Unacclimatized lowlanders at high altitude Genetics and -omics in unacclimatized individuals traveling to high altitude Physiologic effects of chronic intermittent hypoxia Having examined the phenotypic variation and other health issues in long-term residents of high altitude in Chapter 4, we now examine another major group of people who experience high altitude on a regular basis, unacclimatized lowlanders traveling to high altitude. In addition to the ever-growing number of climbers, trekkers, and other people engaged in sports and leisure activities at high altitude, this includes an increasing number of people who go to high altitude to work in a variety of different occupations, including mining, astronomy and other commercial and scientific endeavors, and military service. After reviewing the epidemiology of the increasing leisure and recreation activities at high altitude, the chapter describes the various forms of work-related travel to high altitude with an emphasis on the mining operations, radio telescopes, and military operations. We then examine the genetics and -omics that underlie many of the physiological responses to acute hypoxia and explore the physiology of chronic intermittent hypoxia, a unique form of hypoxic exposure associated with work at high altitude. The chapter concludes with a discussion of methods for mitigating risk associated with travel to high altitude for both leisure and work-related purposes, with additional attention to the topic of room oxygen enrichment of both stationary facilities and high altitude railway cars. The physiologic responses to acute hypoxia and the main clinical problems faced by unacclimatized lowlanders traveling and working at high altitude are the focus of the later chapters of this book. There are two major groups of unacclimatized lowlanders who ascend to high altitude, those engaged in a wide range of endeavors that are best classified as leisure activities and those working at high altitude on an intermittent basis. Of the unacclimatized lowlanders traveling to high altitude, the largest numbers are clearly those engaged in recreational and athletic endeavors. It has been estimated, for example, that in 2013, more than 100 million people visited regions at high altitude (Burtscher et al. 2013). By way of other examples, the number of tourists entering Tibet increased from 2.5 million in 2006 to more than 15 million in 2014, (National Bureau of Statistics of China 2018), while in the western United States, 87 million people visit Colorado on an annual basis with more than one-third visiting a mountain resort (Colorado Tourism Office 2018) and more than 590,000 people alone visiting the summit of Pikes Peak (4301 m) (Pikes Peak—America’s Mountain 2019). One of the highest profile groups of high altitude travelers are individuals engaged in trekking and mountain climbing in various regions of the world. For example, steadily increasing numbers of people have visited the Sagarmatha National Park in the Mount Everest region in the past two decades including more than 53,000 people in 2019 alone (Sagamartha National Park Office 2020) (Figure 5.1). In 2019, the Himalayan Database reported that more than 660 people summited Everest from the Nepal side and an additional 216 summited from the Northeast Ridge in Tibet (Figure 5.2). With these increasing numbers have come increasing risk for climbers. In the spring of 2019, for example, crowding high on the mountain during the narrow summit window was of major concern, as climbers waited in long queues to reach the summit of Everest, and a total of 11 people died. Figure 5.1Annual number of entrants to Sagarmatha National Park from 1998 to 2018. There were significant decreases in park visitation during the Maoist insurrection from 1996 to 2006 and as a result of the Nepal earthquake in 2015. (Source: Sagarmatha National Park Office, Namche Bazar, Solokhumbu.) Figure 5.2Total number of climbers (gray bars) and number of successful ascents (blue bars) of Mount Everest (A) and all 8000 m peaks in the world (B). Figures created with data from the Himalayan Database (2004). One particular area of high altitude recreational activities that has seen a surge of interest in recent years is athletic events conducted at high altitude. This includes events such as the Everest Marathon, which starts at Everest base camp (5365 m) on May 29th each year in celebration of Tenzing Norgay Sherpa and Sir Edmund Hillary’s first successful climb of the mountain on May 29, 1953. Numerous other events exist, including, but not limited to, the Leadville 100 running and mountain bike races in Leadville, Colorado; the Western States 100 ultramarathon in the Sierra Nevada Mountains, California; the 171-km Ultra-Trail du Mont Blanc, which utilizes the Tour du Mont Blanc and runs through Switzerland, Italy, and France; and the Patrouille des Glaciers ski mountaineering race in the Swiss