Abstract
Tricuspid atresia (TA) is a type of cyanotic congenital heart defect (CCHD) characterized by complete obstruction of the atrioventricular valve associated with the morphologic right ventricle. It is an archetypal lesion representing single-ventricle physiology. It is the third most common CCHD. The definition, embryology, presentation, diagnostic assessment, and management of tricuspid atresia are discussed. This lesion represents a gamut of variations in anatomy and physiology requiring the cardiac team to palliate these patients using many different techniques and strategies to obtain the goal of a total cavopulmonary connection. Because the systemic ventricle is the morphologic left ventricle, patients with TA tend to have a good prognosis when compared with other single-ventricle lesions.
Key Words
Tricuspid atresia, Fontan, bidirectional Glenn, Blalock-Taussig shunt, hemi-Fontan, fenestrated Fontan, lateral tunnel Fontan
Definition
Tricuspid atresia (TA) is a type of cyanotic congenital heart defect (CCHD) characterized by complete obstruction of the atrioventricular (AV) valve associated with the morphologic right ventricle (RV). The floor of the right atrium is formed by muscular tissue or an imperforate vestigial remnant of the right AV valve. It is an archetypal lesion representing single-ventricle physiology.
Epidemiology
It is estimated that one in 10,000 babies born in the United States will have a diagnosis of TA. It is the third most common CCHD, after transposition of the great arteries and tetralogy of Fallot. TA represents 1.6% and 2% of all diagnoses in neonates and infants, respectively, in the Society of Thoracic Surgeons Congenital Heart Surgery Database-2016. There is a male preponderance with this defect when associated with transposition of the great vessels. Without any intervention the lesion is associated with a 90% mortality by the end of the first year of life.
Embryology
The true embryologic basis for TA remains elusive, as is the case with many congenital heart defects. It appears to be related to a result of malalignment of the ventricular septum in relation to the atria and endocardial cushions. The ultimate size of the bulbus cordis (RV) is determined by the extent to which the septum is shifted to the right and the size of the bulboventricular foramen (BVF). The series of abnormalities described earlier appears to occur during days 25 and 55 post conception. The incidence of TA also appears to increase with maternal febrile illness. Animal studies in mice associate ZFPM2 / FOG2 and HEY2 genes in the pathogenesis of TA.
Anatomy and Classification
The following are the anatomic characteristics of TA: (1) lack of communication between the right atrium (RA) and the morphologic RV, (2) the presence of an interatrial communication, (3) an enlarged left-sided AV valve, and (4) total absence of the inlet and varying degrees of deficiency of the trabecular portions of the RV (referred to as the infundibular chamber ) because a “true” ventricle needs to have an inlet. A communication (present 90% of the time) between the infundibular chamber and the left ventricle (LV) known as a bulboventricular foramen (BVF), commonly referred to as a ventricular septal defect (VSD). Twenty percent of patients with TA have other associated cardiac anomalies (such as transposition of the great vessels, coarctation of the aorta, aortic arch hypoplasia).
Kuhne (1906) proposed a classification system for TA based on the interrelation of the great arteries. This has been further modified by Edwards and Burchell and Rao ( Figs. 65.1 and 65.2 ). The principal groups based on the ventriculoarterial relations are depicted in Box 65.1 .


Type 1: Normally related great vessels
Type 2: d-Transposition of the great arteries
Type 3: l-Transposition of the great arteries
Each type is further subdivided based on the extent of restriction to pulmonary blood flow.
Subtype A: Pulmonary atresia
Subtype B: Pulmonary stenosis
Subtype C: No obstruction
A commonly associated anomaly with type 1 is a left superior vena cava (left SVC). Types 2 and 3 can be associated with varying degrees of obstruction at the level of the BVF and aortic arch.
Five variants of tricuspid valve morphology are found in TA. The most common (76% to 84%) is muscular atresia. Dimpling of the muscular floor may be seen. The membranous form accounts for 4% to 12%. A valvar form (6%) is identified by a thin, imperforate membrane of tissue consisting of minute fused-valve leaflets. This form may be associated with rudimentary chordae. The Ebstein form (4% to 6%) and AV canal type (2%) account for the other types.
