Chapter 9
Traumatic Brain Injury
Written by R. Roos, B.M. Morrow and H. van Aswegen
Traumatic brain injury (TBI) is a problem worldwide, due in part to the high prevalence of road traffic accidents and the presence of violence such as civil unrest and wars. Prior to the twentieth century, severe head injury was most often fatal, but now, due to improved medical management, patients are surviving and the need for rehabilitation services has increased (Cifu et al., 2010). Traumatic brain injury can result in significant impairments, physical limitations and participation restrictions that require rehabilitation. The rehabilitation services available are often limited depending on the country in which the injury occurs. Medical and therapeutic strategies to enhance brain protection in the early phase following TBI is therefore of extreme importance to try and lessen mortality and improve functional outcome.
The information covered in this chapter is focused on the acute care management of a patient with TBI and includes:
•The causes and mechanisms of injury.
•The types of TBI.
•Primary and secondary damage associated with TBI.
•The severity of injury.
•Medical and surgical management of a patient who sustained TBI.
•The physiotherapy aims for the management of a patient who sustained TBI in the intensive care unit and neurosurgical ward.
•The contraindications and precautions related to the physiotherapy management of a patient with TBI.
•Physiotherapy interventions for patients who suffered TBI.
•Adult and paediatric clinical case scenarios.
There is a wealth of information related to the rehabilitation of individuals with TBI in the later stages of rehabilitation. Information for further reading on chronic rehabilitation of a patient with TBI is provided in Section 9.10.
9.1. Causes and Mechanisms of Injury
9.1.1. Injury in adults
Traumatic brain injury is defined as an alteration in brain function or other evidence of brain pathology caused by an external force (CDC, 2011). Events that can lead to TBI include falls, motor vehicle accidents (MVA) and assault (CDC, 2011). Other causes of TBI include blast-related injuries and gun shots. Sixty percent of blast-related injuries will result in TBI due to the primary blast wave alteration in atmospheric pressure, flying shrapnel and the propulsion of an individual against a hard object (Cifu et al., 2010). Motor vehicle accidents are the most common cause of TBI in teenagers and young adults, whereas falls occur more in children or older adults. Individuals more at risk of TBI are males, the elderly and children younger than four years (Marik et al., 2002; CDC, 2011).
9.1.2. Injury in paediatrics
Children are more predisposed to TBI than adults because they have a larger head in relation to body size and lax spinal ligaments. These factors predispose young children to coup and contre-coup brain lesions. It is rare for a child with multiple injuries to present without an associated head injury (Dykes, 1999). The immature brain is more prone to injury and the protective cranial bones are thinner than in adults. Children may lose large volumes of blood with scalp lacerations, which may predispose them to hypovolaemic shock. Scalp lacerations may be accompanied by deeper and more serious head injuries because of the relative lack of protection of the child’s brain through anatomical structures (Weiner and Weinberg, 2000).
Abusive head trauma remains an important cause of trauma death in children under three years old in developed and developing countries, and is an important cause of morbidity in infants and young children (Duhaime et al., 1998; John et al., 2013). Compared to children and adults with accidental head injury, victims of non-accidental head injury are likely to present with more severe symptoms (such as cardiorespiratory compromise, seizures, altered mental status), which lead to severe brain injuries, worse neurologic outcomes and higher mortality (Herman et al., 2011).
Falls are the most common cause of TBI in children between two and four years of age, as they explore higher surfaces such as furniture or jungle gyms. In younger children devices like highchairs and walkers, designed to protect them, have been associated with significant head injuries when the child falls from them. In older children, vehicle-related accidents become a more frequent cause of head injury (bicycles, passengers in cars and victims of pedestrian MVA) (Weiner and Weinberg, 2000).
Crush injuries often occur at home when heavy objects pulled by small children land on their heads (Weiner and Weinberg, 2000). Violent penetrating injuries in children are still relatively uncommon in most geographical areas, but in turbulent communities it is not uncommon for a child to be caught in cross-fire and sustain a gunshot wound. In cases of domestic violence a child may even be used as a human shield. Accidental penetrating injuries may occur as a result of a fall onto a sharp object (Weiner and Weinberg, 2000).
Traumatic brain injury involving adults or children can be classified according to a number of factors, such as the mechanics of injury, location (e.g. configuration of the skull, specific brain pathology), extent (e.g. diffuse or focal injury, primary or secondary damage) and severity of injury (patient level of consciousness).
9.1.3. Forces related to traumatic brain injury
Primary injury refers to the initial traumatic force applied to the head that results in neuronal damage (refer to Section 9.3). Mechanisms related to primary TBI include forces of high or low velocity that result in compression, traction or shearing of brain tissue (Paz and West, 2009). These forces are categorised as deceleration forces (e.g. being struck by a blunt object), acceleration forces (e.g. fall from a height), coup and contre-coup forces (e.g. collision of the head with the steering wheel of a motor vehicle, in which the impact moves the head into extension resulting in posterior contact with the seat) and, lastly, rotational forces (e.g. rolling of a motor vehicle causing torsion of vascular structures). The latter three incidences could result in significant orthopaedic (e.g. multiple rib fractures with pulmonary contusions) and additional neurologic (e.g. spinal cord) injury that may complicate the acute care management of a patient. The physiotherapist working in a trauma ICU should therefore have the necessary translational physiotherapy skills to manage cardiopulmonary as well as orthopaedic or specific neurological complications in a trauma victim who presents with multi-system involvement.
