About half of vertebral fractures and substantial ligamentous injuries of the spine in children occur in the cervical region. However, serious cervical spine injuries in children are rare. This relates in part to the resilient nature of ligaments and bones in children. In addition, young children are infrequently exposed to high-force injury mechanisms. In infants and young children, child abuse and birth trauma are the most common mechanisms of cervical spine trauma. Birth-related injuries of the spine and spinal cord most often are associated with a traumatic breech delivery. These injuries are usually in the lower cervical or upper thoracic region, and often result from traction forces. Spine injuries suffered during cephalic delivery tend to occur in the upper cervical region, and typically involve rotational forces. In older children and teenagers with spine injuries, common mechanisms include diving, sports injuries, and motor vehicle incidents. The prevalence of pediatric cervical spine injuries increases with age. In older patients, the mid and inferior aspects of the cervical spine are most commonly involved, whereas infants and young children tend to suffer injuries at the C3 level or higher. Because the pediatric spine has greater elasticity than the spinal cord (which is fixed by nerve roots), trauma-related neurological injuries can occur in the absence of fractures or malalignment.1–3
Cervical spine injuries range from mild ligamentous injuries to severe fracture-dislocations, with or without spinal cord involvement. The 5 major mechanisms consist of flexion, extension, lateral flexion, rotation, and axial compression. With excessive flexion, compressive force is applied to the anterior aspect of the vertebra, and distraction force posteriorly; this can lead to an anterior vertebral body fracture and posterior ligamentous disruption. Extension injuries subject the vertebrae to compressive forces dorsally and distracting forces anteriorly; fractures of the articular facets, pillars, and posterior elements may occur, as well as vertebral body avulsion injuries. Lateral flexion mechanisms can lead to vertebral compression injuries, fractures of the transverse or uncinate processes, and contralateral avulsion of the brachial plexus. Rotation injuries can cause locked facet, rotatory subluxation, or rotatory fixation; there are usually concomitant flexion or extension injuries. Burst-type fractures can result from axial compression forces.
The most common cervical spine fractures in children occur in the vertebral bodies; more than half involve the C6 or C7 vertebral bodies. Neural arch fractures of the cervical spine are uncommon in children. The most common site is the articular pillar, especially at the C6 level. These fractures are difficult to detect on standard radiographs; CT is diagnostic. Other neural arch fractures are less common. Laminar fractures tend to occur at the C5 and C6 levels. Most spinous process fractures involve C6 or C7. Fractures of the pedicles tend to occur at the C2 level. Transverse process fractures are rare in the cervical spine; the C7 level is the most frequent site of this injury.
Approximately 5% to 10% of pediatric cervical spine fractures involve the dens, usually at the base. An additional 5% to 10% of fractures involve the body or neural arch of the axis. Fractures of the atlas account for approximately 5% of pediatric cervical spine fractures; these are usually burst or focal arch fractures. The upper cervical spine can also be involved with atlantoaxial dislocation or atlantooccipital dislocation injuries.
Selection of the optimal diagnostic imaging approach to the child with suspected cervical spine trauma requires consideration of the specific situation. These concepts are reviewed in the American College of Radiology Appropriateness Criteria (www.acr.org/ac). For adults and most older children with acute cervical spine trauma, helical CT is the procedure of choice. In many institutions, a three view radiographic examination is the usual evaluation method for young children (AP, lateral, and open-mouth frontal views); CT is reserved for those patients who require additional characterization of a known fracture and those for whom there is a high clinical suspicion of a radiographically occult injury. MR is sensitive for demonstrating marrow edema due to a vertebral injury and for detecting ligamentous injuries. MR is the technique of choice for patients with suspected cord injury, nerve root injury, or intraspinal hemorrhage. Flexion and extension lateral radiographs and/or fluoroscopy are of benefit for selected patients to detect ligamentous injuries, although MR has greater sensitivity for this indication. Flexion and extension radiographs should only be performed if the patient is responsive and able to report pain during positioning maneuvers.
