thromboses are most commonly caused by pseudointimal hyperplasia and turbulent blood flow, which may cause endothelial damage resulting in an outflow stenosis in the draining HV.52 TIPS stenoses and thromboses are relatively common when “bare” stents are used. Primary patency rates for bare stents are reported to be in the range of 25% to 78% at 1 year39,43,45,53,54 and as low as 13% at 5 years.53 If stenoses in bare stents are corrected, the assisted patency rate is higher, close to 85% at 1 year41,45 and 36% at 5 years.53
The recent introduction of the partially covered Viatorr polytetrafluoroethylene (PTFE) stent graft for TIPS creation
has significantly improved primary patency, with a reported 6-month patency rate of up to 90% and a 24-month patency rate of up to 75%.55,56,57 The Viatorr stent graft has a 2-cm segment of bare metal stent that is placed in the PV and a variable length of PTFE-covered stent extending to the HV-IVC confluence. Use of the partially covered Viatorr stent graft has now become standard of care for TIPS creation. However, even with the partially covered stent graft, identifying an underlying stenosis in a TIPS before it becomes symptomatic is of the utmost clinical importance, as reinter-vention and revision may prevent TIPS failure. Since most TIPS stenoses are found in asymptomatic patients, surveillance is still often recommended.58 DUS is the least invasive and least expensive method for screening patients for TIPS stenoses. A baseline examination followed by DUS at 6 months, 12 months, and then annually is considered a reasonable surveillance schedule.40 However, because the primary patency rate of PTFE-covered stents is so high, it remains controversial whether or not long-term surveillance with DUS is necessary.59
has significantly improved primary patency, with a reported 6-month patency rate of up to 90% and a 24-month patency rate of up to 75%.55,56,57 The Viatorr stent graft has a 2-cm segment of bare metal stent that is placed in the PV and a variable length of PTFE-covered stent extending to the HV-IVC confluence. Use of the partially covered Viatorr stent graft has now become standard of care for TIPS creation. However, even with the partially covered stent graft, identifying an underlying stenosis in a TIPS before it becomes symptomatic is of the utmost clinical importance, as reinter-vention and revision may prevent TIPS failure. Since most TIPS stenoses are found in asymptomatic patients, surveillance is still often recommended.58 DUS is the least invasive and least expensive method for screening patients for TIPS stenoses. A baseline examination followed by DUS at 6 months, 12 months, and then annually is considered a reasonable surveillance schedule.40 However, because the primary patency rate of PTFE-covered stents is so high, it remains controversial whether or not long-term surveillance with DUS is necessary.59
Duplex Follow-up of TIPS Procedures
The DUS imaging protocol for patients with TIPS should include evaluation for recurrent varices or ascites, since recurrence suggests that the TIPS is not working effectively and is likely to be stenosed or occluded. Direct interrogation of the shunt typically requires a 2.5-MHz curved array transducer, as the TIPS is usually placed deep in the right lobe of the liver. Doppler interrogation requires numerous acoustic windows and velocity scales. Higher scales are required for interrogation of the TIPS than for the intrahepatic veins because velocities are typically substantially higher within a normally functioning TIPS. PV and shunt velocity will decrease with deep inspiration, so velocity should be measured at the end of gentle respiration. Velocities should be obtained from the main PV 2 to 3 cm proximal to the TIPS, in the proximal, mid, and distal shunt, and in the IVC or draining vein. Direction of flow should be noted in the main and intrahepatic portal, splenic, and hepatic veins.
