Transudative Pleural Effusions
Transudative pleural effusions occur when the systemic factors influencing the formation and absorption of pleural fluids are altered so that pleural fluid accumulates. In this chapter, the various causes of transudative pleural effusions are discussed.
CONGESTIVE HEART FAILURE
Congestive heart failure (CHF) is probably the most common cause of pleural effusion. The reason for the low incidence of pleural effusions secondary to heart failure in most studies is that researchers interested in pleural effusions usually do not see most patients with pleural effusions of this origin. In an epidemiologic study from the Czech Republic, CHF was the most common cause of pleural effusion (1). The incidence of pleural effusions in patients with CHF is high. Kataoka and Takada (2) studied 60 patients admitted to a Japanese hospital for an exacerbation of stable CHF with a computed tomography (CT) scan, ultrasound, and a chest radiograph. They reported that by CT scan, 50 patients (83%) had a right-sided pleural effusion, whereas 46 patients (77%) had a left-sided pleural effusion. Approximately one third of the effusions had volumes that exceeded 700 mL (2).
Race et al. (3) reviewed the autopsies at the Mayo Clinic between 1948 and 1953 of 402 patients who had CHF during life. The researchers found that 290 of the patients (72%) had pleural effusions with volumes greater than 250 mL. Of these, 88% had bilateral pleural effusions, whereas 8% and 4% had unilateral right-sided and left-sided effusions, respectively (3).
Pathophysiology
In recent years, concepts of pleural fluid formation and reabsorption in patients with heart failure have undergone significant modifications. In the past, it was believed that the pleural fluid that accumulated in patients with CHF was due to increased pressure in the capillaries in the visceral or the parietal pleura. These increased pressures were thought to result in an increased entry of fluid into the pleural space from the parietal pleura and a decreased removal of fluid through the visceral pleura, according to Starling’s equation.
The current theories on pleural fluid formation and reabsorption give us a different entry pathway and a different exit pathway for pleural fluid in patients with CHF. It appears that most of the fluid that enters the pleural space in patients with CHF comes from the alveolar capillaries rather than the pleural capillaries (4). When the pressure in the pulmonary capillaries is elevated, increased amounts of fluid enter the interstitial spaces of the lung. The increased fluid in the interstitial spaces results in an increased interstitial pressure in the subpleural interstitial spaces (5). The fluid then moves from the pulmonary interstitial spaces across the visceral pleura into the pleural space. There appears to be relatively little resistance to fluid movement from the pulmonary interstitial spaces across the visceral pleura (4). When pulmonary edema is produced in sheep with volume overloading, approximately 25% of the pulmonary edema fluid exits the lung through the visceral pleura (6).
Currently, it is believed that almost all fluid exits the pleural space through the lymphatics in the parietal pleura rather than by passively diffusing across the visceral pleura (see Chapter 2). Pleural fluid accumulates in patients with CHF when the rate of entry of fluid into the pleural space exceeds the capacity of the lymphatics in the parietal pleura to remove the fluid. In normal sheep, the capacity of the lymphatics
to remove fluid is approximately 0.28 mL/kg/hr (7). If there is elevated pressure in the systemic veins, the lymphatic clearance is decreased and the rate of fluid formation from the capillaries in the parietal pleura is increased (8).
to remove fluid is approximately 0.28 mL/kg/hr (7). If there is elevated pressure in the systemic veins, the lymphatic clearance is decreased and the rate of fluid formation from the capillaries in the parietal pleura is increased (8).
In the clinical situation, it appears that the accumulation of pleural fluid in patients with CHF is related more to left ventricular failure than to right ventricular failure. Wiener-Kronish et al. (9) prospectively evaluated 37 patients with CHF secondary to ischemic heart disease or to cardiomyopathy who were admitted to a coronary care unit. Nineteen patients had a pleural effusion. The mean wedge pressure in the patients with an effusion (24.1 ± 1.3 mm Hg) was significantly higher than in those without an effusion (17.2 ± 1.5 mm Hg). There was also a greater likelihood of finding pleural effusions if severe rather than mild pulmonary edema was found roentgenographically. In a subsequent study (10), these same researchers were unable to demonstrate any pleural effusions in 27 patients with chronic pulmonary hypertension or chronically elevated right atrial pressures.