The normal cervical spine assumes a mild lordotic curve in the neutral position, as viewed on a lateral radiograph. The cervical spine should be straight on the frontal view. Deviation from this appearance raises the possibility of muscle spasm or osseous or ligamentous injury. On the lateral projection, neck muscle spasm leads to straightening of the cervical spine or mild kyphosis. Unlike adults and teenagers, however, isolated lack of lordosis is common in normal children. The apex of spasm-related kyphosis is usually located at the C2-C3 level. Localized kyphosis at other levels may be an indicator of dorsal ligamentous injury. On the frontal projection, muscle spasm leads to scoliosis or torticollis.
Proper evaluation of a lateral neck radiograph of the child who has suffered trauma requires careful assessment of the paravertebral soft tissues. On a properly positioned lateral neck radiograph without swallowing artifact, the retropharyngeal space (posterior pharyngeal wall at C2-C3 to the anterior margin of the vertebra) should be 7 mm or less and the upper retrotracheal space (distance between the trachea and the vertebra at C4-C5) should be 14 mm or less. The prevertebral soft tissues at the C6-C7 level should be 21 mm or less. As a rule, the width of the normal prevertebral soft tissue space below the level of the glottis is less than twice the anterior-posterior diameter of the adjacent vertebral body. The width of the normal retropharyngeal space is less than the anteroposterior diameter of the second cervical vertebra.
Prevertebral soft tissue widening can indicate the presence of hemorrhage or edema, and therefore is an important indicator of possible osseous or ligamentous injury of the cervical spine. However, this finding is often lacking in the presence of a mild compression fracture or a nondisplaced fracture of the dens. The most common cause of prevertebral soft tissue thickening is artifact due to swallowing or neck flexion. Findings that suggest patient swallowing during image acquisition include air in the esophagus, distortion of the hypopharynx, and elevation of the glottis. Ancillary findings that are sometimes helpful for identifying changes due to a true cervical spine injury include anterior displacement of the airway and anterior displacement of the prevertebral fat stripe.
Widening of the predental space (atlas-dens interval) is an important radiographic indicator of potential ligamentous or osseous injuries in this region. This is defined as the distance from the anterior aspect of the dens to the posterior-inferior margin of the anterior arch of the atlas. In adults, the predental space measures 2.5 mm or less. However, the measured distance on a lateral radiograph is up to 4.5 mm in normal children. On CT, the predental space should measure less than 2.6 mm. Variations of up to 3 mm can occur between flexion and extension in children. In general, pathological widening of the predental space suggests disruption of the transverse ligament that bridges C1 and the dens. Disruption of this ligament is uncommon, however, even with a fracture of the dens; most instances of predental space widening are artifactual or related to chronic abnormalities such as Down syndrome, rheumatoid arthritis, or dens hypoplasia.4
Abnormal vertebral body alignment as viewed on a lateral cervical spine radiograph can occur in the presence of a fracture or ligamentous injury. Most often, there is anterior displacement of a vertebral body with respect to the next most inferior vertebra. This type of malalignment is usually associated with a flexion injury or, less commonly, a rotatory or extension injury. Posterior displacement of a cervical vertebral body with respect to the subjacent vertebra occasionally occurs during the acute phase of an extension injury. Lateral vertebral body displacement can occur in the presence of a fracture-dislocation.
The appearance of anterior vertebral body displacement in the upper portion of the pediatric cervical spine must be interpreted with caution, because mild displacement occurs as a normal finding. This physiologic displacement can involve the upper 4 vertebral bodies or C2 on C3 only. The physiologic nature of the displacement is obvious when multiple vertebral bodies are involved. Isolated anterior displacement of C2 on C3 is more easily confused with true pathology such as a neural arch fracture of C2. Assessment of the posterior element alignment and the prevertebral soft tissues is helpful in differentiating physiological versus pathological localized cervical vertebral body displacement.
Assessment of the posterior cervical line is often useful for evaluating apparent localized anterior subluxation of C2 on C3. This line extends from the anterior aspect of the cortex of the spinous process of C1 to the same point on C3 (see Chapter 22). With physiologic subluxation, the anterior cortex of the C2 spinous process is within 2 mm of the posterior cervical line. Injuries that cause excessive deviation of the C2 spinous process from the posterior cervical line include odontoid fractures, atlantoaxial subluxation due to a transverse atlantal ligament disruption, C2 neural arch fractures, and C2-C3 dislocation (Figure 25-1).
Figure 25–1
Type II odontoid fracture.
