Fig. 1
RRI is measured by Doppler sonography in an intrarenal artery, as the difference between the peak systolic (PS) and end-diastolic (ED) blood velocities divided by the peak systolic velocity (PSV)

The morphology of Doppler spectrum of RRI is mainly determined by the velocity/time (V/t) curve that is assayed along the main renal artery, that can be defined as a “low resistance” curve. The systolic phase quickly increases to peak velocity and is followed by a progressive and gradual deceleration phase with a telediastolic velocity that does not decrease below 30–40 cm/sec. This Doppler spectrum is common and peculiar to all parenchymal flows, because a sufficient oxygen supply must be assured throughout the cardiac cycle (Meola et al. 2016b).
RRI was introduced in 1950 and initially proposed for the semi-quantitative assay of intra-renal vascular resistance by Pourcelot in (1974). He showed that the ratio was influenced by changes in vascular resistance distally to the point of RRI assay. The term RRI has been kept to the present time, even if the strict relationship between RRI and actual renal vascular resistance has become very weak (Chirinos and Townsend 2014; O’Neill 2014).
According to these findings RRI was initially used for the diagnosis and follow-up of acute and chronic renal disease (Radermacher et al. 2002; Viazzi et al. 2014) which are associated to dynamic and/or structural changes in intra-renal vessels. Later on RRI was proved to be a strong independent predictor of renal failure (Radermacher et al. 2002; Sugiura 2011). However, in the meantime growing evidence showed that RRI is the result of many intra and extra-renal determinants and that renal vascular resistance is only one of these, and not the most important (Boddi et al. 2015) (Fig. 2). Remarkably, in 1991 Gosling et al. (1991), and in 1999 Bude and Rubin (1999), clearly showed by in vitro experiments performed in simple artificial circuits, that RRI is dependent on both renal vascular compliance and resistance, becoming less dependent on resistance as compliance decreases. When compliance is zero, RRI is independent of changes in renal vascular resistance. Moreover, new experimental (Chirinos and Townsend 2014; Tublin et al. 2003) and clinical data (Chirinos and Townsend 2014; O’Neill 2014) were obtained showing that RRI was markedly affected by the changes in renal (renal interstitial and venous pressure) and systemic (pulse pressure) determinants of vascular compliance, and only scarcely by the chronic increase in renal vascular resistance. In chronic renal diseases and in transplant recipients, RRI mainly depends on systemic vascular compliance assayed as pulse pressure, rather than renal vascular properties (Chirinos and Townsend 2014; O’Neill 2014). In these patients the increase in RRI is strictly associated with the decrease in systemic vascular compliance assayed as pulse pressure that is negatively modulated by aging and traditional cardiovascular (CV) risk factors, among which hypertension plays a major role (Chirinos and Townsend 2014). According to this point of view, in hypertensive patients with and without renal function impairment, the increase in RRI predicts worse renal and general outcomes, as a marker of systemic atherosclerotic burden rather than of local renal damage. However, this statement is still a matter of debate and do not weaken the clinical relevance of RRI measurement for risk scoring.


Fig. 2
Different renal and extrarenal systemic determinants concur to determine RRI. (1a) and (2a): renal and systemic determinants that decrease RRI. (b): glomerular resistance scarcely or not affect RRI. (1c) and (2c): renal and systemic determinants that increase RRI (Adapted by Boddi et al. 2015)
On the contrary full agreement was reached on the clinical use of RRI as a specific marker of renal damage, in subjects affected by those renal pathologies that can promote the progression of renal damage in hypertensive patients, i.e. hydronephrosis, renal vein thrombosis, increased abdominal pressure and acute kidney injury. In all these conditions the acute and marked increase in renal tubule-interstitial and venous pressure due to hydrostatic or inflammatory edema leads to the direct increase in RRI values (Boddi et al. 2015).