Pleural effusions also occur in some patients with right ventricular failure (11,12,13). Tang et al (11) reviewed the records of 147 patients with idiopathic or familial hypertension and reported that 21 patients (14%) had pleural effusion for which there was no explanation. The patients with pleural effusion had significantly higher mean right atrial pressures than did those without effusion (11). The majority of the effusions were trace to small and right sided or bilateral (11). When the fluid was analyzed, it usually was a transudate (11). In another study by the same group, Luo et al. (12) reviewed the records of 89 patients with pulmonary arterial hypertension due to connective tissue disease and reported that 32.6% of the patients had a pleural effusion without an alternative diagnosis. The patients with pleural effusion had significantly higher right atrial pressures, BNP, and lower cardiac outputs (12). Patients with scleroderma had a higher incidence than did patients with systemic lupus erythematosus (12). The pleural effusions were predominantly small in size and bilateral. It therefore appears that many patients with right heart failure have pleural effusions (13).
In summary, it appears that pleural fluid accumulates in patients with CHF when they have either left or right ventricular failure. The high pressures in the pulmonary capillaries lead to increased amounts of fluid in the interstitial spaces. The fluid in the interstitial spaces enters the pleural space through the highly permeable visceral pleura. Fluid accumulates when the entry of fluid into the pleural space overwhelms the capacity of the lymphatics in the parietal pleura to remove the fluid. Small amounts of fluid may enter the pleural space from the capillaries in either pleural surface. Elevation of the systemic venous pressure also leads to the accumulation of pleural fluid because of increased fluid formation from the capillaries in the parietal pleura and decreased lymphatic clearance from the pleural space.
Clinical Manifestations
Pleural effusions due to CHF are usually associated with other manifestations of that disease. The patient often has a history of increasing dyspnea on exertion, increasing peripheral edema, and orthopnea or paroxysmal nocturnal dyspnea. The dyspnea is frequently out of proportion to the size of the effusion. Physical examination usually reveals signs of both right-sided heart failure with distended neck veins and peripheral edema and left-sided heart failure with rales and an S3 ventricular gallop as well as signs of the pleural effusions.
The chest radiograph almost always reveals cardiomegaly and usually bilateral pleural effusions. CHF is by far the most common cause of bilateral pleural effusions, but if cardiomegaly is not present, an alternate explanation should be sought. In one series of 76 patients with bilateral pleural effusions but a normal-sized heart, only 3 (4%) were due to CHF (14). Although in the past it was thought that pleural effusions due to CHF were commonly unilateral on the right or at least were much larger on the right side, this does not appear to be the case. In the autopsy series of Race et al. (3), 88% of the patients studied had bilateral pleural effusions. Moreover, the mean volume of pleural fluid in the right pleural space (1,084 mL) was only slightly greater than the mean volume of pleural fluid in the left pleural space (913 mL). In this series, 35 patients had unilateral pleural effusions, and of these 35 patients, 16 (46%) had either pulmonary embolism or pneumonia (3). A recent letter (15) to the editor summarized the sidedness of 444 effusions due to heart failure in five separate studies. Of the effusions, 69% were bilateral, 21% were unilateral on the right, and 9% were unilateral on the left. Of the bilateral pleural effusions, most were similarly sized but either side can be larger.
In patients with pleural effusions secondary to CHF, mediastinal lymphadenopathy is common. Erly et al. (16) reported that the prevalence of mediastinal lymph nodes with a diameter greater than 1 cm
on the short axis was 81% in 36 patients with an ejection fraction less than 35%.
on the short axis was 81% in 36 patients with an ejection fraction less than 35%.