Alps. Regardless of the particular activity in which they are engaged, all of these recreational high altitude travelers, as well as the groups discussed in the remainder of this chapter, are at risk for developing one of several forms of acute altitude illness—acute mountain sickness (AMS), high altitude cerebral edema (HACE), and high altitude pulmonary edema (HAPE). The clinical aspects, pathophysiology, and management of these problems are described in greater detail in Chapters 20–22, while issues faced by travelers with underlying medical conditions are considered in Chapter 25. Athletes engaged in races such as those mentioned earlier must face the additional challenge of decreases in exercise capacity at high altitude and resolve questions such as how long to spend at high altitude prior to their planned athletic event. These issues are considered further in Chapter 18. While much of the attention regarding nonoccupational travel to high altitude is focused on trekkers and climbers, far greater numbers of people participate in religious pilgrimages to various high altitude locations on an annual basis and have been doing so since well before trekking and climbing began in various regions of the world (Basnyat 2006; 2014). Although precise information is lacking, it has been estimated that more than 2 million people travel to elevations between 3100 m and 4600 m as part of at least seven major and several other lesser festivals in the Himalaya with the largest being in Badrinath (3100 m) and Kedarnath (3500 m), India, between May and October. These two festivals attract an estimated 1 million and 600,000 people, respectively, each year (Basnyat 2014). Many pilgrims try to participate in as many of these pilgrimages as possible over their lifetimes as they serve as important opportunities for spiritual fulfillment and a path to salvation. At the same time, these pilgrimages pose significant physical challenges to the participants and, in many cases, a threat to health and physical well-being. In addition to infection control issues related to overcrowding and poor hygienic conditions, many participants ascend to high elevation at overly rapid rates, which significantly increases the risk of the acute altitude illnesses described in Chapters 20–22 (Basnyat et al. 2000; MacInnis et al. 2013). This problem is exacerbated by a general lack of awareness of acute altitude illness, underlying health problems and poor physical fitness among many pilgrims, as well as the fact that fasting is a common practice during the pilgrimages (Basnyat 2014). A further problem is that many pilgrims believe that physical suffering is an integral part of the experience and may even value completion of the pilgrimage over protecting their physical well-being. With increasing recognition of these problems, efforts have been made by groups such as the Himalayan Rescue Association (HRA) in Nepal to educate pilgrims and health care providers about the importance of pretravel counseling, how best to protect themselves during the pilgrimage itself, and how to set up and staff clinics, such as the temporary HRA clinic at Lake Gosainkunda (4300 m) during the August pilgrimage to that location. Even if they are not engaged in recreational or religious activities or athletic competition, some unacclimatized lowlanders are acutely exposed to hypobaric hypoxia in the course of rail travel including the central railway of Peru, which crosses the Andean crest at Ticlio at an altitude of 4800 m and the more recently completed railway from Golmud in Qinghai Province, China (2810 m), to Lhasa, Tibet (3656 m). The latter is more than 1100 km in length with more than three-quarters of the distance above 4000 m altitude. In fact, the track crosses some of the highest ranges on the Tibetan plateau, the highest altitude being just over 5000 m. Travel along the railway poses risks of acute altitude illness among the train’s passengers (Wu et al. 2010) while the more than 100,000 construction workers reported to have been employed during construction also faced a significant burden of these and other medical problems (Wu et al. 2007a; 2009). One of the most challenging problems was constructing the tunnels at very high altitudes, for example the Fenghuoshan Tunnel at an altitude of 4905 m. Excavations were done with hand drills and the physical demands on the workers were enormous. To partially relieve the hypoxia, large volumes of high concentration oxygen were produced by generators located outside the tunnel, and the gas was pumped to the workface through a large pipe. It was reported that this increased the inspired PO2 by as much as 15 mmHg, which would have reduced the equivalent altitude by about 1200 m. Another remarkable challenge was the Mount Kunlun Tunnel at an altitude of 4660 m, which is 1686 m long and believed to be the longest tunnel through permafrost in the world. Road building is also