This 4-year-old child suffered a hyperflexion injury when he was struck in the head by a truck tailgate. A. A lateral radiograph shows anterior displacement of the odontoid process and widening of the synchondrosis. The C2 spinous process projects posterior to the posterior cervical line due to anterior displacement of C1. There is prevertebral soft tissue prominence (arrows). B. A sagittal CT image confirms a fracture through the synchondrosis of the odontoid. There are small fracture fragments adjacent the base of the displaced odontoid.

Alterations in disc space height can occasionally occur with cervical spine trauma. Disc space narrowing usually is related to a flexion or rotation injury, whereas pathologic disc space widening is associated with an extension injury. Normally, the intravertebral disc spaces are of equal height throughout the cervical spine. Disc space widening or narrowing is best appreciated on a properly positioned lateral radiograph or a reformatted CT image. MR may show signal abnormality within the injured disc.
The lateral cervical spine radiograph of a child who has experienced trauma should be carefully evaluated for alignment of the apophyseal joints. An abrupt discrepancy in apophyseal joint alignment is suggestive of a locked facet due to rotatory subluxation. The involved apophyseal joint may also appear widened or narrowed. Apophyseal joint dislocation is usually related to a flexion mechanism.
Alignment of the spinous processes should be carefully evaluated on both the frontal and lateral cervical spine views. Posterior spinal ligament disruption with a hyperflexion injury typically leads to widening of the interspinous distance at the involved site. The widening increases on flexion views. It is important to recognize that there is substantial variation in the interspinous distance at C1-C2 in normal individuals. Focal deviation of spinous process alignment on the frontal view can indicate the presence of an underlying vertebral arch fracture or unilateral locked facet. With unilateral locked facet, the superior spinous process is displaced toward the side of locking.
Atlantooccipital dislocation (occipitoatlantal dissociation) is a rare injury that is due to stretching or disruption of the tectorial membrane and the alar ligaments. This injury is usually associated with a deceleration mechanism in which the head moves forward and the torso is restrained. Atlantooccipital dislocation is usually fatal, due to associated damage to the brainstem and cervical spinal cord. Vascular injuries and damage to cranial nerves and upper cervical spinal nerves can also occur. Infants and young children are relatively susceptible to this injury due to their small occipital condyles and horizontally oriented atlantooccipital joints.
Radiographs of patients with atlantooccipital dislocation show malalignment and widening at the craniocervical junction (Figure 25-2). Because there is usually no associated fracture, the imaging diagnosis requires visualization of malalignment. In some instances, the findings on standard radiographs are subtle. Sagittal and coronal reformatted CT images best demonstrate the pathological anatomy. Occasionally, there is an associated occipital condyle fracture. Complete dislocation is more common than partial. Dislocation is further classified as anterior, posterior (rare), and purely distracted forms. The most common pattern is concomitant anterior and distracted.
On lateral cervical spine radiographs, various measurements can be utilized to evaluate atlantooccipital alignment. (1) The distance between the occipital condyles and the condylar surfaces of the atlas should be 5 mm or less (<2.5 mm on CT). (2) The distance between the basion and the tip of the odontoid process (basion-dens interval) should be 12 mm or less. (3) A line drawn along the posterior aspect of the dens should pass within 12 mm of the basion. (4) Malalignment is suggested if a line (the “Wachenheim clivus line”) drawn along the posterior aspect of the clivus does not intersect, or is tangential to, the odontoid process. (5) The “Powers ratio” (BC/OA ratio) should be less than 1 (<0.9 on CT). This is the distance from the basion (the inferior tip of the clivus) to the inner margin of the posterior arch of the atlas divided by the distance from the inner margin of the anterior arch of the atlas to the posterior lip of the foramen magnum. The Powers ratio is useful for detecting anterior atlantooccipital dislocation.4
Fractures can occur in any part of the atlas, but the most common pattern is bilateral vertical fractures though the neural arch. Disruption of the apophyses of the anterior arch can occur in young children. A Jefferson fracture is a burst injury of the atlas, with fractures of the anterior and posterior arches, a tear of the transverse ligament, and centripetal displacement of the fragments. Most commonly, there are 3 fractures of the atlas, one in each posterior hemiarch and one in the anterior hemiarch. This combination of injuries results in widening of the ring of the atlas and separation of the lateral masses. The Jefferson fracture is usually due to an axial load applied to the vertex. Spinal cord compression is rare with this injury.