Diagnosis
The diagnosis of pleural effusions secondary to CHF comes readily to mind every time a patient is seen with CHF. One must be careful to avoid the trap of ascribing the pleural effusion to CHF when it has another cause. In the series of Race et al. (3), more than 25% of the patients with CHF and pleural effusions had either pulmonary emboli or pneumonia at autopsy. Certainly, if the patient is febrile, has pleural effusions that are greatly disparate in size, has a unilateral pleural effusion, pleuritic chest pain, or does not have cardiomegaly, a diagnostic thoracentesis should be performed.
If the patient has cardiomegaly and bilateral pleural effusions, is afebrile, and does not have pleuritic chest pain, we initiate treatment of the CHF and observe the patient to determine whether the pleural fluid is reabsorbed. If the effusions do not disappear within a few days, we then perform a diagnostic thoracentesis. One problem with this approach is that with diuresis, the characteristics of the pleural fluid may change from those of a transudate to those of an exudate. Romero et al. (17) performed a thoracentesis on 21 patients with CHF before and every 48 hours after diuretic therapy was initiated. Before diuretics were administered, only one effusion was misclassified as an exudate by Light’s criteria, but at the time of the third thoracentesis 10 effusions were misclassified as exudates. Between the first and the third thoracentesis, the mean protein level increased from 2.3 to 3.5 gm/dL and the mean lactate dehydrogenase (LDH) level increased from 176 to 262 IU/L (17). Other authors (18,19) have also reported that the characteristics of the pleural fluid changed with diuresis.
The pleural fluid from a patient with CHF is typically a transudate with a ratio of pleural fluid to serum protein below 0.5, a ratio of pleural fluid to serum LDH under 0.6, and an absolute pleural fluid LDH level below two-thirds of the upper limit of normal for serum (Light’s criteria) (20). If the foregoing criteria are satisfied in a patient with CHF, the patient has a transudative pleural effusion that can be ascribed to the CHF, and no further diagnostic studies are indicated. Such transudative pleural effusions may be blood tinged, and the pleural fluid differential cell count may reveal predominantly polymorphonuclear leukocytes, small lymphocytes, or other mononuclear cells (21).
The pleural fluid from approximately 20% to 25% of patients with CHF will be classified as exudates by Light’s criteria (22). Most patients who are misclassified are receiving diuretics (22). If the pleural fluid meets exudative criteria but the effusion is thought to be due to CHF, the serum to pleural fluid protein gradient should be examined. If this gradient is greater than 3.1 g/dL, the pleural effusion in all probability is due to the CHF and additional diagnostic studies are not indicated (17). In patients with CHF on diuretic therapy, the protein gradient does not decrease much with diuresis (17). If the protein and LDH criteria are not met and the protein gradient is less than 3.1 g/dL, the pleural effusion is probably not due to the heart failure. Rather, the patient has an exudative pleural effusion, and further diagnostic tests such as pleural fluid cytologic study and a CT angiogram of the chest should be obtained. In previous editions of this book, it was recommended that in borderline pleural effusions the albumin gradient between the serum and pleural fluid be measured. If this gradient was more than 1.2 g/dL, then the effusion could be ascribed to the CHF. Currently, the protein gradient of 3.1 g/dL is preferred to the albumin gradient because the protein gradient is already available when Light’s criteria are measured. Moreover, the protein gradient appears to be as effective as the albumin gradient in making the distinction (17).
Another test that should be considered for establishing the diagnosis of CHF is measurement of the serum or pleural fluid N-terminal pro-brain natriuretic peptide (NT-proBNP). When the ventricles are subjected to increased pressure or volume, the biologically active BNP and the larger aminoterminal part NT-pro-BNP are released in equimolar amounts in the circulation (23). The thresholds for the diagnosis of heart failure via the BNP recommended by the manufacturers are 100 pg/mL for the Triage BNP assay and 125 pg/mL for the Elecsys pro-BNP assay (23). However, in clinical practice, levels below 100 pg/mL are thought to make CHF unlikely, whereas levels above 500 pg/mL are considered diagnostic of CHF (24). It should be noted that serum BNP levels are increased with acute pulmonary embolism when there is right ventricular dysfunction (25).