increasing in many mountainous regions previously only accessible by air or trekking such as northern India and parts of Nepal. While road access is helpful from a socioeconomic standpoint, as it increases the speed of travel and facilitates faster and cheaper transport of supplies to these regions, it also raises the risk that individuals ascend to higher elevations at too rapid a rate and, as a result, face an increased risk of acute altitude illness. For example, after completion of a road from Besisahar (760 m) to Manang (3540 m) in the Annapurna region of Nepal in December 2014, a greater number of individuals were diagnosed with acute altitude illness at the HRA clinic in Manang when compared to a several-year period before road completion with the number of patients with acute altitude illness increasing in tandem with the number of people arriving in Nepal by motor vehicle (Figure 5.3) (Reisman et al. 2017). To meet the public health challenges posed by this situation, it will be necessary to increase efforts to educate the people traveling by road and also to build medical infrastructure in these areas seeing increasing numbers of travelers. Figure 5.3Mode and duration of travel and demographic characteristics of patients diagnosed with acute altitude illness at the Himalayan Rescue Association clinic in Manang, Nepal (3540 m), from the fall of 2010 to the fall of 2016. The road from Besisahar (760 m) to Manang was completed in 2014. (Source: Reisman et al. 2017.) There is a wide array of reasons for which individuals ascend to high altitude for work. While it is not possible to discuss all of the occupations that bring people to high altitude for work (e.g., porters, mountain guides, search and rescue, etc.), it is worth considering several categories of such work, as they provide examples of the physiological and logistical challenges associated with occupational exposure to high altitude as well as the different patterns of exposure to hypobaric hypoxia. As outlined later in this chapter, there are important neurocognitive implications of exposure to high altitude for occupational work. Mistakes in judgment in a mine can be disastrous, telescope equipment is extremely expensive and sensitive, and military operations require troops to be combat-ready and alert at all times. Mining activities have been conducted at high altitudes for many centuries. Chinese sources suggest that Tibetans worked as high as 6000 m in the Tanggula range of central Tibet mining quartz; chromate mines were also found in central Tibet (Ward 1990). The open cast mines at Thok Jalung (thok is Tibetan for gold) were investigated in 1867 by Nain Singh, one of the early clandestine native explorers of the Survey of India (Waller 1990). In several areas of the South American Andes, there is evidence that mining activities were carried out by the Incas before the Spanish conquest. The Spanish conquistadors founded the imperial city of Potosí (4060 m) in Bolivia, the site of an enormous silver mine, in the 1540s. Today, many mines are still located above 4000 m in Tibet and South America. In the former, for example, copper and gold are mined in the Shetongmon, Gyama, and Yulong districts as China expands its quest for resources (Lafitte 2011), while 10 mines are located between 4800 m and 5000 m in Peru, Bolivia, and Chile from which a variety of minerals are extracted including copper, gold, lead, molybdenum silver, tin, and zinc (Arendal 2020). A large amount of infrastructure is necessary in communities proximate to a mine, including schools, medical facilities, and meeting halls, all of which increases the expenses of the mine. In some locales, notably in South America where low and high altitude regions are often close geographically, these considerations have led some mining operations to develop a commuting pattern where the families live at or near sea level and the miners commute to the mine itself where they spend a period of 7–10 days. In Tibet, on the other hand, due to the extent of the Tibetan plateau, commuting on a frequent basis is difficult. Miners must either reside on a permanent basis or at least for much longer periods of residence reaching into months and years. Thus the “commuting” approach to working at high altitude applies only to areas where descent to low altitudes is practical, safe, cheap, and quickly achieved. An example of the commuting pattern is provided by the Collahuasi mine, a very large, open-cut copper mine in northern Chile. The working areas of the mine are at altitudes of 4400–4600 m, although the mining camp where the miners sleep is at 3800 m. There are currently several thousand people working at the mine, which makes it one of the largest copper mines in the world. The miners’ families live at sea level in Iquique in accommodations supplied by the mining company. Within a few hours, the miners are transported to the mine by special buses, which