Pathophysiology
Prenatal Circulation
Despite the clinically significant alterations in fetal circulation inherent in TA, these do not seem to be detrimental to fetal somatic development. The systemic venous return is forced across the foramen ovale into the left heart. As a consequence, the usual PO 2 differential present in a normally developing fetus is not present. The lowered PO 2 to the heart and brain and the elevated PO 2 to the lungs do not seem to produce any clinically significant anomalies. Almost all of the left ventricular output is ejected through the aorta in a fetus with type 1 defect; as a result the aorta is big and is rarely associated with arch obstruction. This is in contradistinction to type 2 defects, which predictably are associated with a higher prevalence of arch obstruction (30% to 50%).
Postnatal Circulation
An unrestrictive atrial septal defect (ASD) is crucial to allow mixing of the systemic and pulmonary venous blood and maintenance of cardiac output because there is a lack of communication between the RA and the RV. The pathophysiology of TA is dictated by the amount of pulmonary blood flow, which is determined by the anatomic obstruction to pulmonary blood flow (size of the BVF and degree of pulmonary stenosis [PS]), patency of the ductus arteriosus, and pulmonary vascular resistance (PVR). Patients may present with decreased pulmonary flow, increased pulmonary blood flow, or in the rare instance with a balanced circulation.
For patients with TA and normally related great vessels, blood from the LV enters the RV across the BVF to provide pulmonary blood flow. For those with concomitant PS or restriction at the BVF the pulmonary blood flow is provided via a patent ductus arteriosus (PDA). Patients may present with cyanosis due to reduced pulmonary blood flow or pulmonary overcirculation in the event that the BVF is nonrestrictive and there is not important PS. Cyanosis may worsen from progressive PS, narrowing of the BVF (there is a tendency for the BVF to decrease in size over the first 2 to 3 years of age), or closure of the PDA.
For patients with TA and transposed great vessels, systemic circulation is dependent on the size of the BVF, and the blood from the LV must traverse the BVF to reach the systemic great vessel. Systemic blood flow may become ductal dependent if there is severe subaortic stenosis, usually at the level of the BVF. Most patients present with pulmonary overcirculation, especially in cases in which there is a degree of obstruction to systemic blood flow (either at the level of the aortic arch or at the subaortic region). Patients with l-transposition of the great vessels (l-TGA) have a higher incidence of subaortic stenosis.
Systemic arterial desaturation is present in all patients with TA secondary to an obligatory admixture of systemic, coronary, and pulmonary venous blood in the left atrium (LA). The degree of desaturation depends on the pulmonary blood flow. A pulmonary-to-systemic blood flow ratio (Q p :Q s ) ratio of 1.5 to 2.5 : 1 usually results in adequate saturations, and higher shunt fractions will result in left ventricular volume loading, pulmonary overcirculation, and heart failure. Normal systemic ventricular function is one critical component of successful single-ventricle palliation, which eventuates in the Fontan operation.
Obstruction at the level of the interatrial communication is rare. This is evident when there is a pressure gradient of more than 3 mm of Hg across the ASD or giant a waves. Spontaneous closure of the BVF has an estimated prevalence of 38% to 48%. The mechanism involved is usually progressive muscular encroachment and fibrosis from endocardial proliferation. Echocardiographic studies have shown that a cross-sectional area of the BVF of less than 2 cm 2 /m 2 may predict later restriction at the BVF or even indicate prescient obstruction.
Diagnostic Assessment
Chest x-ray examination may demonstrate oligemic or plethoric lung fields based on the amount of pulmonary blood flow. Echocardiography provides definitive diagnosis ( Fig. 65.3 ). The “tricuspid atresia” is seen as a hyperechoic shelf. Varying degrees of RV hypoplasia are seen. Attention must be directed to the relationship of the great arteries, arch obstruction, restrictiveness of the ASD and BVF, presence of and degree of PS, presence and size of the ductus, and identification of other associated anomalies. Electrocardiogram demonstrates RA enlargement, LV hypertrophy, and left axis deviation. The P wave duration is increased with a short PR segment. ST depression may be seen in the lateral leads, especially in patients with TGA ( Fig. 65.4 ).