The stent walls are very echogenic and should be smooth and parallel. When covered stents are first placed, air embedded in the covering fabric typically causes dense shadowing along the entire length of the stent, preventing Doppler interrogation and mimicking thrombosis (Fig. 22.17).40,60 This air is gradually absorbed, and the shadowing disappears within 2 to 3 days, allowing ultrasound evaluation. Color should fill the lumen of the stent and extend to the echogenic walls (Fig. 22.18A). Spectral Doppler findings in patients with TIPS are variable and likely depend on the cause and severity of PHT, the diameter and type of stent graft used, and the residual portosystemic gradient following TIPS placement. In general, flow velocity is high in the shunt, ranging from 100 to 200 cm/s, and there is little change over the length of the shunt in a given patient (see Figs. 22.18B-D). Increased flow velocity (>40 cm/s) will be observed in the main PV (see Fig. 22.18E), and flow in the HA is also typically increased (>130 cm/s).45,46,61,62,63,64,65 Optimally, the gradient across the shunt following TIPS placement will be less than the residual gradient in the liver parenchyma and, therefore, flow will be directed toward the shunt (hepatofugal) in the right and left intrahepatic PVs (see Fig. 22.18F).40 However, depending upon technical success and the effect of reduced hepatic congestion on the residual portosystemic gradient in the liver parenchyma, in some patients, the residual parenchymal gradient may not be higher than the gradient across the TIPS in all segments of the liver. In such patients, flow may not reverse in all of the intrahepatic PVs. For this reason, a baseline examination of the intrahepatic PVs is extremely important to assess the direction of flow.
Reappearance of varices, refractory ascites, and pleural effusion are associated with TIPS occlusion or stenosis. Lack of detectable flow on color and pulsed Doppler interrogation, in combination with intraluminal echoes, is highly accurate in diagnosis of TIPS thrombosis or occlusion (Fig. 22.19), although occasionally it may be difficult to demonstrate flow within a TIPS if it is located too deep in the abdomen.45,46,60,61,62,63,64,65 Reported DUS diagnostic criteria for TIPS stenoses vary from institution to institution, likely in