3 RRI Threshold in Clinical Practice
Aging is associated with a progressive quantitative decrease of renal microvascular bed and with an increased thickness of tunica media of renal arterioles (Fig. 2). This determines a decrease in the lumen/vessel wall ratio. Both these changes result in a progressive increase in RRI (Boddi et al. 1996) that can be amplified by the contemporaneous increase in systemic arterial stiffness. The steep age-dependent rise in RRI values is specific to the renal vasculature and is not seen in other vascular beds. The age-related hypertrophic remodelling of the vessel wall of renal microvessels can be further amplified by hypertension and/or diabetes (Pontremoli et al. 1999a; Maestripieri et al. 2012). In healthy adults most authors use >0.70 as the cut-off limit for pathological RRI and do not establish normal values according to age. However, in healthy subjects >70 year, RRI >0.70 can be measured in the absence of renal diseases, whereas in subjects aged >40 year can be the first marker of renal damage that anticipates GRF reduction and the occurrence of albuminuria (Boddi et al. 1996). Further an emerging clinical issue is the evaluation of the actual renal function in elderly normal patients or diabetics, since the estimated values of GFR by math formula suffer of limits in these subgroups. So that, in elderly subjects with normal renal function or diabetics, RRI assessment might be considered an other non-invasive way to reveal early renal damage.
In a recent large multicentric family-based population study, age was confirmed as a determinant of RRI. Ponte et al. (2014) also showed that the relationship of RRI with age is nonlinear and that RRI increases sharply after the age of 40.
In the same multicentric study female sex was associated with higher RRI values due to hormone differences and the fact that RRI has a genetic tract was reported (Ponte et al. 2014); the clinical relevance of these findings must be investigated by dedicated studies.
In newborns and in children under the age of four, RRI > 0.70 can be found because of renal anatomical structure in this period and is not associated with renal pathologies (Bude et al. 1992).
4 Systemic and Renal Determinants of RRI
In any arterial vascular tract, Doppler waveform is the integrated result of what happens before and downstream from the point where the flow is assayed.
4.1 Systemic and Extrarenal Determinants
4.1.1 Stiffness-Related Systemic Pulse Pressure
The ratio of systolic to diastolic blood pressure (see RRI equation) is an inverse function of pulse pressure. Thus, for any given intra-renal vascular resistance an increase in systemic systolic arterial pressure promotes a higher peak renal velocity and/or a decrease in diastolic arterial pressure. That results in a lower end-diastolic velocity. As a direct consequence, in vivo any increase in systemic arterial stiffness that causes increased pulse pressure is associated with high RRI values, both in physiological (aging) and pathological (hypertension) conditions (Fig. 2c). Changes in pulse pressure can also be tonic or phasic, as during an infusion of L-NG-monomethyl argirine (L-NMMA), an inhibitor of endothelial NOS. Neither RRI under baseline conditions nor RRI during L-NMMA infusion were related with renal vascular resistance or renal perfusion, assayed by para-aminohippuric acid and insulin clearance (Raff et al. 2010). On the contrary, RRI changed according to variations of central pulse pressure.
The relationship between RRI and pulse pressure has also been investigated in recipients of kidney transplants where systemic pulse pressure is recipient-specific, whereas the compliance of interlobular arteries is donor specific; in these kidneys RRI correlated with the age of the recipient but not of the donor, with recipient pulse pressure but not parameters of allograft function and with RRI of other (i.e. splenic) districts of the recipient (Naesens et al. 2013). As a whole the findings observed in transplant recipients strongly support that RRI primarily reflects the properties of the systemic vasculature that can hidden or weaken the effects of local renal damage on intrarenal vasculature.