Cardiac catheterization is indicated for therapeutic interventions, such as enlargement of a restrictive ASD or ductal stenting, which is becoming more common in lieu of aortopulmonary shunts. Pre-Glenn and pre-Fontan cardiac catheterizations are common. In patients undergoing a cavopulmonary shunt, the study provides important hemodynamic and anatomic details. These include the PVR, distortion of the pulmonary arteries, any arch obstruction if intervention on the arch was performed initially, and the location and size of the SVC. Pre-Fontan studies are aimed at obtaining hemodynamic data, including pulmonary artery (PA) pressure, transpulmonary gradient, PVR, left ventricular end-diastolic pressure (LVEDP), as well as anatomic data, including the presence of important PA stenosis, PA and bidirectional cavopulmonary shunt anatomy, presence and location of aortopulmonary and venovenous collaterals, and intervention on these collaterals as indicated. Recently magnetic resonance imaging (MRI) has been gaining popularity as a modality of anatomic and hemodynamic assessment before the Glenn or Fontan operation. The lack of exposure to radiation and the noninvasive nature of the study are very appealing. Cardiac catheterization is reserved for patients in whom (1) noninvasive tests indicate the need for intervention, (2) ventricular function or hemodynamics appear poor, and (3) MRI is contraindicated (implants, pacemakers).
Management of Tricuspid Atresia
TA was the first lesion successfully managed as a “single ventricle” and in fact was the lesion that was initially described in the so-called perfect Fontan. The treatment remains mainly surgical. The ultimate goal of the single-ventricle pathway is through a series of operations to convert parallel circulation of the systemic and pulmonary beds into an in-series circulation. The pulmonary circulation remains passive, whereas the single ventricle maintains systemic circulation. The transpulmonary gradient remains the driving force for the blood from the systemic venous side to the pumping ventricle. The classic stages include (1) providing a stable source of pulmonary blood flow with either an aortopulmonary shunt or ductal stent; (2) constructing passive flow of the upper-body venous blood to the pulmonary arteries with either a bidirectional cavopulmonary shunt (Glenn) or a hemi-Fontan; and (3) Fontan completion, which entails separation of the circulations by creating a pathway for the lower-body venous blood (inferior vena cava [IVC] and/or hepatic veins) to enter the passive pulmonary circulation.
Management of the Newborn With Decreased Pulmonary Blood Flow
The subgroups of neonates with decreased pulmonary blood flow ( Fig. 65.5 ) require some form of a shunt to increase pulmonary blood flow. These patients are dependent on the PDA for their pulmonary blood flow. Ductal closure would lead to severe hypoxemia, metabolic acidosis, and death, therefore necessitating continuous infusion of prostaglandin E 1 (PGE 1 ) to maintain ductal patency. Pulmonary overcirculation is a condition in which there is increased blood flow to the lungs compromising systemic perfusion and adequate tissue oxygen delivery. Arterial oxygen saturation, as measured by pulse oximetry, is surrogate to the Q p :Q s (shunt fraction). For example, an oxygen saturation of 75% approximates a Q p :Q s of 1 : 1, 85% of approximately 2 : 1, and 95% of 5 : 1. The serum lactate levels are a good indicator of the systemic cardiac output and systemic oxygen delivery. Lactate levels are elevated (>2) when the tissue perfusion is inadequate. Lactate levels are not a reflection of Q p :Q s except that inadequate Q p might result in significant hypoxemia, leading to inadequate tissue oxygen delivery, and excessive Q p (in relation to Q s ) might correlate with inadequate Q s (as more output is shunted toward the pulmonary circulation) and inability to sustain necessary systemic cardiac output. A Q p :Q s of 1 : 1 requires a “double” cardiac output from the single ventricle (one output for the pulmonary circulation and one for the systemic circulation), and a Q p :Q s of 3 : 1 requires a quadruple cardiac output! So it is understandable how the demands of increasing cardiac work from unlimited pulmonary blood flow can overwhelm the capability of the heart to provide essential systemic flow. Optimal Q p :Q s is obtained by medical management with adjustments in pulmonary and systemic vascular resistance. This includes correction of acidosis, maintenance of normal blood glucose and calcium levels, normothermia, and manipulation of the blood PO 2 and CO 2 levels. Mechanical ventilation and sedation may be required to maintain physiologic Q p :Q s . The systemic vascular resistance can be altered by using vasodilators and vasoconstrictors. It is critical to realize that the Q p :Q s is a ratio and not an absolute number for cardiac output. Lactic acidosis is an excellent parameter to reflect whether or not systemic oxygen delivery is adequate in the face of the Q p :Q s presented by the patient. A patient with a systemic oxygen saturation of 95% and normal lactate levels may have a high Q p :Q s but is clearly maintaining adequate systemic oxygen delivery (e.g., has a normal cardiac output). A patient with an oxygen saturation of 80% (Q p :Q s 1.5 : 1) and a lactate level that is at 3 and rising may have less overall cardiac work but is also showing signs of inadequate cardiac output.