9.2. Types of Traumatic Brain Injury
9.2.1. Open or closed injury
A patient with TBI may present with damage to the skull and brain tissue or structures of the brain only. An open injury results in a brain injury in the presence of a skull fracture. Penetrating injuries such as gunshot or stab injuries result in open head injury. The presence of skull fractures implies that a large force was applied to the patient’s head, and the likelihood of an intracerebral haematoma is increased in such a situation (Fig. 9.1). In a closed injury there is damage to the brain but the skull remains intact, such as in an MVA, assault to the head or sports-related injury (Marik et al., 2002; Paz and West, 2009).
Skull fractures are common during infancy because the cranium is relatively thin. Most simple, undisplaced skull fractures are not associated with underlying brain injury; however, if this has occurred, separation of the edges of the fractured skull may indicate brain swelling or raised intracranial pressure (ICP) (Weiner and Weinberg, 2000).
Diastatic fractures occur uniquely in children when there is traumatic separation of the cranial sutures (most often lamboidal). Growing fractures are sometimes seen, particularly in the toddler age group, in which a diastatic fracture grows as the brain herniates through the torn dura into the fracture site. This type of fracture often presents some time after the acute injury and there is persistent welling or a pulsatile mass of herniated tissue palpable. Urgent repair of the dura is required in such cases (Weiner and Weinberg, 2000).
In adults and children, every case of TBI should be screened for the presence of cervical spine injury, both clinically and radiologically.
9.2.2. Focal or diffuse injury
In addition to being described as an open or closed injury, TBI can also be described as diffuse or focal. These terms are used to describe whether the injury is gross or specific in nature.
9.2.2.1. Focal injury
A focal injury is an injury to a specific part of the brain. The types of focal injuries can be specified as epidural, subdural, subarachnoid and intracerebral haematomas (Marik et al., 2002; Morton et al., 2005). A description of each type is provided below.
9.2.2.1.1. Epidural haematoma
An epidural haematoma develops when blood accumulates between the skull and dura mater. Road traffic accidents, falls, assault or laceration of the middle meningeal artery will result in the development of such a haematoma. It is most often found in the temporal or temporo-parietal region. Quick surgical evacuation of the haematoma usually results in favourable outcomes (Bullock et al., 2006).
9.2.2.1.2. Subdural haematoma
A subdural haematoma develops with bleeding between the dura mater and arachnoid meninges. It occurs more often in a trauma victim than epidural haematoma. Haematoma formation occurs due to damage inflicted on the bridging veins between the cerebral cortex and venous sinus. A subdural haematoma appears as a crescent-shaped collection between the brain and dura on computerised tomography (CT) scan.
Subdural haematomas are the most common forms of intracranial bleeds that occur in infants. In this age group they most commonly occur in the fronto-parietal area by a tearing of the bridging meningeal veins. Subdural haematomas are frequently associated with significant underlying parenchymal damage. Clinically, infants with subdural haematomas often present with initial loss of consciousness and a depressed mental state or they may be comatose. Following CT confirmation of the diagnosis, urgent surgical review and prompt surgical intervention when required is essential. All young children presenting with subdural haematomas should be investigated for possible non-accidental injury (e.g. shaken-baby syndrome), particularly when associated with retinal haemorrhage (Levin, 2010).
9.2.2.1.3. Subarachnoid haematoma
Subarachnoid haematoma refers to bleeding in the subarachnoid space due to the tearing and shearing of micro-vessels. Subarachnoid haematomas often accompany other severe brain injuries, adding to poorer outcomes (Bullock et al., 2006) (Fig. 9.2). Subarachnoid haemorrhage also has non-traumatic causes such as ruptured aneurysm; reports from most clinical settings indicate that a large number of patients with this type of haemorrhage are seen.
9.2.2.1.4. Intracerebral haematoma
Intracerebral haematomas are commonly seen with moderate to severe TBI (Fig. 9.3). The majority of lesions occur in the frontal and temporal lobes. Intracerebral haemorrhage may also be related to a ruptured aneurysm, as mentioned above.
9.2.2.2. Diffuse injury
A diffuse injury such as diffuse axonal injury (DAI) may occur when the head is struck with an object. The rapid acceleration and deceleration forces cause the brain to move forwards and backwards in the skull and different areas of the brain are compressed and stretched. These shearing forces affect axons that transverse a large area of the brain stem. It results in dysfunction of the reticular activating system. This type of injury is often not visible on initial CT scan. It may cause immediate and prolonged unconsciousness and patients may also have a high mortality rate. If they survive, their morbidity is high (Marik et al., 2002; Morton et al., 2005).