The pleural fluid levels of NT-proBNP are elevated in patients with heart failure. Porcel et al. (26) measured NT-proBNP levels in 117 pleural fluid samples including 44 with heart failure, 25 with malignancy, 20 with tuberculous pleurisy, and 10 with hepatic hydrothorax. They reported that the median level of NT-proBNP was 6,931 pg/mL in the patients with CHF,
which was significantly higher than that of 551 pg/mL in the patients with hepatic hydrothorax or 292 pg/ mL in the 63 patients with exudative effusions. A cutoff level of 1,500 pg/mL in the pleural fluid provided a sensitivity of 91% and a specificity of 93% in the diagnosis of heart failure. Tomcsányi et al. (27) compared the pleural fluid and serum NT-pro-BNP levels in 14 patients with CHF and 14 patients with pleural effusions of other etiologies. They reported that the median NT-pro-BNP levels in the patients with heart failure and other diseases were 6,295 and 276, respectively, in pleural fluid and 5,713 and 231, respectively, in serum (27). There was no overlap between the two groups. Interestingly, in the latter study, the correlation between the pleural fluid BNP levels and the serum levels was very high (R2 = 0.95) (27). Kolditz et al. (28) measured the serum and pleural fluid NT-pro-BNP levels in 93 patients including 25 with CHF. They confirmed the results of the study by Tomcsányi et al. (27) in that the levels of serum and pleural fluid NT-pro-BNP again were closely correlated (R2 = 0.90). They reported that an NT-pro-BNP cutofflevel of 4,000 pg/mL had a sensitivity of 92% and a specificity of 93% in making the diagnosis of CHF. Moreover, in this study nine patients with heart failure met Light’s exudative criteria and all of them had pleural and serum NT-pro-BNP levels greater than 4,000 pg/ mL (28). From the latter two studies, it appears that there is no need to measure both the pleural fluid and the serum NT-pro-BNP levels.
which was significantly higher than that of 551 pg/mL in the patients with hepatic hydrothorax or 292 pg/ mL in the 63 patients with exudative effusions. A cutoff level of 1,500 pg/mL in the pleural fluid provided a sensitivity of 91% and a specificity of 93% in the diagnosis of heart failure. Tomcsányi et al. (27) compared the pleural fluid and serum NT-pro-BNP levels in 14 patients with CHF and 14 patients with pleural effusions of other etiologies. They reported that the median NT-pro-BNP levels in the patients with heart failure and other diseases were 6,295 and 276, respectively, in pleural fluid and 5,713 and 231, respectively, in serum (27). There was no overlap between the two groups. Interestingly, in the latter study, the correlation between the pleural fluid BNP levels and the serum levels was very high (R2 = 0.95) (27). Kolditz et al. (28) measured the serum and pleural fluid NT-pro-BNP levels in 93 patients including 25 with CHF. They confirmed the results of the study by Tomcsányi et al. (27) in that the levels of serum and pleural fluid NT-pro-BNP again were closely correlated (R2 = 0.90). They reported that an NT-pro-BNP cutofflevel of 4,000 pg/mL had a sensitivity of 92% and a specificity of 93% in making the diagnosis of CHF. Moreover, in this study nine patients with heart failure met Light’s exudative criteria and all of them had pleural and serum NT-pro-BNP levels greater than 4,000 pg/ mL (28). From the latter two studies, it appears that there is no need to measure both the pleural fluid and the serum NT-pro-BNP levels.