As mentioned previously, the first priority for management is to ensure a stable source of pulmonary blood flow. Flow generated across a BVF may be adequate initially, but the proclivity of the BVF to close mandates close follow-up of oxygen saturations and echocardiograms with willingness to place a shunt if the flow into the pulmonary arteries across the BVF becomes inadequate. If the baby is ductal dependent for pulmonary blood flow (common), then the initial management might require choosing between a ductal stent or a surgically placed aortopulmonary shunt.
The Modified Blalock-Taussig Shunt
Creating an aortopulmonary shunt is used to provide a stable source of pulmonary blood flow. The classic Blalock-Taussig shunt (which entailed division of the subclavian artery and anastomosis of the subclavian artery to the PA), Potts shunt (direct connection between the left PA and the descending aorta), and Waterston shunt (direct connection between the posterior portion of the ascending aorta and the right PA where it courses behind the aorta) remain mostly of historical interest only. At present the modified Blalock-Taussig shunt remains the choice for augmenting pulmonary blood flow in the neonate. Introduced by de Leval et al. in 1975, this aortopulmonary shunt is a modification of the original description of the Blalock-Taussig shunt in 1944. It involves interposing an expanded polytetrafluoroethylene (ePTFE; Gore-Tex) graft between the innominate or subclavian artery and the ipsilateral PA ( Fig. 65.6 ). The goal of the operation is to provide pulmonary blood flow to obtain a systemic oxygen saturation of approximately 75% to 85%. This provides a Q p :Q s of 1 to 1.8 : 1. Depending on the size of the neonate and on whether there is an additional source of pulmonary blood flow (such as across a restrictive BVF), the most commonly used shunts are 3.5 mm or 4.0 mm in diameter. As shown by the Congenital Heart Surgeons Society study, an indexed shunt size of 0.8 to 1.0 results in more favorable outcomes (mortality and successfully transitioning to a cavopulmonary shunt). The provision of a stable source of pulmonary blood flow allows for symmetric growth of the PAs without overcirculation, thus allowing favorable anatomic conditions for future cavopulmonary anastomosis.

The postoperative management of the newborn with an aortopulmonary shunt can be challenging. Optimum management requires meticulous attention to the Q p :Q s and often involves titration of inotropic medications to ensure adequate systemic perfusion combined with ventilator management to minimize overcirculation. The goals are to maintain adequate shunt flow, arterial oxygen saturation (SaO 2 ) between 75% and 85%, and adequate ventricular function. If ventricular function is adequate to manage the increased cardiac output demands of increased Q p :Q s , many babies tolerate oxygen saturations of 85% to 90% quite well. Use of near-infrared spectroscopy (NIRS) has become standard with these neonates, and trends in both the cerebral and somatic NIRS may offer early warning signs of poor systemic perfusion. The differential diagnosis for low SaO 2 includes hypotension, shunt stenosis ( Fig. 65.7 ) or occlusion, lung disease (pulmonary venous desaturation), and impaired ventricular function (or low cardiac output). Although many surgeons favor subtherapeutic “low-dose” unfractionated heparin drip in the immediate postoperative course, which can be later transitioned to enteral aspirin to maintain shunt patency, there are no data to support the use of subtherapeutic heparin, and the efficacy of long-term use of aspirin may depend on platelet response to aspirin as measured by current assays. Some studies have shown that a significant number of neonates and infants are refractory to aspirin with respect to platelet effect. More recently, some surgeons have favored using ePTFE grafts that are infused with heparin, although the benefit of these grafts is also unproven. Perhaps the most important factor for graft patency is the technical adequacy of the anastomosis and the quality of the vessels to which the graft is connected.

Excessive pulmonary overcirculation may be manifested by high SaO 2 levels, pulmonary edema, and failure to wean from mechanical ventilation. This often requires manipulation of the PVR by judicious use of milrinone, permissive hypercapnia, and selective inotropic support to improve the ability of the heart to maintain the increased cardiac output demands of a high Q p :Q s . In extreme circumstances, persistent pulmonary overcirculation may also require operative revision to reduce shunt size (often by “clipping” or banding the shunt). An important factor in this type of circumstance is whether or not the ductus was left open at the time of shunt creation. Many surgeons leave the ductus alone and allow it to close by discontinuation of prostaglandin infusion. This has the advantage that if a shunt occludes in the postoperative period, the intensive care unit (ICU) team can reinstitute prostaglandin in hopes of restoring ductal patency. (The logic behind this is simply that in the era of classic BT shunts, performed via a thoracotomy, the PDA was never ligated). Occasionally, however, a PDA does not close after the cessation of prostaglandins and can create a significant additional source of pulmonary blood flow that may need surgical attention. Although this is unusual, it is important for the ICU team to know whether or not the ductus was ligated at the time of surgical shunt placement.