9.3. Primary and Secondary Injury Associated with Traumatic Brain Injury
The extent of TBI should be evaluated according to the pathophysiology related to primary and secondary damage. As mentioned previously, the initial traumatic force applied to the head (e.g. fall, assault, penetrating injury) is the primary injury that results in neuronal damage due to contusion, damaged blood vessels and axonal injury. This is the direct mechanical damage caused by the injury and cannot be influenced by therapy received after injury (Werner and Engelhard, 2007).
Secondary injury is the delayed non-mechanical damage that develops over hours and days after the primary injury. The degree of secondary brain injury that the patient develops is related to the extent and duration of cerebral ischaemia and intracranial hypertension (Bullock and Povlishock, 2007; Werner and Engelbrand, 2007; Shirley, 2009). Factors that lead to cerebral ischaemia include hypoxaemia (e.g. pulmonary infection, atelectasis and blood volume loss), systemic hypotension (e.g. blood volume loss due to trauma), cerebral hypoperfusion (e.g. cerebral vasospasm), inflammatory processes (e.g. release of proinflammatory cytokines and free oxygen radicals) and intracranial hypertension. Intracranial hypertension is caused by cerebral hyperperfusion, often as a result of hypercarbia, and cerebral oedema (Werner and Engelbrand, 2007). Figure 9.4 gives an outline of the factors that influence secondary brain injury.
Two groups of patients are more at risk of developing secondary brain damage. These are women, as they are more prone to develop cerebral oedema leading to increased ICP, and also patients with TBI who received anticoagulation therapy on admission (Protheroe and Gwinnutt, 2011).
Intensive care unit (ICU) management of a patient with TBI therefore focuses on limiting secondary damage by managing ICP and cerebral perfusion pressure (CPP) and promoting optimal oxygenation in order to limit cellular injury (Meyer et al., 2010).
9.3.1. Intracranial pressure
Intracranial pressure is the pressure within the cranium and is influenced by the volume occupied by the brain tissue, cerebrospinal fluid (CSF) and cerebral blood flow. The interdependence between ICP and the contents of the rigid skull can be explained using the Monro-Kellie hypothesis. This hypothesis describes the volume-pressure relationship between the brain tissue, CSF and cerebral blood flow. If there is an increase in the volume or amount of either one of these components (brain tissue, CSF or cerebral blood flow), there will be a decrease in one or both of the other components in an attempt to maintain normal ICP. Of the components that take up volume in the skull, CSF can be displaced most rapidly into the spinal epidural space. Alternatively, the absorption rate of CSF can be rapidly increased in an attempt to maintain ICP within normal limits (Mokri, 2001; Dunn, 2002). A rise in ICP will be initiated when this compensatory mechanism becomes exhausted, as is the case with TBI. After TBI the volume of brain tissue increases due to oedema formation and haemorrhage and blood supply to the brain is disturbed. Increased pressure in the skull will lead to a lack of blood supply to the brain cells and eventual hypoxia. Hypoxia in itself is a stimulus for the blood vessels to dilate and for blood pressure (BP) to be raised to allow greater blood flow to the brain, which in turn will contribute to a further rise in ICP (Dunn, 2002). It is known today that aggressive ICP monitoring and treatment in patients with severe TBI lessens mortality and leads to favourable outcomes following critical illness (Stein et al., 2010).
Patients with TBI that present the signs and symptoms in Table 9.1 should undergo ICP monitoring.
Various methods can be used to monitor ICP, including: a) epidural sensor; b) subdural bolt; c) subarachnoid bolt; d) parenchymal catheter; and e) intraventricular catheter (Morton et al., 2005) (Fig. 9.5). The ventricular catheter is the most accurate of the abovementioned devices (Bullock and Povlishock, 2007).
Table 9.1:Indications for ICP monitoring*.
•Glasgow coma scale score less than 9 out of 15 •Prolonged non-neurosurgical surgery •Abnormal findings on CT scan •Age over 40 years with uni- or bilateral motor posturing •Age over 40 years with systolic blood pressure less than 90 mmHg |
*(Fakhry et al., 2004; Bullock and Povlishock, 2007; Mejaddam and Velmahos, 2012).
Fig. 9.5:Intracranial pressure monitoring devices. Printed with permission from The Mayfield Clinic.
Certain pathophysiological changes occur with a raise in ICP and are outlined in Table 9.2 (Morton et al., 2005).
It is important to note that with handling, be it nursing procedures or physiotherapy, ICP will most likely fluctuate.
Key Message
Physiotherapy treatment should not proceed in cases in which ICP is sustained above 25 mmHg despite the administration of additional sedative or paralysing drugs. However, if sputum accumulation in the lungs is the cause for the high ICP, physiotherapy treatment to effectively clear the patient’s airways should be commenced without delay.
