Ultrasound Device Settings, Examination Technique, and Artifacts
2.2Transducer Selection and Instrument Settings
2.3Examination Technique, Limitations, and Artifacts
2.4Effect of Imaging Technique on Spatial Resolution and Lesion Detectability
2 Ultrasound Device Settings, Examination Technique, and Artifacts
2.1 Introduction
Color flow imaging (CFI) combines the pulse-echo technique of B-mode imaging with the capabilities of functional evaluation by Doppler ultrasound. Other techniques such as power Doppler, B-flow imaging, and contrast-enhanced ultrasound have broadened the range of diagnostic capabilities. Each of these procedures has special technical and equipment characteristics that must be matched to the organ or vascular region of interest and to the clinical question that the study is intended to address.
2.1.1 Color Flow Imaging (CFI)
Usually, the first step in an ultrasound examination is to obtain an optimum B-mode image. Then the desired technique for flow imaging is selected:
•Generally, it is best to start with color flow. Doppler spectra can then be selectively sampled depending on the clinical question and findings.
•Power Doppler (power mode, energy mode) can help to detect weak signals in examinations of the kidneys or tumor blood flow.
•B-flow is calculated from the whole US image, a “color” box is not needed. Advantages are high special and time resolution, less artifacts, like blooming or aliasing. Due to the subtraction technique, stationary echoes are eliminated, strong moving echoes like bowel gas or swirling of tissue due to a high-grade stenosis that may cause typical, time dependent artifacts.
•Depending on the number of scatters and their velocity, CFI can only image vascularization whereas US contrast agent can image the perfusion and is thus much more sensitive compared to all the other CFI techniques.
This chapter will deal with operator-controlled parameters that are most important for the optimum utilization of various sonographic techniques, the analysis of flow information, and the identification of artifacts.
2.2 Transducer Selection and Instrument Settings
2.2.1 Prerequisites
The basis for all techniques is B-mode ultrasound, which can provide a detailed morphologic depiction of the vessel wall and lumen by virtue of increasingly higher resolution and frame rates. The echoes displayed in the ultrasound image are generated by reflections at interfaces between tissues with different acoustic impedance. If the resistance to sound transmission is low, the acoustic energy will have sufficient penetration for imaging tissues at deeper levels. If large impedance differences are encountered at interfaces such as tissue–air or tissue–bone, almost all of the sound energy will be reflected and deeper objects will not be visualized.
Reflection is an angle-dependent process. For example, when the beam strikes the vessel wall at a 90 degrees angle, that portion of the wall will appear brighter and thicker than the rest of the wall, an effect known as blooming artifact, which is an important artifact and should always be considered in CFI. When the beam strikes an interface at angles other than 90 degrees, only a portion of the wave energy will be returned to the transducer.
When the sound wave strikes a reflective or rough interface that are small relative to the incident wavelength, a great many randomly directed echoes are generated due to a process called scattering. In addition to losses caused by reflection and scattering, some of the sound energy is absorbed in a frequency-dependent fashion due to frictional and relaxation forces in the medium. As a result, the sound pressure declines, but is under normal tissue conditions. The estimated loss will be automatically compensated depending on the depth of the organ or ROI. The degree of this amplitude loss, called attenuation, depends on the distance traveled, the transmission frequency, and patient-specific, sound-conduction properties.
2.2.2 Transducer Selection
The selection of a transducer depends on the clinical question and the anatomic region of interest. The main types of transducer are linear arrays, curved arrays, and phased arrays. The main criteria for selecting a particular type are the necessary field-of-view width, penetration depth, and desired spatial resolution. The selection of transducer frequency is a tradeoff between the required resolution and necessary penetration depth.
2.2.3 Transducers
If a large near-field width of view is desired, say for scanning the carotid artery or limb arteries, the best choice would be a linear-array transducer, also called a linear array (see Chapter 7.2.1.3, 7.2.2.3, and 7.3.3).