On frontal radiographs, a Jefferson fracture is indicated by bilateral spreading of the lateral articular masses of C1 with respect to the corresponding articular surfaces of C2. This is best demonstrated on the open-mouth view. If an open-mouth view cannot be obtained, adequate visualization is often possible on a frontal radiograph with the neck extended. Optimal characterization of the fracture is with CT. In young children, fractures sometimes occur through the synchondroses.
The atlas forms from 3 primary ossification centers. Prior to fusion (anteriorly at approximately 7 years of age and posteriorly at approximately 3 to 5 years), the radiolucent cartilage between the ossification centers should not be mistaken for a fracture. Abnormal widening or other malalignment suggests a fracture through the cartilage. In normal infants and young children, the lateral articular masses of C1 often project slightly beyond the margins of C2; this is termed “pseudospread” of C1 on C2. Up to 6 mm of overlap is considered normal in children up to 7 years of age. Spondyloschisis is a developmental cleft in C1 that can produce mild widening of C1 with respect to C2.
Odontoid fractures result from flexion or extension injuries. Odontoid fractures can be classified into 3 types. The type I fracture is rare, and involves the tip of the odontoid. The Type II fracture involves the base of the dens. The type III fracture extends into the body of the vertebra. Type II and type III injuries occur with approximately equal frequencies. All 3 forms of odontoid fractures can be complicated by subluxation and spinal cord involvement. Type I and type III fractures tend to heal satisfactorily with conservative therapy. The type II injury carries the potential for nonunion and chronic instability.
The normal synchondrosis at the base of the dens in young children is visible radiographically as a linear radiolucency; remnants of this structure sometimes persist in adolescents and adults. Widening of the synchondrosis or malalignment between the dens and the body of C2 are the important radiographic manifestations of pathology. Anterior tilting of the dens nearly always indicates the presence of a fracture; slight posterior tilting is a normal finding, however. Local soft tissue swelling also is a suggestive finding of a fracture. In some instances, a nondisplaced fracture through the synchondrosis in an infant or young child is radiographically occult. Carefully performed flexion imaging under fluoroscopic observation can be helpful (Figure 25-3). MR may demonstrate edema at the fracture site and serves to confirm paraspinal soft tissue edema. Followup radiographs show bony resorption at the margins of the synchondrosis.
Figure 25–3
Type II odontoid fracture.
A. There is no visible fracture or vertebral malalignment on the lateral view obtained in neutral position. Marked prevertebral soft tissue prominence suggests a significant injury, however. B. A spot film image obtained during neck flexion shows anterior tilting of the odontoid and malalignment between the neural arches of C1 and C2.

Fractures of the body of C2 are less common than those involving the dens. A hyperextension teardrop fracture of the C2 body can occur due to violent sudden extension of the neck. The anterior longitudinal ligament avulses a wedge-shaped piece of bone at its insertion site along the anteroinferior aspect of the body of the axis. An obliquely oriented fracture through the body of C2 can be associated with anterior or posterior displacement of fracture fragments. Although the actual fracture line may not be visible on the lateral radiograph, there is apparent increase in the distance between the anterior and posterior margins of the vertebral body: the “fat C2” sign. This is a potentially unstable injury, and when standard radiographs suggest this finding, CT should be performed.
Fractures of the neural arch of C2 are nearly always bilateral, but are often asymmetrical (Figure 25-4). Bilateral pars interarticularis fractures of C2 or fractures of the adjacent portions of the articular facets can lead to traumatic spondylolisthesis, with anterior displacement of the body of the axis (Figure 25-5). This injury has historically been termed the “hangman fracture.” Use of this term is now discouraged because the usual mechanism of traumatic spondylolisthesis is hyperextension or flexion in combination with axial loading, whereas hanging involves violent distraction (rather than axial loading) in combination with hyperextension. In addition, cervical spine fractures are rare with suicidal hangings (asphyxiation is the usual cause of death). Although traumatic spondylolisthesis of C2 is an unstable injury, the capacious nature of the subarachnoid space in the upper cervical spine helps avoid cord injury, unless there is substantial displacement. A common mechanism for this injury involves an unrestrained occupant in a frontal motor vehicle collision, with forceful impact of the forehead against the windshield.