Late signs of increased ICP include changes in the level of consciousness, pupil size and reactivity, motor response and vital signs. Examples of late signs of increased ICP are listed in Table 9.3.
Children with open cranial sutures may display bulging of the fontanelles in response to raised ICP.
Table 9.2:Pathophysiological changes associated with increased ICP.
•The microcirculation in the brain parenchyma becomes compromised when ICP increases to 15–20 mmHg •Venous return is impeded and oedema starts to develop in the non-injured brain tissue when ICP increases to 30–35 mmHg •As ICP increases above 40 mmHg, cerebral perfusion can no longer be maintained and brain death is inevitable if these levels of increased pressure are sustained |
Table 9.3:Late signs of increased ICP*.
Sign | Patient presentation |
Level of consciousness | Coma |
Pupil size and reactivity | Papilledema or ipsilateral fixed and dilated pupil or bilateral fixed and dilated pupils in the presence of brain herniation |
Motor response | Abnormal posturing or flaccidity if brain herniation has occurred |
Vital signs | Cushing’s response, which includes hypertension, bradycardia, altered respiratory pattern and increased temperature |
9.3.2. Cerebral perfusion pressure
Cerebral perfusion pressure refers to the BP gradient across the brain. It is calculated with the equation CPP = mean arterial pressure (MAP) — ICP. If MAP is not recorded on the patient’s chart or displayed on the heart rate monitor, it can be calculated using the equation MAP = diastolic blood pressure (DBP) + [(systolic blood pressure (SBP) — DBP)/3]. In a healthy state, cerebral blood flow is maintained at a constant level over a range of CPP through the process of autoregulation. When autoregulation is disrupted, such as with TBI, changes in BP or ICP will directly impact cerebral blood flow. If autoregulation remains intact after TBI, changes in ICP or BP may influence blood volume (e.g. dilatation or constriction of blood vessels) and influence ICP. Increases in ICP without a concomitant increase in MAP may lead to a decrease in CPP (Dunn, 2002; Toledo et al., 2008).
A normal adult CPP value is more than 70 mmHg. The threshold value for CPP in adults is 50 mmHg, as the effect of secondary brain injury has a marked influence on patient outcome with sustained CPP levels below 50 mmHg. If CPP is maintained at threshold value, blood flow to the brain becomes inadequate and neural hypoxia and brain death can occur (Morton et al., 2005). It is suggested that a CPP of 60–70 mmHg in a patient with TBI is sufficient to maintain cerebral oxygenation (Shirley, 2009). Other authors have noted that if CPP is kept at 70–80 mmHg, mortality in patients with severe TBI is less than 35% (Guha, 2004). The aim of medical management of an adult patient with TBI is therefore to maintain CPP above 70 mmHg. It is essential that ICP and MAP be maintained within normal limits in order to prevent cerebral ischaemia.
Secondary brain injury is a frequent occurrence in severe paediatric head trauma, leading to raised ICP. All head-injured children with Glasgow coma scale (GCS) less than eight, evidence of intracranial mass lesions or brain contusion, shearing or DAI require ICP monitoring (Weiner and Weinberg, 2000; Kochanek et al., 2012). In children, CPP should generally be maintained above 40 mmHg (Alterman and Geibel, 2011; Kochanek et al., 2012). Age-related critical threshold levels for CPP have been reported by Chambers et al. (2006), who suggest that CPP be maintained above 48 mmHg (ages 2–6), 54 mmHg (ages 7–10) and 58 mmHg (ages 11–15).
9.4. Severity of Injury
Severity of injury is evaluated using GCS. The GCS evaluates consciousness by reviewing eye, verbal and motor responses to stimuli. The eye response gives an understanding of arousal or wakefulness; the verbal response information on alertness and awareness; and motor response reveals the extent of motor activity (Palmer and Knight, 2006; Matis and Birbilis, 2008) (Table 9.4). The maximum GCS score is 15/15 and indicates full consciousness; the minimum score is 3/15, which indicates no response to stimuli. If a person is mechanically ventilated, GCS grading is out of a total of 10, as the verbal response is excluded from scoring due to the presence of an artificial airway.
A severe head injury is graded as a GCS score less than 8/15, moderate injury a score between 9/15 and 12/15 and mild injury between 13/15 and 14/15. A mild injury is commonly diagnosed as a concussion and could be further assessed by evaluating the severity of amnesia. The extended GCS (GCS-E) serves as an additional tool to evaluate and monitor mild injuries (Nell et al., 2000; Matis and Birbilis, 2008). In children less than three years of age, the paediatric GCS should be used to evaluate consciousness due to communication skills not being fully developed in very young children (Table 9.5).
Table 9.4:Glasgow coma scale for adult patients*.
Eye response 4 — spontaneous opening of eyes 3 — opens eyes on response to speech 2 — opens eyes on pain stimulus 1 — no response elicited Verbal response 5 — orientated and converses normally 4 — confused and disorientated 3 — utters inappropriate words 2 — makes inappropriate sounds 1 — makes no sound Motor response 6 — obeys commands 5 — localises to painful stimulus 4 — flexes or withdraws to painful stimulus 3 — abnormal flexing to painful stimulus (decorticate response) 2 — extension to painful stimulus (decerebrate response) 1 — no motor response present |
*Adapted from Palmer and Knight (2006).