4.1.2 Stenosis-Related Pulse Pressure
Severe (>80 %) renal artery thoracic or sovra-renal abdominal aorta or valvular aortic stenosis all decrease pulse pressure in vascular districts distal to stenosis, and decrease RRI values (<0.60) as a result of low peak systolic velocity (Fig. 2, 2a). The dampened flow is revealed by the peculiar Doppler wave pattern characterized by a “tardus”, slow, and “parvus”, small pulsus (Figs. 2 and 3). The finding of low RRI in the homolateral kidney and the lateralization of RRI (delta > 0.05) is indirect but reliable proof of severe renal artery stenosis (Fig. 4). In fact the gradual reduction of renal perfusion pressure up to 40 % does not substantially change renal blood flow and glomerular filtration rate, thanks to the self-regulating mechanisms of intrarenal circulation. In these conditions RRI is not affected. This mechanism becomes ineffective when morphological renal arterial stenosis is >75 %, renal perfusion pressure falls >40 % and renal systolic pressure is <70–80 mm Hg (Textor and Wilcox 2001; Jacobson 1988). This renal stenosis is defined hemodynamically significant, because it activates the renal renin angiotensin system (Meola et al. 2016b; Butterly and Schwab 2000) and demodulates Doppler waveform at intrarenal arteries. However, when distal renal vascular disease coexists due to chronic ischemic kidney, the hemodynamic effects of renal artery stenosis may be hidden. In these patients RRI is symmetrically high, not lateralized and the hemodynamic effect of arterial stenosis on renal parenchyma cannot be evaluated by Doppler ultrasound (Meola et al. 2016b; Boddi et al. 2015) (Fig. 4) (see also Ultrasound diagnostics of renal artery stenosis, page 14).



Fig. 3
Schematic representation of possible RRI changes. From the left to the right:(a) low RRI values (0.50) because of low peak systolic velocity (PSV) with peculiar Doppler wave pattern of post-stenotic flow characterized by a “tardus”, slow, and “parvus”, little pulsus; (b) normal Doppler wave pattern and PSV/EDV at interlobar arteries; (c, d) high RRI (0.75–0.90) due to high peak systolic (PSV) and decreased end-diastolic velocity (EDV) (Adapted by Boddi et al. 2015)

Fig. 4
Schematic representation of Doppler flow patterns assayed at and distal to a hemodynamically arterial renal stenosis; RRI is lateralized (delta > 0.05); when vascular or parenchimal nephropathies coexist, RRI values symmetrically increase and the hemodinamic effect of renal artery stenosis is hidden (Adapted from Boddi et al. 2015)
4.1.3 Heart Rate
Changes in heart rate can affect RRI independently from the other hemodynamic parameters because of changes in diastolic duration that modulate end-diastolic velocity. During bradicardia diastolic duration increases and high RRI is measured. On the contrary during tachicardia diastolic duration shortens and RRI decreases (Fig. 2, 2a and c).
4.2 Renal Determinants
4.2.1 Renal Interstitial and Venous Pressure
The renal capillary wedge pressure (interstitial tissue plus venous pressure) is a major renal determinant of RRI. In ex vivo rabbit kidney model elevations in ureteral pressure were significantly correlated with increased RRI values, mean renal vascular resistance (pressure/flow) and decreased mean conductance (flow/pressure) (Tublin et al. 1999). In humans in vivo the acute increase of renal interstitial pressure by hydronephrosis or of venous pressure by venous thrombosis, or of both by abdominal hypertension, results in a linearly related increase in RRI (Fig. 2, 1c). Also renal hematoma can acutely increase the pressure of interstitial compartment and elevate RRI (Platt et al. 1989).
Most importantly, acute kidney injury (AKI) is associated with an acute increase in interstitial pressure because of sustained vasoconstriction and ischemic and inflammatory damage of the tubulo-interstial compartment by sustained hypoperfusion. In all these clinical conditions the occurrence, severity and progression of renal damage can be well monitored by changes in RRI values (Platt et al. 1989; Schnell et al. 2012; Dewitte 2013; Le Dorze et al. 2012; Darmon et al. 2011). Recently, in critical patients admitted for medical, surgical or trauma disease, high RRI values at admission were significantly and independently associated with in-ICU mortality and persistent AKI at ICU discharge (Boddi et al. 2016).