Figure 25–5
Neural arch fractures of C2.
A lateral radiograph of a child involved in a motor vehicle collision demonstrates bilateral C2 pars interarticularis fractures. There is traumatic spondylolisthesis, with anterior displacement of C1 and the body of C2 relative to the remainder of the cervical spine.

Atlantoaxial rotatory fixation (atlantoaxial rotatory subluxation) involves locking of the anterior facet of C1 on the facet of C2. If there is anterior displacement of the lateral mass of C1 relative to C2 in combination with widening of the predental space, atlantoaxial rotatory dislocation is present. Muscular torticollis causes a benign form of rotatory fixation that usually resolves in response to conservative therapy. The more severe forms require bracing or traction. Recalcitrant malalignment occasionally requires surgical fusion.
Patients with atlantoaxial rotatory fixation have torticollis and severe limitation in the ability to rotate the neck. The head is usually flexed laterally on the side opposite to the rotated chin, producing a “cock robin” appearance. Attempts by caregivers to rotate the neck in a direction to correct the deformity often exacerbate pain. Most cases are due to trauma, but it can also occur spontaneously with no known injury. Occasionally, there is an underlying congenital vertebral abnormality or other preexisting condition (e.g., juvenile rheumatoid arthritis or Down syndrome). Grisel syndrome refers to atlantoaxial rotatory subluxation/dislocation in association with head and neck infection, apparently caused by infectious laxity of the transverse ligament.
The Fielding Classification divides atlantoaxial rotatory fixation and dislocation into 4 categories, based on the degree of displacement of C1 with respect to C2.5 Type I involves no displacement of C1, and is the most common type (Figure 25-6). With type II, there is 3 to 5 mm of anterior displacement of C1; this indicates abnormality of the transverse ligament. With type III, there is greater than 5 mm of anterior displacement of C1 on C2; this is associated with deficiency of the transverse and alar ligaments. With type IV subluxation, C1 is displaced posteriorly with respect to C2; this is the least common type.
Figure 25–6
Atlantoaxial rotatory fixation; Fielding type I.
A. A CT scout image of a 15-year-old boy with torticollis shows rotation of the head to the left. B. An axial CT at the level of the atlas shows approximiately 35° rotation of the head and the atlas relative to the tabletop. There is no separation between the odontoid and the anterior aspect of C1. C. A more inferior image shows C2 to be in neutral position.


The Pang classification recognizes 3 types of atlantoaxial rotatory fixation based on the findings on axial CT image sets with the head and neck in the presenting position, with the head turned into the neutral position, and with the head turned to the side opposite to the presenting position. With type I, there is no significant correction during corrective counterrotation. Type II refers to those patients with reduction of the C1-C2 separation angle during forced correction, but C1 does not cross C2. With type III, C1-C2 crossover occurs with forced correction. Pang classifies those patients with dynamics between normal and type III as belonging to a “diagnostic gray zone.” Individuals with muscular torticollis have normal motion in response to extrinsic head positioning.6
With simple atlantoaxial rotatory fixation, radiographs show rotation of C1 on C2, without widening of the atlantodens interval. On lateral radiographs, there is malalignment of the atlas relative to the remainder of the cervical spine. One lateral mass of C1 rotates forward and appears wider and closer to the midline, whereas the other is narrower and farther away from the midline. With atlantoaxial rotatory dislocation, there is anterior displacement of the lateral mass of the atlas relative to the axis, and the atlantodens interval is widened. The pathological anatomy is more accurately demonstrated with CT. As described above, the presence of fixation can be documented by comparing images obtained with the patient in neutral position to those obtained during contralateral rotation of the neck and head.7,8
Acute ligamentous injury at the atlantoaxial or atlantooccipital articulations (i.e., atlantoaxial or atlantooccipital joint disruption) is rare in healthy children, requiring a relatively high-force injury mechanism. Imaging of an atlantoaxial joint disruption may show an associated fracture of the odontoid or small-avulsed fracture fragments (Figure 25-7). Acute atlantoaxial instability can also occur due to retropharyngeal infection (Grisel syndrome) and rarely with adenoidectomy. Chronic atlantoaxial instability is relatively common in patients with Down syndrome, juvenile rheumatoid arthritis, and a variety of developmental bone abnormalities such as Klippel-Feil syndrome, chondrodysplasia punctata, Morquio syndrome, metatropic dysplasia, and some forms of spondyloepiphyseal dysplasia. Patients with os odontoideum are at increased risk for atlantoaxial malalignment. Despite the presence of a predisposing congenital lesion in many patients, the clinical presentation typically is not until mid-childhood.