Abnormal flexion and extension on the GCS indicates abnormal posturing. Abnormal flexion is noted when a patient exhibits the following movement pattern: adduction of the upper limbs, flexion of the arm, wrist and fingers with extension and internal rotation of the lower limbs and plantar flexion of the feet. This is called decorticate posturing. Abnormal extension is demonstrated as adduction and hyperpronation of the upper limbs with extension of the lower extremities and plantar flexion. The head and neck may also be arched backwards. This is decerebrate posturing (Matis and Birbilis, 2008). Both these abnormal postures indicate loss of higher motor control function and poor outcomes (Palmer and Knight, 2006).
9.5. Medical and Surgical Management
Effective management of the patient with TBI is dependent on interdisciplinary team work. The interdisciplinary team in the acute care setting includes paramedics, medical doctors (trauma surgeon, neurosurgeon, ICU consultant or orthopaedic surgeon), nursing staff and physiotherapists. Additional therapeutic specialists, such as occupational therapists and speech and language therapists, and counsellors are included in the team as the patient’s condition improves and they move into the sub-acute and chronic phases of recovery. Family members and caregivers form part of the team and should be educated regarding their role throughout the patient’s recovery, even if it only includes sensory stimulation of the patient by touch and speech during the acute phase. Lastly, the patient is central to this team once consciousness is regained, and active participation in physical activity can be encouraged in the acute care setting.
Table 9.5:Paediatric Glasgow coma scale.
Eye opening response | ||
≤2 years | Score | >2 years |
Spontaneous | 4 | Spontaneous |
To speech | 4 | To voice |
To pain | 2 | To pain |
None | 1 | None |
Verbal response | ||
≤2 years | Score | >2 years |
Coos and babbles | 5 | Oriented |
Irritable and cries | 4 | Confused |
Cries to pain | 3 | Inappropriate |
Moans to pain | 2 | Incomprehensible |
None | 1 | None |
Motor Response | ||
≤2 years | Score | >2 years |
Normal, spontaneous | 6 | Obeys commands |
Withdraws to touch | 5 | Localises pain |
Withdraws to pain | 4 | Withdraws to pain |
Abnormal flexion | 3 | Flexion to pain |
Abnormal extension | 2 | Extension to pain |
None | 1 | None |
9.5.1. Primary survey and resuscitation of vital functions
The airways, breathing, circulation, disability, exposure (ABCDE) approach to assessment and care provided during the primary survey for patients with suspected TBI consists of a similar approach to that described in the previous chapters of this book (Chapter 5, Section 5.3.1). The establishment of a patent airway as well as assessment for disability using the alert, voice responsive, pain responsive or unresponsive (AVPU) (Chapter 5, Section 5.3.1) approach and GCS are of particular importance during the primary survey of a patient with TBI.
9.5.2. Secondary survey as adjunct to primary survey
The secondary survey of a patient with TBI consists of similar assessment and management approaches as that described in the previous chapters of this book (Chapter 5, Section 5.3.2). Computed tomography scanning or three-dimensional CT (if available) is performed for all patients with TBI to assess the extent of the injury and to identify those in need of immediate surgical intervention (Coles, 2007). Assessment for other injuries is also performed, especially in relation to the spinal cord (Thim et al., 2012).
9.5.3. Definitive care
9.5.3.1. Care provided in the ICU
Monitoring of a patient with TBI forms an integral part of the care provided in the ICU. Monitoring includes routine physiological parameters and also more specific neurological parameters such as ICP, CPP, pupil size and reactivity and assessment for the presence of neurogenic pulmonary oedema. These neurological parameters are concepts that physiotherapists, working with patients with TBI, should know and understand well, as they influence the management of such patients. Critical care management of the patient with TBI also includes prevention of secondary brain insults related to hyponatraemia, hypotension, hypoxaemia, hypercarbia and raised ICP, as well as early diagnosis and treatment of newly developed medical and surgical problems (Mauritz et al., 2007; Timmons, 2012).
The following strategies are implemented to limit secondary brain insults during ICU stay.
•The patient is nursed in a 30º upright (angle of the head on an ICU bed) position with the head in midline. This position facilitates ventilation, reduces ICP by encouraging venous drainage, improves CPP and limits the onset of ventilator-associated pneumonia, as the risk for aspiration is reduced in this position (Meyer et al., 2010; Haddad and Arabi, 2012).
•Compression of the jugular veins with tight tape fixation of the endotracheal tube (ETT) is avoided as this might impede cerebral venous drainage and lead to an increase in ICP (Haddad and Arabi, 2012).