ride on roads built by the mining company. Miners typically spend seven days at the mine, where they work for up to 12 hours per day, and then sleep in the mining camp at an altitude of 3800 m. At the end of seven days, they are transported by bus down to Iquique, where they spend the next seven days with their families. This 7 × 7 schedule is not universally employed in the high altitude mines that use commuting, as periods of work lasting from 10 to 21 days have also been used. In contrast to mining, the practice of siting telescopes at high altitude is a considerably more recent phenomenon. There are several advantages in placing telescopes at high altitudes. One is improvements in image quality due to the fact that the instrument is above much of earth’s atmosphere, which otherwise absorbs some of the optical and radio waves. Another advantage is that in some areas, such as the Chajnantor plateau, the atmosphere is extremely dry and absorption of radio waves by water vapor is therefore much less. Finally, remote mountain sites tend to have little light or radio wave pollution, although this advantage can also be achieved in other remote areas at lower altitudes. Two of the major telescopes are described next. This site is located on the extinct volcano on the big island of Hawaii and has an international complex of least 12 facilities housing 13 telescopes within a 525-acre special land use zone referred to as the Astronomy Precinct near the 4200 m summit. Mauna Kea is less than 100 km from the sea-level city of Hilo, and it is possible to drive from one site to the other in a couple of hours. There is also an intermediate station with dormitories at 3000 m at Hale Pohaku, where some newcomers can spend a night there before going to the summit. However, the majority of the staff who operate the telescopes commute from sea level to the summit every day, where they are exposed to an average barometric pressure at the summit of about 465 mmHg, an inspired PO2 of 87 mmHg, and an alveolar PO2 of about 45 mmHg with acute exposure. Another major telescope site is Llano de Chajnantor in northern Chile’s Atacama Desert. This area is easily accessible by road from San Pedro de Atacama (altitude 2440 m), the first part of which is an international highway leading from Chile to Bolivia and Argentina. The drive from San Pedro de Atacama to Chajnantor takes about 1 hour, making it one of the few places in the world where it is possible to reach an altitude of 5000 m so quickly by road. In addition to the ease of accessing the facility, another advantage of the site is the fact that it sits in the rain shadow of the Andes Mountains and the Chilean Coastal Range, making it one of the driest places on earth. The barometric pressure at the site, altitude 5050 m, is about 420 mmHg, with an inspired PO2 of only 78 mmHg. There are two major telescope projects at this site. The first is the Atacama Large Millimeter/submillimeter Array (ALMA), a large project jointly run by the National Radio Astronomy Observatory (NRAO) (United States) and the European Southern Observatory (Figure 5.4). ALMA uses 66 high-precision dish antennas, including 54 12-m antennas and 12 7-m antennas, amounting to a total collecting area of more than 71,000 square feet (NRAO 2020). The second major project is the Atacama Pathfinder Experiment (APEX), a 12-m diameter antenna located at 5100 m and jointly run by the Max Planck Institute for Radioastronomy (Germany), the Onsala Space Observatory (Sweden), and the European Southern Observatory. Figure 5.4Some of the antenna dishes of the ALMA radio telescope at an altitude of 5050 m. The huge transporter that moves the telescopes up to the site is shown on the right. The most noteworthy military campaign at high altitude is the conflict between India and Pakistan over the Jammu-Kashmir region, in which large numbers of soldiers have been stationed at altitudes up to 7000 m for substantial periods of time. Many of these soldiers come from low elevations within these countries, and as a result are at risk for acute forms of altitude illness and problems associated with more prolonged stays. Classic early work on the risks to human health during acute exposure to very high altitudes was provided by Singh et al. (1969), while further accounts of the issues faced by the soldiers have been provided by Anand et al. (1990). Their work included one of the first descriptions of “adult subacute mountain sickness,” a particular form of altitude illness marked by the development of right heart failure and peripheral edema (Anand et al. 1990). Other problems have also been noted among these soldiers including a high incidence of stroke (Jha et al. 2002). Additional military operations have been conducted at high altitudes, including the Soviet and later United States-NATO conflicts in Afghanistan. The pattern of exposure to high altitude varies significantly from conflict