Figure 25–7
Acute atlantoaxial joint disruption.
A. A sagittal reformatted CT image shows widening of the atlas-dens interval and abnormal separation of the dens from the basion. There are small avulsed fracture fragments anterior to the dens, as well as a nondisplaced fracture of the superior portion of the dens. B. A sagittal T2-weighted image from an MR examination performed 2 weeks later demonstrates focal narrowing of the spinal canal. There is residual hyperintense edema between the dens and the anterior arch of C1.

Instability at the atlantoaxial or atlantooccipital joints carries the potential for neurological deficits due to spinal cord compression. In normal individuals, up to 25° of flexion and extension motion occur at the atlantooccipital joint, but there is only minimal axial or lateral bending motion. At the atlantoaxial joint, however, up to 40° of axial rotation occur in each direction. Up to 20° of flexion/extension motion can occur at the atlantoaxial joint. The major supporting structures at the occipital-atlantal level include the occipital condyles and superior articular surfaces of the ring of C1, the capsular ligaments, the atlantooccipital membranes, and the tectorial membrane. Stability at the atlantoaxial joint is provided primarily by the odontoid process and the transverse ligament.9,10
The most common cause of atlantoaxial instability is Down syndrome, in which the abnormality predominantly relates to ligamentous laxity. Osseous abnormalities may also play a role in these children. The spectrum of potential osseous abnormalities of the craniocervical junction in Down syndrome patients include C1 hypoplasia or assimilation, flattening of the atlantooccipital joint, odontoid process hypoplasia, and os odontoideum. Instability can also occur at the atlantooccipital joint in children with Down syndrome. Children without an underlying genetic disorder or skeletal dysplasia can also have localized congenital anomalies at the craniocervical junction that predispose to atlantoaxial instability; for example, hypoplasia of the dens, absence of the anterior arch of C1, occipitalization of the atlas, basilar invagination, asymmetrical occipitoatlantoaxial facet joints, or C2-C3 fusion.
Clinically significant atlantoaxial instability occurs in 10% to 30% of patients with Down syndrome.10 These patients are at increased risk for atlantoaxial subluxation during athletic events or anesthesia induction for surgical procedures. The Special Olympics mandated in 1983 that radiographic screening be performed on all Down syndrome patients before participating in “high risk” sports; this policy was supported by the American Academy of Pediatrics in 1984. In 1995, the Committee on Sports Medicine and Fitness of the American Academy of Pediatrics published a position paper on atlantoaxial instability in children with Down syndrome in which the 1984 policy was retired. The 1995 statement includes several arguments that disfavor screening of children with Down syndrome for atlantoaxial instability.11 Screening examinations are still advocated by many physicians, however.12 Radiographs can reliably detect most craniocervical junction abnormalities in children with Down syndrome; however, even those children with normal radiographs can develop symptoms acutely after trauma or anesthesia.13
A variety of imaging measurements are available for the detection and grading of atlantoaxial instability. These are most often based on flexion and extension radiographs; however, CT provides more accurate measurements and greater sensitivity for other vertebral deformities. In selected instances, MRI allows precise measurement of the dimensions of the thecal sac and subarachnoid space, and demonstrates any cord impingement. The distance between the posterior surface of the anterior arch of C1 and the anterior surface of the dens is the atlas-dens interval (ADI). The neural canal width (NCW) is the distance between the posterior surface of the dens and the anterior surface of the posterior arch of C1 as measured on a lateral radiograph; the neural canal width can be measured directly on sagittal MR images. For determination of the clivus-posterior odontoid process distance, a vertical line is drawn along the posterior margin of the dens, and the distance from the anterior lip of the foramen magnum to the point at which this line crosses the plane of the foramen magnum is measured. The subarachnoid space width is determined on MR by subtracting the width of the spinal cord from the width of the neural canal.