•Sedatives such as morphine and fentanyl are first-line drugs in the management of a patient with TBI in the ICU. Propofol is also used in some trauma centres to reduce ICP. These drugs are particularly important to reduce the effect of manual handling on ICP and CPP during nursing procedures and physiotherapy treatment (Haddad and Arabi, 2012; Mejaddam and Velmahos, 2012).
•Neuromuscular blocking agents (paralysing drugs) may be prescribed as a means to inhibit the cough reflex and induce muscle relaxation in an attempt to control ICP (Guha, 2004).
•The prescription of barbiturates assists in lowering ICP by suppressing cerebral metabolism. This is achieved by lowering cerebral metabolic demands and cerebral blood volume. An adverse result of barbiturates is the subsequent reduction in BP that can cause a fall in CPP. Close monitoring of cardiovascular status of the adult and paediatric patient with TBI is therefore required during administration of barbiturates (Bullock and Povlishock, 2007; Roberts and Sydenham, 2009; Kochanek et al., 2012).
•The use of corticosteroids to reduce ICP is associated with high mortality in both adult and paediatric patients with TBI and is not recommended (Bullock and Povlishock, 2007; Kochaneck et al., 2012; Rosenfeld et al., 2012).
•Anti-seizure prophylaxis is given, using phenytoin in both adult and paediatric patients to reduce the number of early posttraumatic seizure episodes. It is recommended that phenytoin be used only during the first seven days after TBI in order to prevent the onset of its side-effects (Bullock and Povlishock, 2007; Kochaneck et al., 2012; Mejaddam and Velmahos, 2012).
•An external ventricular drain (EVD) may be positioned to drain excess CSF (Meyer et al., 2010) (Figs 9.6A and B).
•The patient is mechanically ventilated to ensure adequate oxygenation and reduce metabolic demand. Hyperventilation (partial pressure of carbon dioxide in arterial blood (PaCO2) 30–35 mmHg (3.9–4.6 kPa)) is employed for only brief periods to manage episodes of acute neurological deterioration in order to reduce ICP by creating cerebral vasoconstriction and reduce cerebral blood flow. The use of hyperventilation in the first 24 hours after admission is, however, not advocated due to the reduction in cerebral blood flow in brain tissue that might already be close to ischaemic threshold (Bullock and Povlishock, 2007; Robert and Schierhout, 2009). Adequate ventilation should ensure arterial blood gas values as follows: PaCO2 of 35–40 mmHg (4.7–5 kPa), partial pressure of oxygen in arterial blood (PaO2) greater than 80 mmHg (10 kPa) and oxygen saturation equal to or greater than 95% (Arabi et al., 2010). As with the adult, prolonged hyperventilation should be avoided in the head-injured child to prevent secondary ischaemic brain injury. Hyperoxia should be avoided, as this has been associated with poor outcome in adult and paediatric studies (Kochanek et al., 2012).
Fig. 9.6B:Fixed CT scan image of a nine-year-old girl with severe head injury and EVD after a motor vehicle accident.
•Early tracheostomy is advocated for adult patients with TBI in order to reduce the number of mechanical ventilation (MV) days (Bullock and Povlishock, 2007).
•Body temperature is controlled to a mild to moderate hypothermic state for up to 48 hours to lessen secondary brain damage (Peterson et al., 2008; Meyer et al., 2010). This appears to be especially efficacious with young adults (younger than 45 years), but results from paediatric trials showed no benefits (De Deyne, 2010; Alterman and Geibel, 2011).
•Prophylaxis for deep venous thrombosis in adult patients with TBI is achieved with the use of graduated compression stockings and low molecular weight heparin (Bullock and Povlishock, 2007).
•Patients who present with neurological deterioration more than 24 hours after admission undergo repeat CT scanning in order to identify the cause for the deterioration (Brown et al., 2007; Kochanek et al., 2012).
In addition to the strategies outlined above, special attention is given to the monitoring of pupil size and reactivity and the development of neurogenic pulmonary oedema.
9.5.3.1.1. Pupil size and reactivity
Evaluation of pupil size and reactivity provides information regarding the integrity of the third cranial nerve and thus brain stem function. The size of the pupils is observed as well as their reaction to light. Pupil size is measured in millimetres and reactivity in terms of ‘brisk’, ‘sluggish’ or ‘fixed’ by observing response to light (Morton et al., 2005). If assessment indicates a normal response, the following acronyms are often used in ICU documentation: PEARL (pupils equal and reactive to light) or PERL (pupils equal reactive to light). Poor prognosis is reflected by fixed and dilated pupils. Examples of and explanations for abnormal pupil presentations in patients with TBI are summarised in Table 9.6.
Table 9.6:Abnormal pupil presentation in a patient with TBI*.
Pupillary presentation | Possible explanations |
Pinpoint pupils | •Medication such as opiates •Eye drops for glaucoma •Small reactive pupils indicative of metabolic abnormalities •Small reactive pupils indicating bilateral dysfunction of the diencephalon •Damage to the pons if pupils non-reactive |
Dilated pupils | •Seizures •Sedative drugs such as thiopentone •Recreational drugs such as cocaine •Fear and panic •Unilateral non-reactive dilated pupil (‘blown pupil’) indicative of third cranial nerve damage following herniation of the uncal portion of the temporal lobe •Bilateral fixed dilated non-reactive pupils due to brain herniation |
*(Morton et al., 2005; Paz and West, 2009).