to conflict. Whereas soldiers in the India-Pakistan conflict reside for months at a time at high elevation, soldiers in the Afghanistan theater and other locations may reside at bases at moderate elevation but deploy in a rapid fashion to higher elevation for various operations. As discussed in Chapter 7, humans acclimatize to hypoxia through a series of physiologic responses, and individual tolerance of acute and chronic hypoxia varies considerably. Despite similar exposure to the same hypoxic stress, some individuals develop acute altitude illness (AMS, HACE, or HAPE) while others remain healthy. Genomic studies have set a foundation for other large-scale -omics avenues of research, including the analysis of transcriptomic and epigenomic (gene expression), proteomic, and metabolomic profiles that may be altered under environmental conditions. In studies of genetics and -omics, the ultimate goals are to provide predictive insight, preventative measures, and potentially tailored treatment for individuals with innate susceptibility to stresses experienced at altitude. Efforts to characterize these profiles and identify molecular functions and regulatory changes that occur at the tissue, cellular, or molecular level in individuals traveling to high altitude have increased within the past decade. How these factors underlie particular responses to hypoxia, in coordination with or independent of genetic factors, to influence acclimatization is a growing area of research (Bhandari and Cavalleri 2019). Whether genetic factors are able to predict susceptibility to exaggerated hypoxemia and acute altitude illness is an interesting and important subject that remains at an early stage of understanding (MacInnis et al. 2010; MacInnis et al. 2011; Rupert and Koehle 2006). Twin studies have demonstrated heritable control of key acclimatization responses, such as ventilatory and exercise response under conditions of hypoxia, indicating these physiological responses likely have a genetic basis (Masschelein et al. 2015). Population differences further suggest a genetic basis for high altitude diseases, as a higher frequency of AMS was reported in Han Chinese than Tibetans (Wu 2005; Wu et al. 2009). However, studies seeking to identify the underpinnings of AMS, for example, have been challenging due to limitations that include subjective diagnostic criteria, unclear pathophysiology, and isolated candidate-gene approaches that have yet to identify a genetic variant with clinical efficacy (MacInnis et al. 2010; MacInnis et al. 2011). While no genetic associations have been reported for HACE, various candidate genes have been investigated as potential contributors to HAPE. Recent genome-wide analyses suggest tissue inhibitor of metalloproteinase 3 (TIMP3), a gene whose product regulates the degradation of the extracellular matrix of lung tissue, is associated with HAPE in Japanese individuals (Kobayashi et al. 2013), and intronic variants of the EGLN1 gene have been associated with HAPE in a population from India (Aggarwal et al. 2010). Further research with standardized assessments and large sample sizes are needed to identify and replicate associations with altitude-related illnesses. In addition, studies of -omics data characterized from individuals ascending to high altitude may, when combined with genetic data, help elucidate key genetic pathways involved in acclimatization and/or susceptibility to altitude illnesses. An emerging field of study based on environmentally induced cues centers on epigenetic modifications that afford plasticity and/or inherited alterations in gene expression. Unlike genetic adaptation, which can take many generations to have an observable effect, epigenetic adaptation can occur in a shorter amount of time, ultimately providing a short-term avenue for adapting to a demanding environment (Giuliani et al. 2015). Epigenetics plays a key role in the response to hypoxia, both at the organism as well as the cellular level, which is well-documented in cancer biology (Watson et al. 2010). Limited studies thus far indicate that incremental exposure to hypoxia may alter the transcription of particular genes due to epigenetic modifications (Nanduri et al. 2017). Efforts to understand the long-term developmental and short-term epigenetic effects on transcriptional and physiological responses to hypoxia, and how these influence acclimatization in travelers to high altitude, are expected to be an increasingly active area of research. Other studies have attempted to identify novel patterns in proteomics, the global analysis of proteins (Altelaar et al. 2013), for AMS. Julian et al. (2014) measured plasma protein levels of individuals (natives of Colorado) at low altitude and then after acute hypobaric hypoxia exposure, comparing the protein levels of those susceptible to AMS and those resistant to AMS. They found an increase in the abundance of proteins with