We compared the pleural fluid NT-pro-BNP levels in 10 patients each with pleural effusions due to CHF, pulmonary embolism, post-coronary artery bypass surgery, and malignancy. All the patients with CHF had NT-pro-BNP levels above 1,500 pg/mL, whereas none of the other patients had such high levels (29). In view of the data given in the preceding text, it appears that a pleural fluid NT-pro-BNP level above 1,500 pg/mL is almost diagnostic that the patient has CHF.
It should be emphasized that the serum or pleural fluid BNP and NT-pro-BNP cannot be used interchangeably in the diagnosis of pleural effusions due to CHF (30). The NT-pro-BNP levels are about 10 times higher than the BNP levels. There is not a close correlation between the BNP levels and the NT-pro-BNP levels (r = 0.78) (31). The diagnostic usefulness of the NT-pro-BNP in making the diagnosis of heart failure is superior to that of the BNP (31,32). The pleural fluid NT-pro-BNP is also superior to the BNP and the protein gradient in identifying patients with heart failure who meet Light’s criteria for exudates (31). In one study of 20 patients with heart failure who met Light’s criteria for exudates, 18 had NT-pro-BNP levels above 1,300, 16 had NT-pro-BNP levels above 1,500, but only 10 had serum pleural fluid protein gradient greater than 3.1 g/dL (31).
Treatment
The preferred treatment of pleural effusion secondary to heart failure is to treat the heart failure with digitalis, diuretics, and afterload reduction. When the heart failure is successfully managed, the pleural effusion disappears. Such treatment effectively manages the pleural effusion in most patients with heart failure. For example, in a recent study of patients undergoing orthotopic heart failure, preoperatively only 19 of 60 (32%) had a pleural effusion and only 1 patient had a pleural effusion that occupied more than 25% of the hemithorax (33).
Occasionally, large pleural effusions cause patients to be very dyspneic. The removal of 500 to 1,000 mL of pleural fluid from such patients may rapidly relieve the dyspnea. If the effusions cannot be controlled with standard therapy, interventions to control the pleural effusions should be considered. One option is pleurodesis with a sclerosing agent (34) and currently, doxycycline 500 mg through a chest tube is recommended (see Chapter 10). Bleomycin is not recommended in this situation because it is not an effective agent in the rabbit model with normal pleura (35).
An alternative approach is to insert an indwelling pleural catheter or to use a pleuroperitoneal shunt. Herlihy et al. (36) inserted indwelling catheters (PleurX, CareFusion Corporation, San Diego, CA) into five patients with pleural effusions refractory to the usual therapy. Initially, the catheter was effective in controlling the effusion in all patients (36). However, after 5 and 15 months, two patient developed an empyema and after 4 months another patient developed a loculated pleural effusion (36). We have used this device in several patients with excellent results. With the PleurX, the patient connects the catheter to vacuum bottles every other day to drain the fluid (see Chapter 10). The pleuroperitoneal shunt (CareFusion Corporation, San Diego, CA) consists of two catheters connected with a valved pump chamber. The two one-way valves in the pump chamber are positioned such that fluid can only flow from the pleural space to the peritoneal cavity through the pump chamber. Because the pleural pressure is almost always more negative than the peritoneal pressure, the pumping chamber must be used to move fluid
from the pleural cavity to the peritoneal cavity. Little et al. (37) reported that two patients with refractory pleural effusions secondary to CHF were managed successfully with the pleuroperitoneal shunt.
from the pleural cavity to the peritoneal cavity. Little et al. (37) reported that two patients with refractory pleural effusions secondary to CHF were managed successfully with the pleuroperitoneal shunt.
HEPATIC HYDROTHORAX
Pleural effusions occur occasionally as a complication of hepatic cirrhosis. Pleural effusions usually occur only when ascitic fluid is present. Lieberman et al. (38) reviewed 330 patients with cirrhosis and ascites and found that 18 (5.5%) had pleural effusions. Johnston and Loo (39) found that 6.0% of 200 patients with cirrhosis had pleural effusions. In the second series, none of the 54 patients having cirrhosis without ascites had a pleural effusion (39). In some patients, the ascites is not clinically evident, but it can almost always be demonstrated with ultrasonography (40). The pleural effusion in patients with cirrhosis and ascites is usually right sided (67%), but occasionally it is left sided (16%) or bilateral (16%) (38,39).