9.5.3.1.2. Neurogenic pulmonary oedema
Neurogenic pulmonary oedema is a clinical syndrome that develops following a significant central nervous system insult, such as TBI, and presents as acute pulmonary oedema. Patients with TBI are therefore at risk of developing neurogenic pulmonary oedema. Individuals in whom the neurological insult occurred in an abrupt and rapid manner, resulting in a sudden increase in ICP, appear to be at the greatest risk of developing neurogenic pulmonary oedema. Autonomic response to the rapid rise in ICP results in a release of catecholamines, leading to blood volume shift from the systemic circulation to the pulmonary circulation. Increased pressure in the pulmonary circulation results in pulmonary oedema. It is also suggested that, during the above process, barotrauma occurs at the alveolar-capillary membrane, which results in persistent vascular leaks (Davison et al., 2012). The early form of neurogenic pulmonary oedema develops within minutes to hours after injury; the delayed form develops within 12 to 24 hours following injury.
Clinical signs and symptoms of neurogenic pulmonary oedema are similar to other causes of pulmonary oedema and include dyspnoea, tachypnoea, hypoxia, cough productive of pink frothy liquid (not sputum) and gravity-dependent bilateral crackles on auscultation. Chest x-ray features include bilateral hyperdense infiltrates widespread over both lung fields, similar to that seen in acute respiratory distress syndrome. Signs and symptoms often resolve spontaneously, but in patients with severe TBI and elevated ICP, neurogenic pulmonary oedema may persist (Davison et al., 2012).
9.5.3.2. Surgical interventions
Surgical intervention is considered in cases in which conservative management alone is not effective in controlling ICP (e.g. ICP sustained at values greater than 25 mmHg). It may also be indicated when neurological deterioration is detected in spite of optimal conservative management of the patient. Craniotomy or decompression craniectomy may be performed for adult or paediatric patients under these conditions in order to prevent further secondary brain injury.
9.5.3.2.1. Craniotomy
Craniotomy is a cut that opens the skull. A bone flap (section of the skull) is removed to allow the neurosurgeon access to the dura and the brain underneath. Craniotomy can be small-sized (coin-sized) or large. Small-sized craniotomy is called burr hole or keyhole surgery and is performed for the insertion of an ICP monitor or ventricular drain. Large-sized craniotomy is called skull base surgery and is usually performed after penetrating trauma (gunshot or stab injury) to the head that resulted in a skull fracture and brain injury. After the procedure the bone flap is replaced and secured to the skull with small plates and screws (Warnick, 2013).
9.5.3.2.2. Decompression craniectomy
Decompression craniectomy involves the removal of a segment of the skull bone to allow the swelling brain to expand without compression. It is a last resort therapy to prevent herniation of the brain. The skull bone is only replaced several days later, when brain swelling has subsided. It is recommended that decompression craniectomy is performed in the early stages of the patient’s management in order for the patient to derive optimal benefits from this procedure (Kochanek et al., 2012). Decompression craniectomy may lead to better control of ICP, but results from studies investigating the long-term outcomes in patients who underwent this procedure are discouraging: neurologic function is reported to be more affected in these patients at six months after discharge than in those who didn’t undergo decompression craniectomy (Mejaddam and Velmahos, 2012; Rosenfeld et al., 2012). The randomised evaluation of surgery with craniectomy for uncontrollable elevation in intracranial pressure (RESCUEicp) trial is a multicentre trial currently investigating whether decompression craniectomy is effective in the management of uncontrollable ICP. Results from this trial are awaited (Hutchinson et al., 2011).
After either of the abovementioned surgical procedures, the patient will be returned to the ICU for monitoring and care.
9.5.3.3. Brain stem death
Brain stem death is a clinical syndrome, whereby reflexes that have pathways through the brain stem cease to function. Death of the patient is therefore certified when brain stem death is confirmed, as the patient would have permanently lost consciousness as well as the ability to breathe spontaneously (Wijdicks et al., 2010). A multi-centre study in Canada found that brain herniation was the most common cause for withdrawal of life-saving treatment for patients involved in MVAs with severe TBI (Cote et al., 2013).
The determination of brain stem death is done in some countries by a neurologist or neurosurgeon and in other countries by two doctors who are registered with the Health Professions Council of that specific country but who may not necessarily specialise in neurology. There are certain prerequisites that need to be in place before brain stem testing can be conducted, and these are summarised in Table 9.7.
The neurologic assessment for brain stem death consists of four steps (Wijdicks et al., 2010):
•assessment for coma (patient must lack all evidence of responsiveness; thus no eye or motor responses to painful stimuli);
•assessment for absence of brain stem reflexes (Table 9.8);
Table 9.7:Prerequisites for brain stem testing*.