4.2.2 Histological Renal Parameters – RRI and the Tubulo-Interstitial Compartment
Twenty years ago Platt et al. showed that RRI was significantly higher in nephropathies with tubulo-interstitial and/or vascular injury than in isolated glomerulopathies (Platt et al. 1990). Glomerular arterial resistance, that accounts for about 20 % of total renal vascular resistance, scarcely concurs to the determination of RRI; and nephropathies characterized by prevalent glomerular involvement are not associated with increased RRI. RRI is not a marker of renal function (Fig. 2).
The studies on the relationship between tubular, interstitial and arterial damage and RRI in renal disease and in kidney transplants show conflicting results: according to Ikee et al., only arteriolosclerosis out of all histological parameters independently correlated with RRI in chronic renal disease (Ikee et al. 2005), whereas in renal transplants investigated at 3, 12 and 24 months after transplantation RRI was not associated with any renal allograft histological features. On the contrary, other Authors reported that high RRI values were related to more severe tubulo-interstitial damage score, and an association between RRI values and the extension of interstitial fibrosis was shown, probably due to the rise in pressure exerted by interstitial fibrosis on adjacent vessels. Remarkably, interstitial fibrosis closely correlated to renal function and long-term prognosis and could underline the role of RRI as an independent marker of renal and clinical outcome in patients with CKD (Sugiura 2011; Bigè et al. 2012).
The possible use of RRI as a marker of tubulo-interstitial nephropathy is supported by the findings that the detection of high RRI values allowed the early identification of both normotensive and hypertensive patients with chronic tubulo-interstitial nephropathy diagnosed by 99 mTc DMSA scintigraphy and signs of tubular dysfunction, when renal function was still preserved (Boddi et al. 2006). Moveover, in hypertensive patients with normal creatinine clearance and no albuminuria, high RRI values were associated with low grade inflammation (Protein C reactive >2 mg/dl) and hyperuricemia (>6.5 mg/dl) (Berni et al. 2012, 2010). Both sustain a tubulo-interstitial nephropathy. In hypertensive patients, serum uric acid strongly correlated with RRI, independently of renal function or albuminuria, but the altered intrarenal hemodynamics did not explain the pathophysiology of hyperuricemic renal damage (Geraci et al. 2016).
A generalized consensus was reached that tubulo-interstitial and not glomerular nephropathies affect RRI and that RRI does not measure renal function.
4.2.3 Role of Arterial Vascular Resistance
Based on early experimental animal data (Bude and Rubin 1999; Tublin et al. 1999), RRI was long considered to directly mirror intrarenal resistance, thus allowing a non-invasive glimpse into intrarenal (patho) physiology (Norris and Barnes 1984). Under physiological conditions RRI assay could detect phasic increase in renal vascular resistance induced by sympathetic activation obtained by cold pressor test or handgrip; in the same subjects the increase of blood volume by acute hydration resulted in an RRI decrease (Boddi et al. 1996). Repeated daily sessions of music-guided slow-breathing increased parasympathetic modulation and decreased RRI early in the study.These changes were being followed by a positive modulation of baroflex sensitivity and decrease in blood pressure (Modesti et al. 2015). In patients with heart failure high RRI values were associated with increased intrarenal vascular resistance due to neurohormonal hyperactivity and independently predicted heart failure progression (Ciccone et al. 2014). In septic shock Doppler ultrasonography and RRI measurements may help determine in each patient the optimal mean aortic pressure for renal blood flow and may be a relevant end-point to titrate the haemodynamic treatment by fluid and norepinephrine administration (Deruddre et al. 2007).Catheter-based renal sympathetic denervation in patients with resistant hypertension reduced RRI probably through a decrease in intraparenchymal resistance, not mediated by reduction in systolic blood pressure (Mahfoud et al. 2012). As a whole these findings sustain that the RRI can detect phasic changes in renal vascular resistance.