part related to differences in patient population and the type and diameter of TIPS shunt, as well as angiographic and DUS technique.40 Velocity less than 30 cm/s in the main PV, velocity less than 50 to 60 cm/s within the TIPS shunt, a focal increase in velocity of greater than 200 to 250 cm/s in the TIPS, and a focal increase or decrease in velocity of greater than 50 cm/s over time (from one study
to the next) or spatially along the length of the TIPS are the velocity criteria most often used to diagnose TIPS stenoses (Figs. 22.20 and 22.21).45,46,61,62,63,64,65 In addition, a focal narrowing on color or power Doppler imaging with color aliasing or intraluminal thrombus may be noted (see Fig. 22.20A). A change in direction of flow from hepatofugal to hepatopetal in the left or right PVs (see Fig. 22.20D), or from hepatopetal to hepatofugal in the main PV, has been reported as a relatively late finding of TIPS malfunction and indicative that the gradient across the TIPS is higher than the portosystemic gradient in the liver parenchyma.40,63 No single Doppler criterion has been reported to have adequate sensitivity or positive predictive value for the diagnosis of TIPS stenosis; however, sensitivity increases when multiple criteria are used.65
to the next) or spatially along the length of the TIPS are the velocity criteria most often used to diagnose TIPS stenoses (Figs. 22.20 and 22.21).45,46,61,62,63,64,65 In addition, a focal narrowing on color or power Doppler imaging with color aliasing or intraluminal thrombus may be noted (see Fig. 22.20A). A change in direction of flow from hepatofugal to hepatopetal in the left or right PVs (see Fig. 22.20D), or from hepatopetal to hepatofugal in the main PV, has been reported as a relatively late finding of TIPS malfunction and indicative that the gradient across the TIPS is higher than the portosystemic gradient in the liver parenchyma.40,63 No single Doppler criterion has been reported to have adequate sensitivity or positive predictive value for the diagnosis of TIPS stenosis; however, sensitivity increases when multiple criteria are used.65
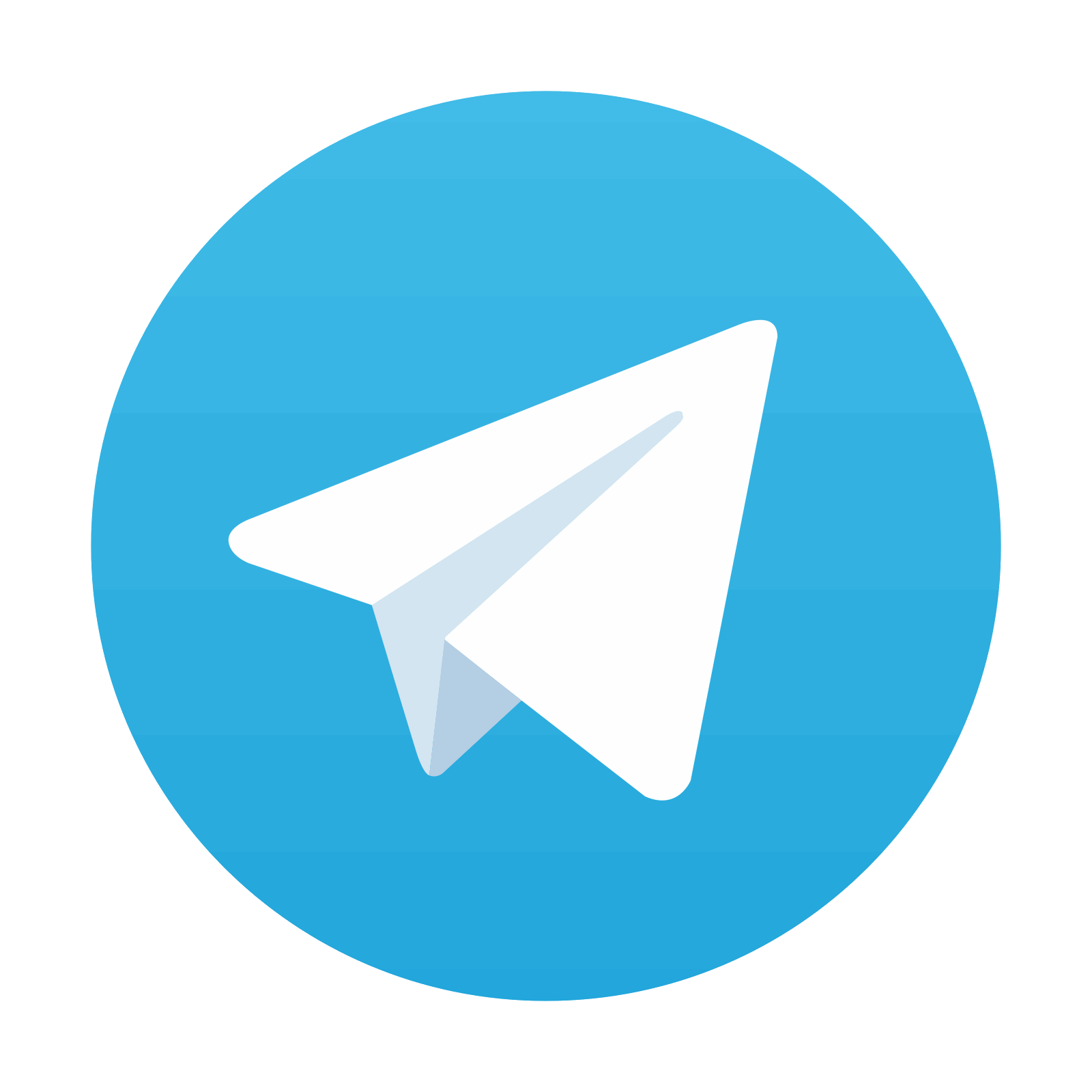
Stay updated, free articles. Join our Telegram channel

Full access? Get Clinical Tree
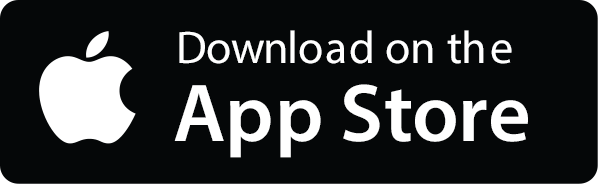
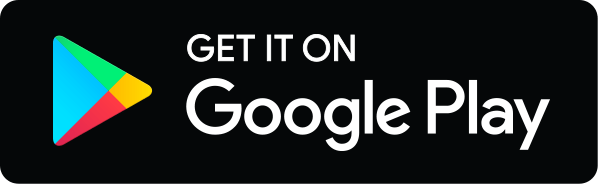