•Determination of the cause of coma
•Patient’s core body temperature must be within normal limits •Patient’s SBP must be within normal limits |
•apnoea test (Table 9.8); and
•confirmation of the irreversibility of these findings by repeated measure.
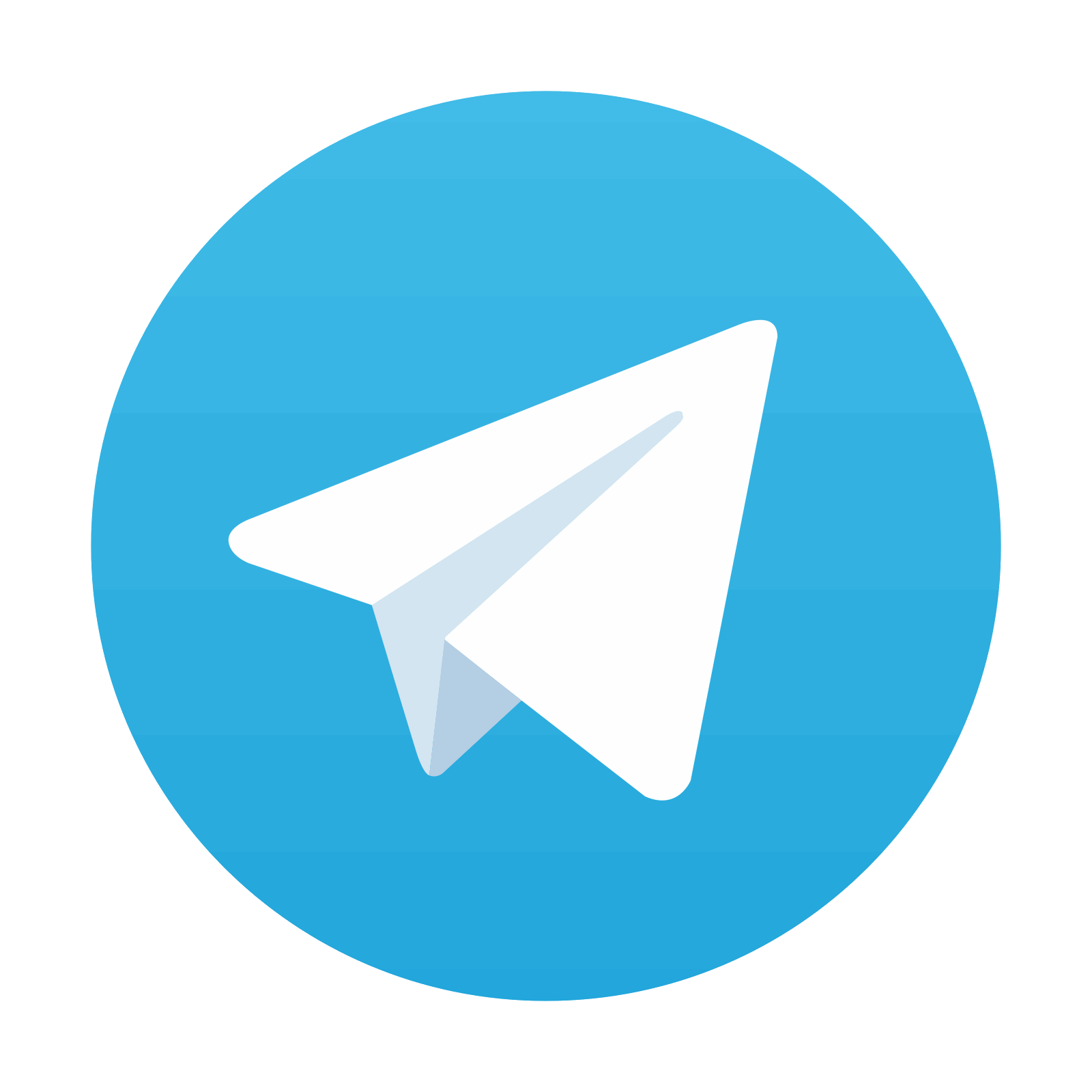
Stay updated, free articles. Join our Telegram channel

Full access? Get Clinical Tree
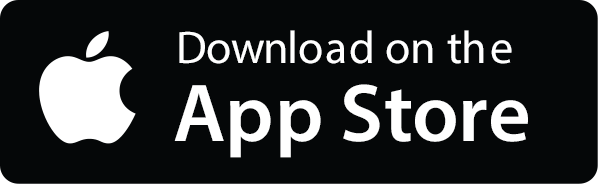
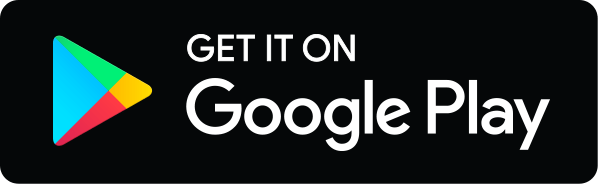