antioxidant properties in individuals susceptible to AMS but not in those resistant to AMS. Lu et al. (2018) conducted a similar study in individuals of Chinese ancestry and found proteins related to tricarboxylic acid cycle, glycolysis, ribosome, and proteasome were significantly reduced in the AMS-resistant but not the AMS-susceptible group. Jain et al. (2018) analyzed saliva for potential proteomic biomarkers and found increased levels of antioxidant enzymes in addition to several other proteins, including apoptosis inducing factor-2. These results indicated that proteomic analysis of saliva may be a feasible, noninvasive method to measure acclimatization and, importantly, failure to acclimatize in disease states such as HAPE or HACE. Metabolomic profiles, which provide information on the metabolites within cells, tissues, or organisms, have also been characterized in individuals at sea level and high altitude. Sun et al. (2016) employed nonbiased high-throughput metabolomics for 21 volunteers taken from sea level to 5260 m and found that erythrocyte S1P, G-protein coupled receptor for the bioactive lysosphingolipid sphingosine 1-phosphate, increased and remained elevated for 16 days at high altitude and was correlated with increased erythrocyte sphingosine kinase 1 activity and hemoglobin oxygen release capacity. These results indicated a shift toward glycolysis when oxygen becomes scarce, which is consistent with other metabolomic studies of hypoxia performed in hypobaric chambers (Tissot van Patot et al. 2009). It has been further suggested that a “metabolic memory” persists beyond the initial exposure to hypoxia, as increased glycolysis remained for up to a week after returning to normoxia (d’Alessandro et al. 2016). Liu et al. (2016) showed that production of erythrocyte 2,3-bisphosphoglycerate (2,3-BPG), a negative allosteric regulator of hemoglobin-oxygen binding affinity, was induced by altitude, remained at increased levels for up to 16 days, and was associated with plasma adenosine concentrations and soluble CD73 activity. While these studies were based on limited sample sizes, the Caudwell Xtreme Everest study (Levett et al. 2010), with more than 200 individuals assessed periodically as they ascended from lowland to Everest base camp (5300 m), obtained both metabolomic and lipidomic information (O’Brien et al. 2019). They also identified metabolic shifts that pointed toward an increased glycolytic rate (i.e., decreasing isoleucine and glucose; increasing lactate) as well as fat-store mobilization (i.e., decreasing triglycerides; de novo lipogenesis; increasing levels of free fatty acids such as palmitic, linoleic, and oleic acids) with ascent. Overall, the studies to date support the notion that metabolic changes occur as early as within a few hours of ascending and have a cascade of downstream effects that can last up to a week or longer. The metabolome has also been characterized in individuals with HAPE (Luo et al. 2012). Comparisons between 10 individuals with HAPE and 10 without revealed that HAPE-susceptible individuals exhibited higher valine, lysine, leucine, isoleucine, glycerol phosphoryl choline, glycine, glutamine, glutamic acid, creatinine, citrate, and methyl histidine levels and decreases in α- and β-glucose, trimethylamine, and the metabolic products of lipids. These pathways provide novel insights into acclimatization for future investigations. While information regarding the pathways involved in acclimatization are starting to emerge, the ability to predict susceptibility to altitude illnesses will require much further research. It also is important to consider that individuals with specific medical conditions may develop increased hypoxemia at altitude, which may lead to increased risk of acute altitude illness. However, determining who will develop exaggerated hypoxemia in the context of certain disease states is challenging. These concerns and other important considerations for travelers to high altitude are provided in Chapter 25. We expect future editions of this textbook will include important new information about genomics throughout the book as our understanding broadens across the spectrum of human adaptation and acclimatization to high altitude. Unacclimatized lowlanders who ascend to high altitude are exposed to the effects of acute hypoxia within minutes to days of their arrival at high altitude. In some cases, individuals ascend for tourism or work to a given elevation and stay there for a period of time, whereas others, such as mountain climbers, will continually ascend toward the summit, with occasional strategic descents to lower altitudes, before leaving the mountain. While this is the most common type of hypoxic exposure experienced by lowlanders ascending to high altitude, chronic intermittent hypoxia is another way by which unacclimatized lowlanders experience hypoxia. Chronic intermittent hypoxia (CIH) describes the