As mentioned earlier in this chapter, the management of patients with shunt physiology is potentially complex. Although the Q p :Q s is an important determinant, it is merely a ratio and not an actual number. It is also important for the management team to assess the overall systemic oxygen delivery as a reflection of cardiac output. For example, if there is truly excessive pulmonary overcirculation, there may be a low systemic cardiac output, and this drives down the mixed venous O 2 saturation. If the mixed venous O 2 saturation is 40%, and there is a 5 : 1 shunt, but the pulmonary venous saturation is 90% due to pulmonary edema, then the O 2 saturation might be 81% (five parts 90 = 450 and one part 40 gives a mixing in the heart of 490, which divided by 6 provides a mixed saturation of 81). Although this may seem ideal, it clearly is not and will become manifest by rising lactate levels. Conversely, a patient may have a saturation of 93% and normal lactate levels. This would reflect that the heart is capable of maintaining excessive additional cardiac output (as much as six outputs to the lung for every output to the body), and, although this may not be a good long-term situation, if it is tolerable, it can be managed with systemic afterload reduction, permissive hypercapnia, and, if necessary, investigation into whether or not there is an additional source of pulmonary flow, such as across a patent ductus or BVF. The point to illustrate is that patients with shunts have abnormal circulation, and the astute management team takes into account a variety of factors beyond the Q p :Q s in determining whether or not the patient is well palliated.
Hypoxemia in the immediate postoperative period represents an emergency. Absence of a “shunt murmur” (in a hypoxemic patient) represents shunt thrombosis until proven otherwise. Confirmation by echocardiography is important, and this usually requires emergent return to the operating room for revision or may even require institution of extracorporeal membrane oxygenation support if the operating room is not available. In instances in which the ductus was not ligated, rapid reinstitution of PGE 1 infusion may be lifesaving. Less acute and severe hypoxemia can sometimes be managed in the catheterization laboratory by balloon angioplasty or stenting of the shunt (see Fig. 65.7 ). Other diagnoses in the differential of postoperative hypoxemia include low cardiac output, lung disease, atelectasis, and phrenic nerve paralysis.
Management of the Newborn With Increased Pulmonary Blood Flow
Infants with increased pulmonary blood flow who present with physiologically important “pulmonary overcirculation” will demonstrate significant clinical effects such as lactic acidosis, poor renal function with rising blood urea nitrogen or creatinine level, and even pulmonary edema—which may actually affect oxygenation leading to hypoxemia, despite the excessive pulmonary blood flow (which is why Q p :Q s is a guideline to be used in conjunction with the overall clinical picture) ( Fig. 65.8A ). Patients with transposed great vessels and TA may actually have significant systemic outflow obstruction (either at the subaortic level or at the level of the aortic arch and isthmus or both). This combination of defects creates the dual problem of decreased systemic perfusion and pulmonary overcirculation; and when there is aortic arch hypoplasia or a prominent coarctation, these infants may be ductal dependent for systemic perfusion and require, despite their “pulmonary overcirculation,” the institution of PGE 1 for maintenance of ductal patency to perfuse the body (when the aorta is obstructed). These are complex circumstances. When pulmonary overcirculation is simply created by flow across a large BVF (VSD) into normal pulmonary arteries (without aortic obstruction), then consideration can be given to placing a PA band. Strict control of the pulmonary blood flow is required to protect the lung bed and prevent lung disease because the ultimate goal of palliation is a Fontan. PA banding was originally introduced by Muller in 1952. Providers must be cognizant of the fact that PA banding may accelerate narrowing of the BVF over time with ensuing subaortic obstruction and cyanosis. Further, PA distortion and ventricular hypertrophy represent additional risks inherent to PA banding. Patients who receive a PA band to control excessive pulmonary blood flow are at risk for developing rapidly progressive cyanosis that may necessitate further intervention in a short-term time frame.