Linear arrays are available with various types of dynamic focusing or mechanical focusing with acoustic lenses (e.g., Hanafy lens). Matrix arrays are also available and are selected according to desired imaging depth, geometric resolution, and tissue-contrast resolution (Fig. 2.1).
The curved-array transducer (curved array) combines high near-field resolution with a wider far field of view than that of a linear array (Fig. 2.2). It provides the best compromise for abdominal imaging (Chapter 11, Chapter 12, and Chapter 14) and for certain examinations of peripheral veins in the thigh or calf.
Fig. 2.1 High-resolution linear array. Longitudinal scan of the common carotid artery from the lateral side of the neck. The image clearly depicts the intima-media layer in the near and far vessel walls, permitting an accurate measurement of carotid intima-media thickness (CIMT).
A phased-array transducer (phased array) has a very small footprint that is excellent for scanning through a small acoustic window as in echocardiography, transcranial Doppler ultrasound, and scanning of the renal arteries through a lateral intercostal approach. Newer scanners have a trapezoidal mode that increases the width of the far field, similar to a curved array, while maintaining good near-field resolution.
Fig. 2.2 Curved array with an optimized B-mode image (liver parenchyma with hepatic veins). The parenchyma shows uniform echogenicity with increasing depth in this subcostal oblique scan. The hepatic veins and diaphragm are sharply defined.
Transducer Frequency
The band width of the transmitted frequencies depends on the transducer. It correlates with the spatial resolution from near to far field. The greater the width of frequencies, the better the near field resolution will be and vice versa. High transducer frequencies are best for the high-resolution imaging of superficial structures such as lymph nodes, thyroid gland, and breast (9–24 MHz) and for supra-aortic arteries (7–14 MHz). Transducer frequencies in the 1 to 8 MHz range performs best for intra-abdominal imaging, depending on the desired penetration and imaging technique.
2.2.4 Optimizing the Image with Operator-Controlled Settings
B-Mode
First a normal-appearing area is imaged within the anatomic region of interest, depending on the specific application. The acoustic output (=power). In plane wave imaging a focaL point(s) are no longer needed. Focussing is managed on the receiving side. For abdominal imaging, these settings can be adjusted in a longitudinal scan of the hepatic left lobe and aorta or in a subcostal transverse scan through the liver (Fig. 2.2). The initial power setting should be as low as possible. Then the TGC control is adjusted to obtain a balanced image. If this does not give adequate visualization of all tissue areas, the power setting should be increased.
Vessel lumina should appear hypoechoic or echo-free, and the intima-media layer should be well defined in examinations of the carotid artery and limb arteries (Fig. 2.1). Speckle noise can be reduced by tissue harmonic imaging (THI), by compound imaging based on scanning at multiple angles (spatial compounding, marketed as SonoCT, SieClear, and XBeam), or by scanning at different frequencies (frequency compounding) in order to improve image quality (Fig. 2.3).
Fig. 2.3 Artifact suppression by tissue harmonic imaging (THI). Longitudinal scan through the common carotid artery shows generalized wall thickening due to vasculitis. The image on the left, acquired in the fundamental mode, shows significant scattering and speckle artifacts. In the image on the right, acquired in THI mode, the wall changes are sharply delineated with almost no artifacts.
Other settings such as dynamic range, the summation of old and new information (persistence, frame averaging), and postprocessing algorithms such as edge enhancement and changes in the gray scale curve should be saved as standard presets so that comparable images can be obtained in follow-up examinations (e.g., of tumors or changes in liver pattern during chemotherapy). For vascular imaging, it is generally best to obtain grainy images with low persistence and a lower dynamic range. The principal operator-controlled parameters are reviewed in Table 2.1.