Pathophysiology
Patients with cirrhosis frequently have decreased plasma oncotic pressure (39), and from Figure 2.1, one might hypothesize that the pleural effusions arise because of it. Indeed, in the experimental animal, the induction of decreased plasma oncotic pressure leads to the accumulation of pleural fluid (41). However, this mechanism does not appear to be the predominant cause of pleural effusions in patients with cirrhosis and ascites. Rather, the pleural effusions appear to be produced by movement of the ascitic fluid from the peritoneal cavity into the pleural cavity.
Johnston and Loo (39) demonstrated that after the intraperitoneal injection of India ink, cells in the pleural fluid contained many carbon particles, whereas cells in the peripheral blood contained none. In addition, after the intravenous injection of radiolabeled albumin, the albumin first appeared in the peritoneal fluid and then in the pleural fluid. Following the intraperitoneal injection of radiolabeled albumin, the concentration of the labeled protein was greater in the pleural fluid than in the plasma; after intrapleural injection, the labeled protein appeared in the plasma before it appeared in the peritoneal fluid (39). Because no air entered the pleural space following the intraperitoneal injection of carbon dioxide in one patient, these researchers concluded that the pleural effusion arose from the transfer of ascitic fluid from the peritoneal to the pleural space by the lymphatic vessels. This conclusion appears to have been incorrect. Datta et al. (42) injected radiolabeled human serum albumin into the peritoneal cavity of a patient with ascites and a large pleural effusion. They were able to demonstrate that when the labeled protein was picked up by the lymphatic system in the diaphragm, it flowed into normal mediastinal lymphatic channels and from them into the subclavian vein. It did not enter the pleural space.
Studies by Lieberman et al. (38) suggest that fluid passes directly from the peritoneal to the pleural cavity through pores in the diaphragm. These researchers introduced 500 to 1,000 mL of air into the peritoneal cavity of five patients with cirrhosis, ascites, and pleural effusions. In all five patients, a pneumothorax developed 1 to 48 hours after the induction of the pneumoperitoneum. Thoracoscopic examination was performed in three other patients after the induction of the pneumoperitoneum, and in one of these patients, air bubbles were seen coming through an otherwise undetectable diaphragmatic defect (38). At postmortem examination, diaphragmatic defects were demonstrated in two of the patients (38). Huang et al. (43) prospectively studied 11 patients with hepatic hydrothorax who underwent thoracoscopy. These researchers classified the morphologic findings into four morphologic types: type I, no obvious defect (one patient); type II, blebs lying on the diaphragm (four patients); type III, broken defects (fenestrations) in the diaphragm (eight patients); and type IV, multiple gaps in the diaphragm (one patient). The movement of fluid from the peritoneal cavity through the diaphragm can also be demonstrated by contrast enhanced ultrasound (44).