experience of spending blocks of time at high altitude punctuated by periods of time at lower elevation. As outlined earlier in this chapter, numerous people, such as workers of mining companies, employees of road construction companies, workers on telescopes or military divisions in high altitude border areas are exposed repetitively to high altitude over a long time by commuting between high altitude and lowland areas. Although the effects of the long-term exposure to this form of chronic intermittent hypoxia on the human physiological responses have been poorly established, the next section reviews what is known about the hematological, respiratory, circulatory, exercise, and neurocognitive responses in these workers. Both between and within given occupations (e.g., mining or military, etc.), there is a diverse combination of days spent working at high altitude and sea level. For example, while miners often have a range of shorter-term patterns of work (e.g., 7 × 7, 14 × 14, 4 × 3, or 11 × 3 days at high altitude and at or close to sea level, respectively), many military interventions involve 3–12 month deployment at higher altitudes. As such, it is challenging to make comparisons to anything other than within a particular work paradigm. It should also be noted that CIH in this occupational setting is very different than that experienced by individuals with sleep disordered breathing at sea level in that the cycle lengths of hypoxia and normoxia are considerably longer for people traveling and working to high altitude. These issues are discussed further in Chapter 17. As discussed in Chapter 13, hypoxia stimulates the release of erythropoietin (EPO) from the kidney within 2–12 hours of exposure (Lacombe et al. 1988; Milledge and Cotes 1985; Vizcardo-Galindo et al. 2020), which acts to increase the number of red blood cells, normally reaching a plateau after ∼10 days at a given altitude (Abbrecht and Littell 1972; Milledge and Cotes 1985). This subsequently leads to increases in hematocrit and hemoglobin concentrations and, as a result, arterial oxygen content. This response is also present in miners exposed to high altitude on an intermittent basis. For example, in a cross-sectional study of 266 men working intermittently at high altitude (14 × 14 days day commuting to 3800 m), there was a positive correlation between the hemoglobin values and the number of years the commuters worked under this regimen (Akunov et al. 2018) (Figure 5.5). However, it appears that the rise in hemoglobin concentration is slower with CIH when compared to sustained hypoxic exposure (Akunov et al. 2018; Brito et al. 2007; Richalet et al. 2002). For example, Richalet et al. (2002) found increased hematocrit after 12 and 19 months of CIH (7 × 7 day commuting to 3800–4600 m), which returned to baseline at 31 months of continued shift work (Figure 5.6). How EPO signaling is “turned off” only after nearly three years of CIH is unknown. In miners exposed to CIH for 12 years or more in a regimen of four days at 3550 m and three days at sea level, hematocrit and hemoglobin reached a plateau at lower values than seen in residents living at or above 4000 m (Akunov et al. 2018). Together, this limited data indicate there is a progressive increase in hematocrit and hemoglobin during the first few years of CIH that stabilizes within 5–10 years and remains lower than permanent residents of high altitude (Akunov et al. 2018; Beall 2006; Heinicke et al. 2003; Moraga et al. 2018b; Prommer et al. 2007; Richalet et al. 2002). Following descent from high altitude during CIH, potential rapid reductions in hemoglobin mass (Ryan et al. 2014) via neocytolysis (Rice et al. 2001) may mediate the maintained hematocrit and hemoglobin levels. Figure 5.5Scatter plot of correlation between the hemoglobin (Hb) levels and the years of exposure to intermittent high altitude. The best-fit line is shown, and the shaded area represents the 95% confidence intervals (N = 266). (Source: Akunov et al. 2018.) Figure 5.6Hematocrit of miners before (0) and after 12, 19, and 31 months of exposure to chronic intermittent hypoxia. Seven days were spent at an altitude of 3800–4600 m followed by seven days at sea level; the cycle was repeated for the whole 31 months. Mean ± SD. Time vs. 0: *, **, ***: p
Introduction
Unacclimatized Lowlanders at High Altitude
Leisure and cultural activities
Recreational Activities and Athletic Competitions
Religious Pilgrimages
Railway and Road Travel
Lowlanders working at high altitude
Mining
Telescopes
Mauna Kea
Chajnantor
Military Operations
Genetics and -Omics in Unacclimatized Individuals Traveling to High Altitude
Genetics
Epigenetics
Proteomics
Metabolomics
Physiologic Effects of Chronic Intermittent Hypoxia
Hematologic system
Stay updated, free articles. Join our Telegram channel

Full access? Get Clinical Tree
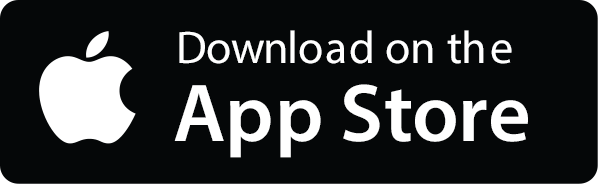
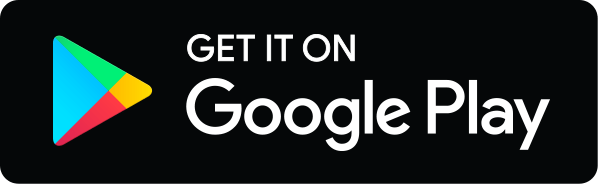