Flow imaging techniques can be chosen either as continuous wave (= one dimensional perfomed with a pencil probe), pulsed wave (PW) Doppler including spectral analysis or two-dimensional techniques that can detect motion simultaneously in a great many spatially distributed volume elements and display that information in a two-dimensional sectional image (Chapter 1). While spectral Doppler plots the changes in velocity over time that are measured at one sampling site, CFI and power Doppler analyze the flow velocities or number of moving scatterers at a great many sampling sites distributed over all or part of the sectional image:
•CFI calculates the frequency-weighted modal frequency shift or mean velocity per volume element (Chapter 1). The results of this computation are color-coded and superimposed over the B-mode image. The colors—usually red and blue—indicate flow direction relative to the transducer. Brightness levels indicate the magnitude of the Doppler frequency shift or, when the beam-vessel angle is known, the flow velocity. Additionally, the variance of the frequency shift can be calculated as an indicator of possible turbulence. Variance may be shown as a separate parameter but is usually added to the flow information as green pixels when turbulence mode is enabled.
•In power Doppler (energy mode, power mode), the intensity of the signal from moving blood cells is usually encoded in varying shades of color. Some machines can add color-coded directional information (bidirectional power Doppler).
•B-flow imaging is a non-Doppler technique in which the change in the location of blood cells is determined from two or four encoded pulses that are successively transmitted along each scan line. The returning echo signals are subtracted from each other. Pixel brightness depends on the number of moving blood cells and, to a degree, on their velocity. Stationary tissue echoes may be completely discarded from the image or may be displayed faintly in the B-flow image for better anatomic orientation.
CW/PW Doppler with Spectral Analysis and CFI
Criteria for a high-quality color duplex image are homogeneous color filling of the vessel in at least one phase of flow, clear delineation of the vessel wall, plaque not overwritten by color, and an uncluttered spectrum with a clear spectral window (e.g., during systole in a large, nonstenosed artery; Fig. 2.4). The most important parameters for scan optimization are as follows:
•Power output
•Gain
•Pulse repetition frequency (PRF)
•Wall filter
•Color and gray scale priority
•Beam-vessel angle
These parameters are discussed jointly (see Table 2.1) due to their equivalent effects in CFI and one-dimensional Doppler techniques (CW and PW Doppler with spectral analysis).
The power output and color gain for signal reception should initially be increased until color noise appears. Then the color gain is decreased until a normal vascular segment shows no extraluminal overflow of color signals. Setting the color gain too low causes loss of color sensitivity (Fig. 2.5). Setting it too high fills the vessel lumen with color but also causes extraluminal color bleed with a decrease in resolution and slice thickness! This can cause the overwriting of possible hypoechoic wall changes. For example, tubes 1 mm in diameter may show inflow signals of up to 4 to 6 mm lateral to the transducer long axis, depending on the power setting and focal position. Vessel diameter is overestimated when viewed in cross section, and hypoechoic thrombi and wall changes may be overwritten. For this reason, classic compression sonography is still superior to CFI for the detection of thrombi in peripheral veins.
Fig. 2.4 Normal-appearing common carotid artery with all settings optimized. Criteria for evaluating the quality of the examination are: accurate display of color information in the vessel lumen with no extraluminal color bleed or aliasing, complete vessel fill-in in at least one flow phase, a Doppler spectrum free of noise and aliasing, and a clear spectral window at the normal site.