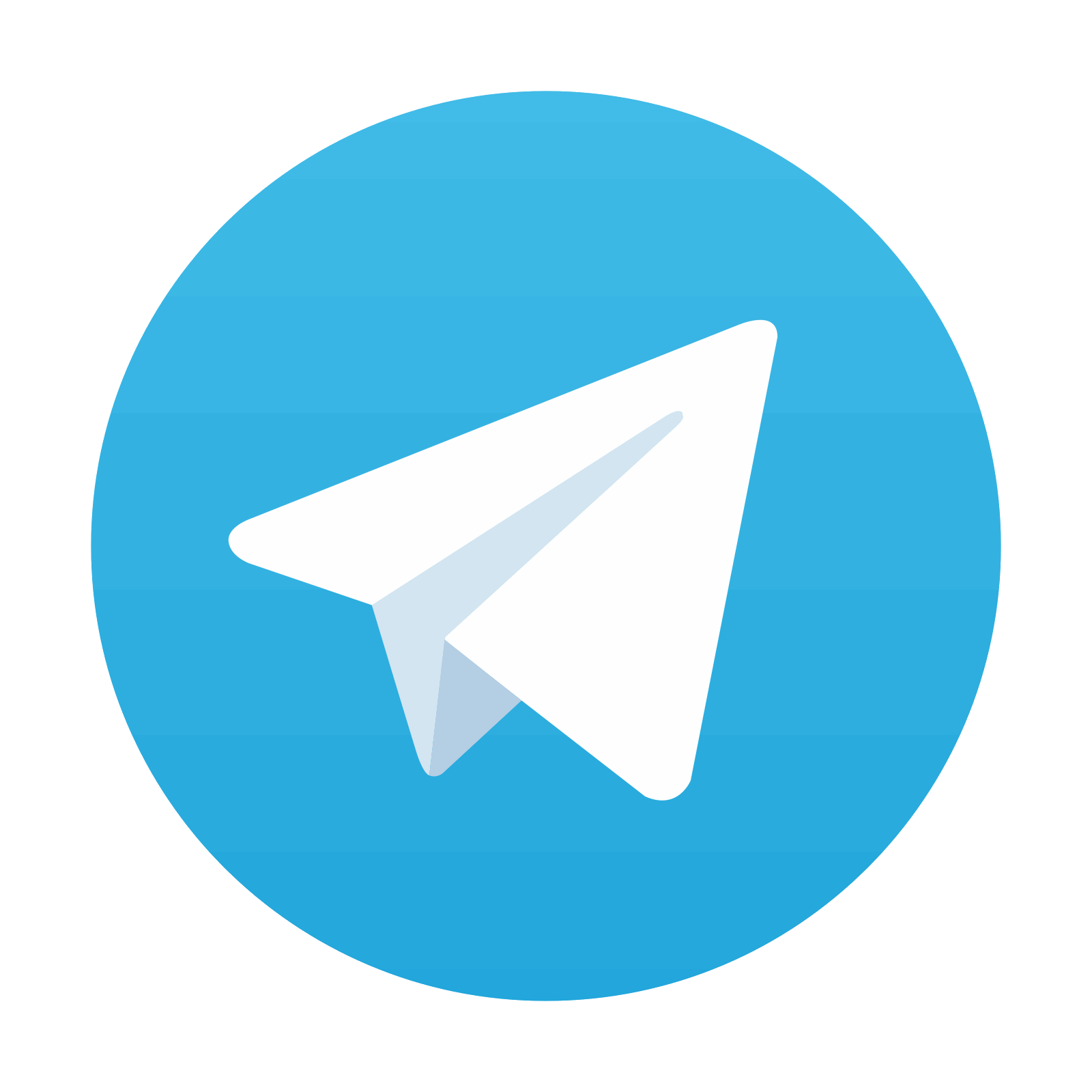
Stay updated, free articles. Join our Telegram channel

Full access? Get Clinical Tree
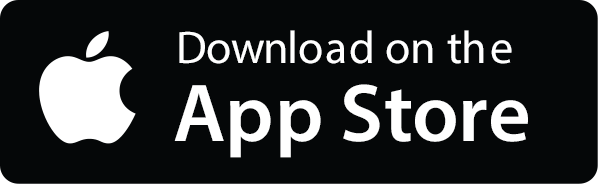
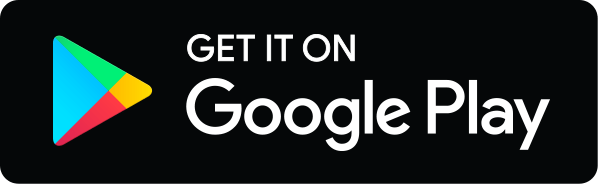