The most commonly utilized standard for significant atlantoaxial widening as measured by the ADI is greater than 4.5 mm. Atlantoaxial motion on flexion-extension radiographs should be less than 3.5 mm. The lower limits of normal neural canal widths based on patient age and sex are reported in (Table 25-1).14,15 The ADI and clivus-posterior odontoid process distance correlate poorly with the subarachnoid space width, whereas the NCW has a better correlation. Patients with an abnormal NCW or an ADI of greater than 4.5 mm should be evaluated with an MRI study that includes sagittal flexion and extension views (Figure 25-8).15
Figure 25–8
Atlantoaxial instability.
A. A lateral radiograph of an asymptomatic 14-year-old girl shows marked malalignment between C1 (arrows) and C2. B. A sagittal CT image demonstrates widening of the atlas-dens interval, narrowing of the neural canal width (the distance between the dens and the posterior arch of C1 [arrow]), and superior extension of the odontoid process such that it nearly contacts the clivus. C. An axial CT image at the level of C1 shows abnormal positioning of the dens in the central aspect of the spinal canal. D. A sagittal T2-weighted MR image obtained with the neck in flexion confirms marked narrowing of the neural canal at the C1 level. There is impingement upon the cervical spinal cord.

The surgical treatment for significant atlantoaxial instability is an upper cervical arthrodesis. Complications are common with this procedure, and radiological evaluation is important for their detection and characterization. Potential complications include nonunion, loss of reduction, neurological deterioration, late subaxial instability, infection, and wound dehiscence.16
Vertebral body anterior cortical buckle fractures, compression fractures and teardrop fractures are relatively common in the mid and inferior aspects of the cervical spine. These fractures are due to severe hyperflexion of the neck, and are common diving injuries. Occasionally, there is more extensive fragmentation of the vertebral body, with the potential for extrusion of fracture fragments into the spinal canal. The burst fracture is a comminuted vertebral body fracture with a retropulsed fragment into the spinal canal (Figure 25-9). This results from axial compression that drives the disc into the vertebral body. A compressive force occasionally leads to a vertical split fracture of the vertebral body, often accompanied by fractures of the neural arch (Figure 25-10). The teardrop fracture is a fracture-dislocation, with a triangular or quadrangular fragment along the anterior-inferior vertebral body margin and posterior offset or frank dislocation of the affected vertebral body relative to the adjacent vertebra (Figure 25-11). By definition, the hyperflexion teardrop injury is unstable.
Figure 25–9
Burst fracture.
A. A lateral radiograph of a 15-year-old girl following a diving injury shows a comminuted fracture of the C7 vertebral body (arrow). B. A sagittal CT image demonstrates displacement of a fracture fragment into the spinal canal. C. The spinal cord is normal (T2-weighted MR image). The C6-C7 disc is expanded into the fractured C7 vertebral body.


Figure 25–11
Teardrop fracture.
A. A lateral radiograph of a 15-year-old boy following a flexion injury of the neck during a football game shows a fracture of the C4 vertebral body. Two triangular fragments are present anteriorly. The posterior aspect of the body is intact. There is slight posterior offset of C4 relative to C5 and minimal widening of the interspinous space. B. A sagittal reformatted CT image confirms the compression injury of the anterior aspect of the vertebral body and slight malalignment.

In children, radiographs following cervical spine hyperflexion injuries often show a minor cortical buckle injury along the anterior margin of the vertebral body. With more severe injuries, there is a triangular fracture fragment of the anterior aspect of the vertebral body or more extensive fragmentation of the bone (Figure 25-12). MR of hyperflexion injuries in which there is substantial posterior distraction or shear often shows manifestations of ligamentous injury adjacent to the posterior elements (Figure 25-13). Vertebral malalignment is an important sign of ligamentous injury on radiographs and CT. Facet joint malalignment can also occur with hyperflexion injuries.