On the contrary, RRI changes during dynamic vasodilation caused by nytroglicerin or (L-NMMA) infusion were poorly associated with the concurring direct measurement of renal resistance by scintigraphy, even if the changes in RRI and in renal vascular resistance moved in the same direction. Rather, RRI changes were directly related to changes in pulse pressure (Raff et al. 2010). Increased RRI has been shown to correlate with systemic arterial stiffness measured by ambulatory blood pressure derived by Ambulatory Arterial Stiffness Index (Ratto et al. 2006). Moreover, a close relationship between RRI and other markers of systemic atherosclerotic burden, as intima-media thickness and ankle brachial index, was shown in hypertensive patients with chronic renal disease, independently of renal damage (Pontremoli et al. 1999b).
For many years the role of high RRI values as an independent marker of renal outcome in patients with CKD was mainly due to the assumption that RRI increase was determined above all by the progressive “tonic” increase in vascular resistance because of: (a) decrease in arterial compliance due to renal arteriosclerosis; (b) elevation of extra-vascular renal pressure exerted by interstitial fibrosis in adjacent vessels; (c) vasoconstriction secondary to hypoxia and to loss of capillaries associated with renal fibrosis. All these are associated with decline in renal function (Boddi et al. 2015).
In recent years evidence has been gathered around RRI being an independent marker of renal and cardiovascular outcomes, because it measures systemic and not renal hemodynamic parameters, and reflects systemic vascular disease (Granata et al. 2014). We agree with O Neill’s title “Renal resistive index. A case of mistaken identity” (O’Neill 2014). However, there is no doubt that both phasic (sympathetic activation) and tonic (arteriolosclerotic ) changes in renal arterial resistance can modulate RRI.
4.2.4 RRI and Subclinical Renal Damage in Hypertension
In clinical practice albuminuria is measured to define subclinical renal damage in hypertensive patients, and the combination of eGFR and albuminuria is a useful predictor of CV disease (Viazzi et al. 2014). In recent years RRI was also validated as a clinical marker of subclinical renal damage as well as a prognostic predictor of renal and CV outcomes to use in addition to the above mentioned markers in order to improve their performance.
In untreated patients with primary hypertension and normal renal function, high RRI (>0.70) highlights subclinical signs of renal damage and shows a direct relationship with the amount of urine albumin excretion (Miyoshi et al. 2016). Further RRI was proved to be a useful index to predict increase in urinary albumin excretion in patients with essential hypertension (Viazzi et al. 2014). With the progression of hypertensive renal damage, high RRI values are often associated with a mild reduction in glomerular filtration rate and increased albuminuria or both (Doi et al. 2012). In hypertensive patients high (>0.70) RRI predicts renal dysfunction evaluated at 12 months by Cystatin C determination (Okura et al. 2010). Evaluation of both eGFR and RRI instead of albuminuria could be another investigative option to identify essential hypertensive subjects without clinical evidence of renal damage and cardiovascular disease, predisposed to worse renal and CV outcomes.
In hypertensive patients undergoing chronic antihypertensive therapy with no microalbuminuria and normal renal function, higher RRI values were found in those with hyperuricemia or low grade inflammation (PCR >2 mg/dl), both associated with tubulo-interstitial inflammation and endothelial dysfunction (Berni et al. 2012). Remarkably, in experimental studies it was found that hyperuricemia causes glomerular hypertension, vasoconstriction and ischemia, a potent stimulus for tubulo-interstitial inflammation and fibrosis (Berni et al. 2010; Sanchez-Losada et al. 2005).