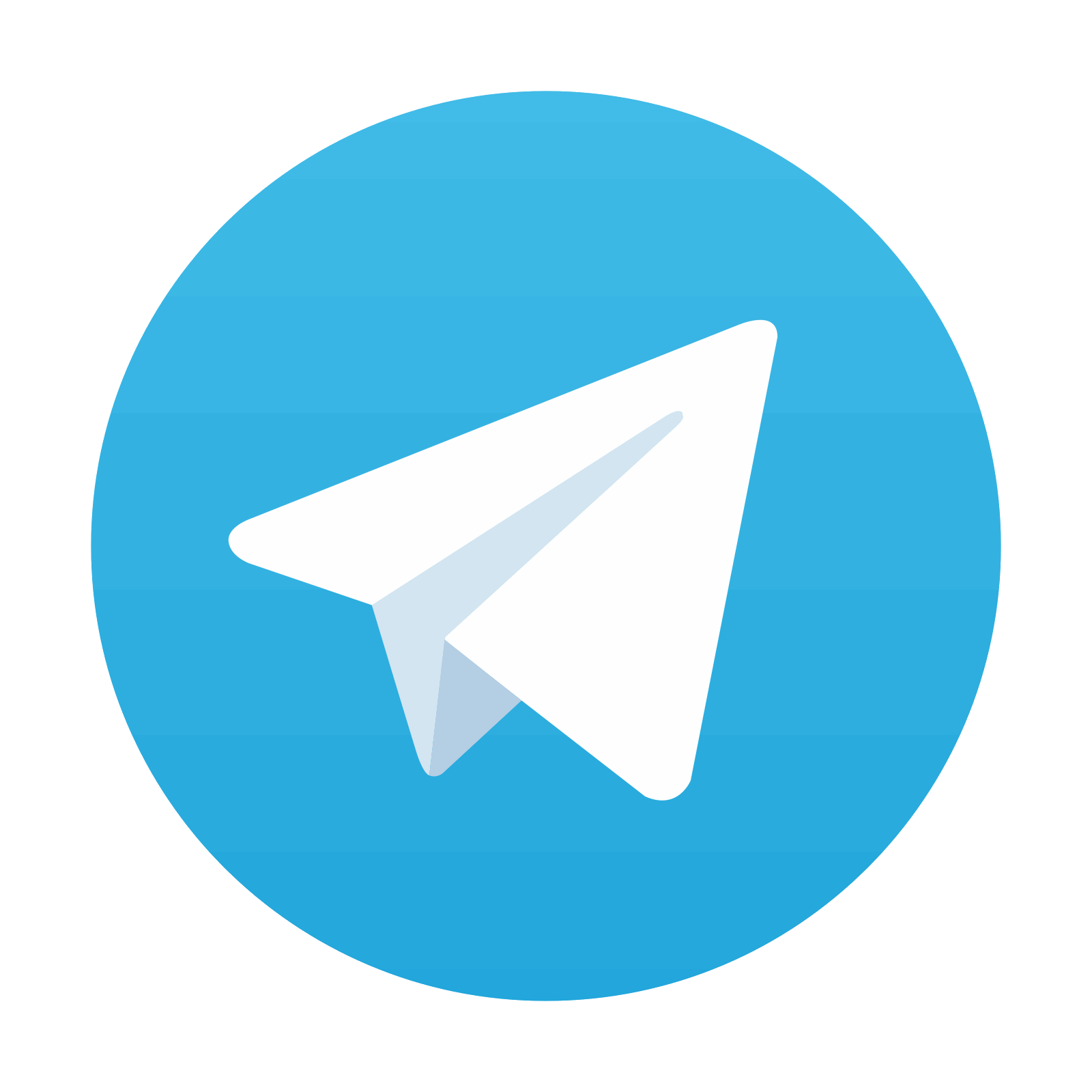
Stay updated, free articles. Join our Telegram channel

Full access? Get Clinical Tree
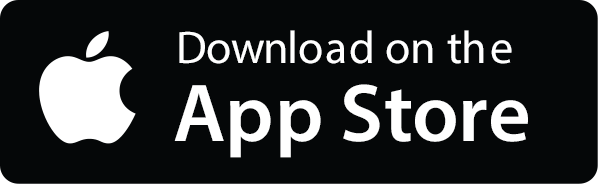
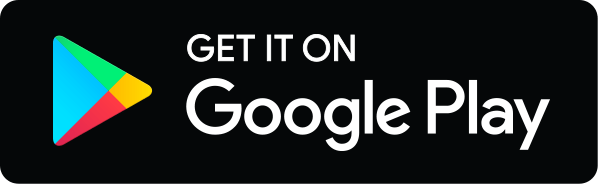