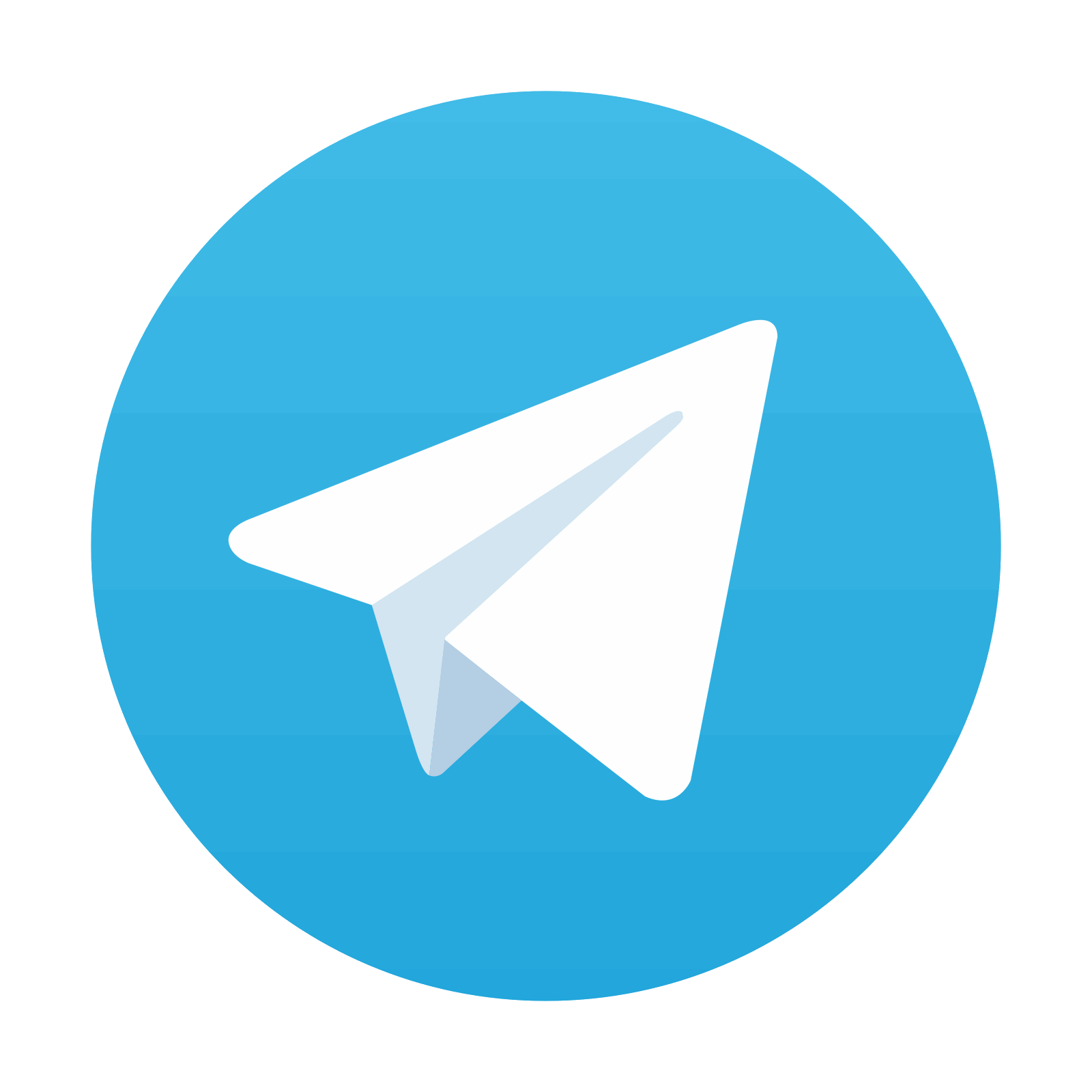
Stay updated, free articles. Join our Telegram channel

Full access? Get Clinical Tree
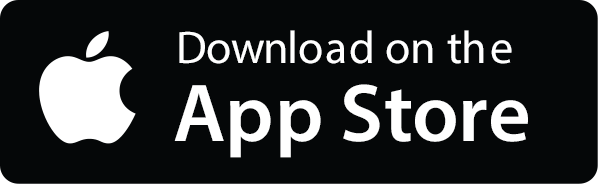
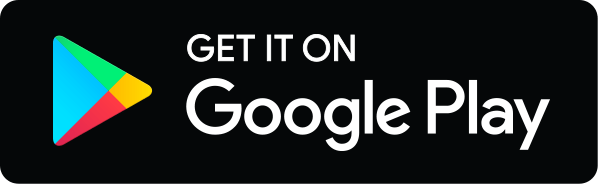