Dynamic evaluation of RRI in normoalbuminuric patients with newly diagnosed hypertension showed that the decrease in RRI induced by nytroglicerine was lower in hypertensives than in controls despite similar baseline RRI (Bruno et al. 2011). Reduced renal vasodilation was independently related to the increase of systemic arterial stiffness and suggests a role of systemic hemodynamic load in determining early renal microvascular alteration in hypertension. RRI determination could help to understand the intricate link between hypertension and subclinical renal damage, till now mainly supported by the relationship between hypertension and microalbuminuria. The unifying mechanism that accounts for the different roles of RRI as a marker of subclinical renal damage and a prognostic predictor of renal and cardiovascular outcomes was suggested by Hashimoto et al. (Hashimoto and Hito 2011) who recorded aortic pressures, aortic and peripheral pulse wave velocities and RRI in 133 hypertensive patients: (a) RRI depends strongly on aortic pulse pressure and aortic stiffness; (b) RRI correlates inversely with the femoral reverse-flow and diastolic forward-flow indices; and (c) RRI predicts urinary albumin excretion together with the aortic pulse pressure. In these hypertensive patients the altered renal hemodynamics due to increased central pulse pressure and aortic stiffness contributed to the development of renal microvascular damage marked by high RRI. Every 0.1 increase in renal RRI was associated with a 5.4-fold increase in the adjusted relative risk of albuminuria (Hashimoto and Hito 2011). According to these findings atherosclerosis increases systemic arterial stiffness, predisposes renal circulation to a greater hemodynamic load (pulse pressure) and results in higher renal microvascular resistance. Increased systemic arterial stiffness underlines the strict relationship between RRI and atherosclerotic damage such as left ventricular hypertrophy, carotid intima media thickness and ankle brachial index (Pontremoli et al. 1999a; Calabia et al. 2014; Geraci et al. 2015). On the other hand high RRI might contribute to systemic arterial stiffening by renal dysfunction and activate a self-perpetuating process. Moreover, RRI proved to be an independent predictor of worse renal and CV outcomes in 426 patients with primary hypertension and no previous CV disease followed for a mean of 3.1 years (Doi et al. 2013). We can conclude that in hypertensive patients with normal renal function RRI is an early clinical marker of subclinical renal damage, that can anticipate the occurrence of microalbuminuria, but also signals systemic atherosclerotic burden. For both reasons high RRI is a good predictor of worse renal and cardiovascular outcomes.
The vast majority of RRI measurements reported in literature are carried out in hypertensives on different pharmacological combinations without a wash-out period; this could result in confounding factors for the study of determinants of RRI.Remarkably, scarce data are available in literature (Leoncini et al. 2002; Watanabe et al. 2006) about the effect of pharmacological therapy on RRI values; whether and how the decrease in RRI values could result in an improvement of renal damage and in renal and CV outcomes is unknown. This fact is mainly responsible for the limited use of RRI in clinical practice and need dedicated studi.
4.2.5 RRI and Renal Damage in Diabetes
RRI can detect early renal damage in patients with diabetes type 1 and 2: when renal function is normal and albuminuria is absent; increased RRI predicts the occurrence of albuminuria (Hamano et al. 2008; Nosadini et al. 2006). Most importantly, in patients without microalbuminuria RRI values >0.70 independently predicted the occurrence of diabetic nephropathy. In diabetic subjects with albuminuria and reduced creatinine clearance, RRI >0.80 predicts a worse renal outcome (Boddi et al. 2015).