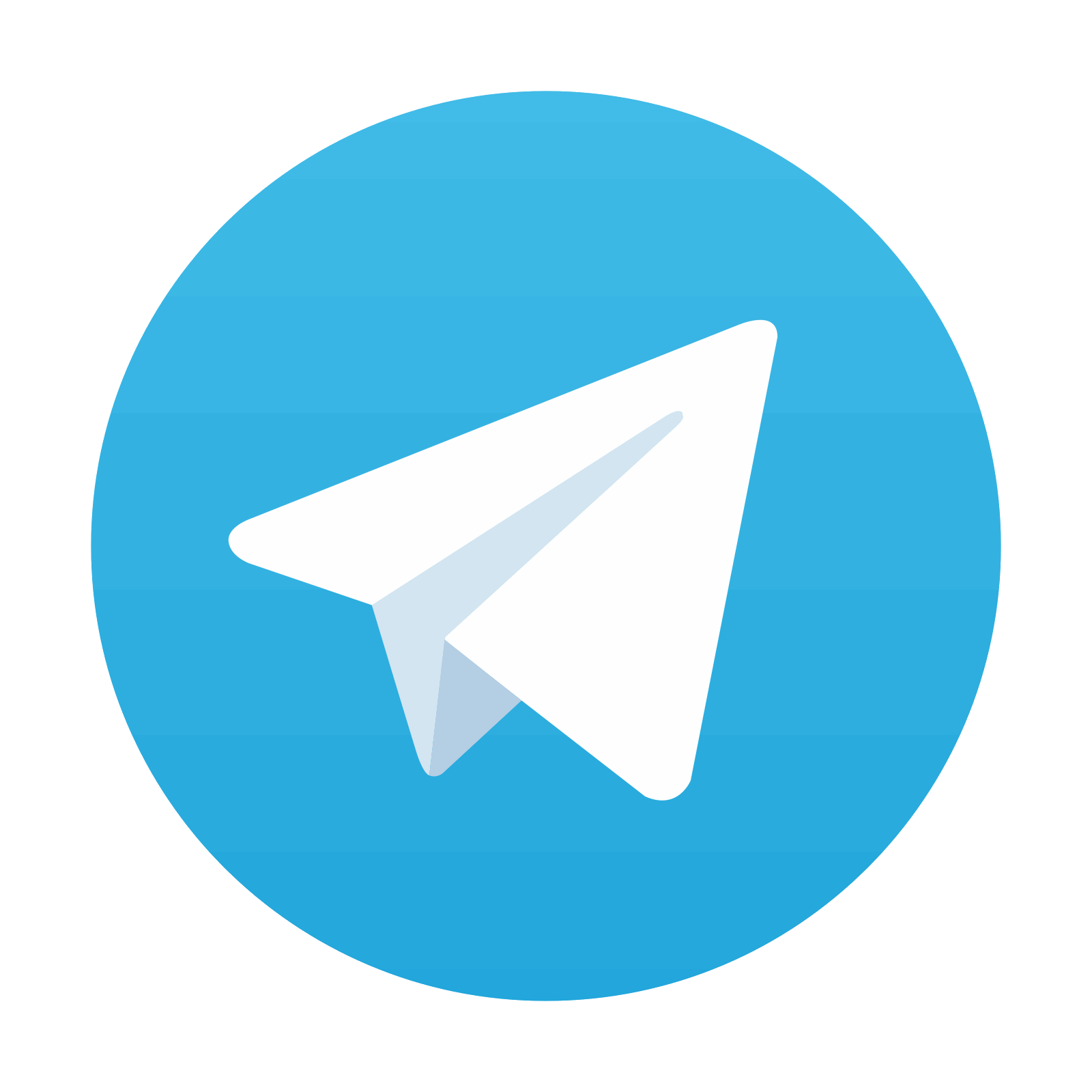
Stay updated, free articles. Join our Telegram channel

Full access? Get Clinical Tree
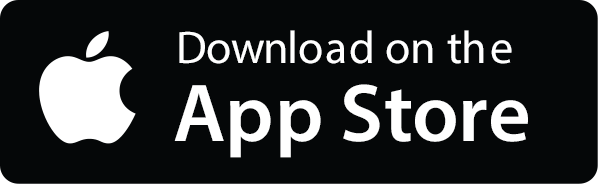
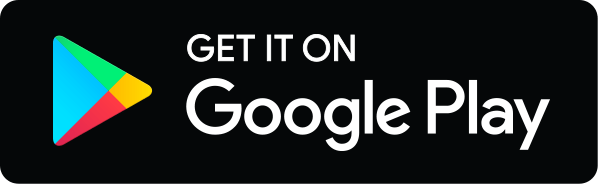