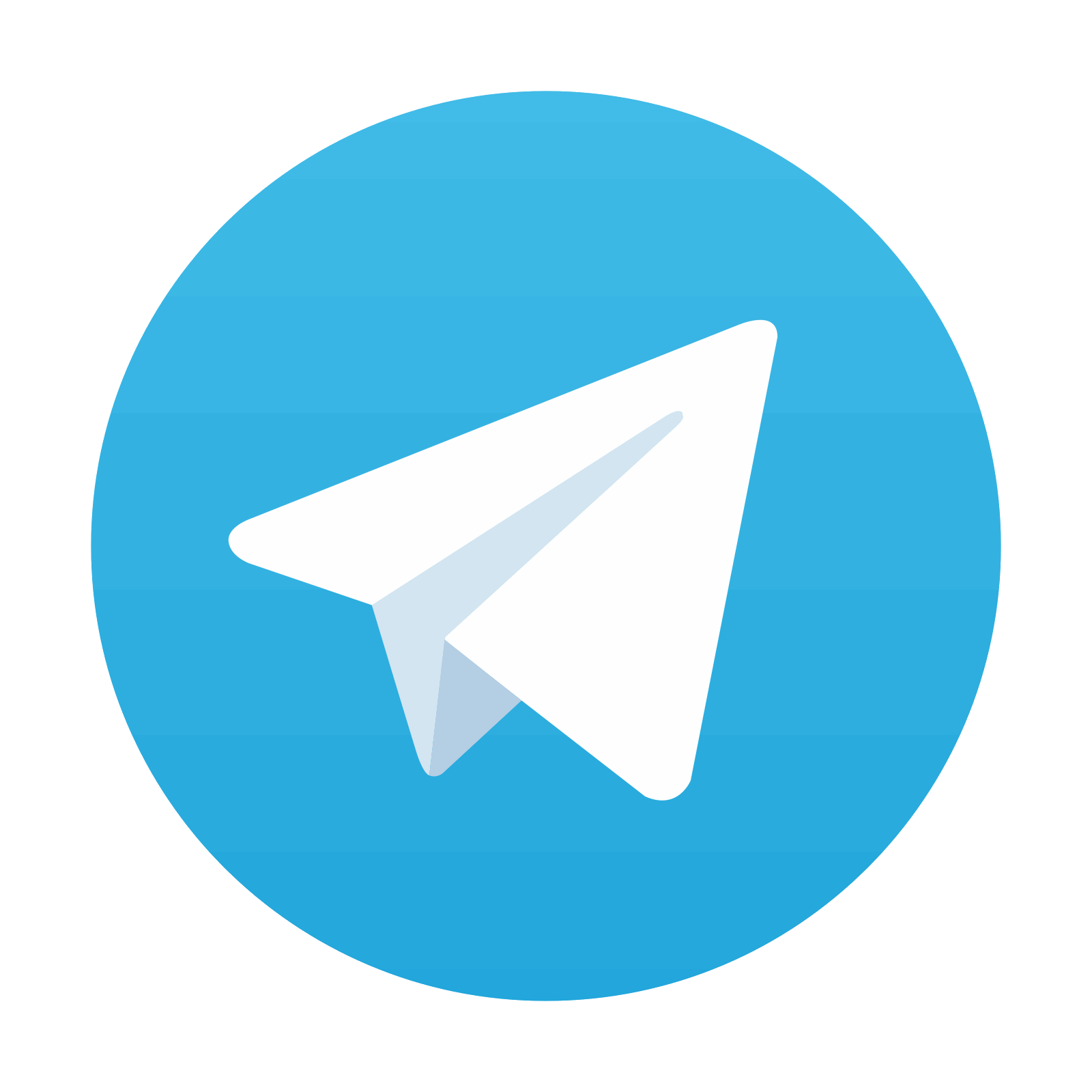
Stay updated, free articles. Join our Telegram channel

Full access? Get Clinical Tree
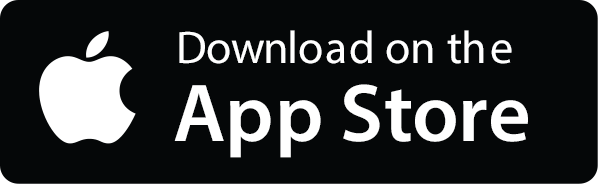
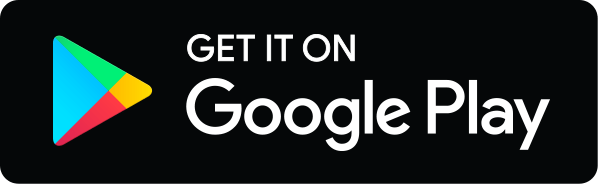