First author
Ergonovine
Acetylcholine
Invasive testing
Japanese Circulation society [33]
Ergonovine 20–60 ug (LCA, IC)
20–100 ug (LCA, IC)
Ergonovine 20–60 ug (RCA, IC)
20–50 ug (RC, IC)
Okumura et al. [19]
200 ug IV
20–100 ug (LCA, IC)
20–50 ug (RCA, IC)
Song et al. [35]
Ergonovine 1–30 ug IC
10–100 ug IC
Sueda et al. [38]
Ergonovine 64 ug (LCA, IC)
20–100 ug (LCA, IC)
Ergonovine 40 ug (RCA, IC)
20–50 ug (RCA, IC)
Takagi et al. [41]
Ergonovine 20–60 ug (LCA, IC)
20–100 ug (LCA, IC)
Ergonovine 20–60 ug (RCA, IC)
20–50 ug (RCA, IC)
Yasue et al. [42]
NA
Suspected vessel 10–100 ug IC
Contralateral vessel:
20–200 ug (LCA, IC)
20–50 ug (RCA, IC)
NA
20, 50, 100 ug (LCA, IC)
20–50 ug (RCA, IC)
In our center, this test has been performed for the past 10 years in over 6000 patients presented with unexplained chest pain syndrome with normal and near-normal coronary artery anatomically (<30 % stenosis). Acetylcholine provocation test results are more sensitive for the typical patients of vascospastic angina despite less specific response than the ergonovine test. It means that it is safe to treat patients according to acetylcholine provocation results and there will be limited chance of false negative results. The usual dose of acetylcholine provocation test is described as in Table 26.1.
26.4 Pathophysiology of Vasospastic Angina
Several mechanisms have been described in the pathogenesis of this vasospastic syndrome though the mechanisms are poorly defined and likely multifactorial. Generally, the mechanisms of vasospasm have been postulated to involve either the vascular endothelial cells or the hyperreactive vascular smooth muscles. Patients have spontaneous angina episodes associated with reversible tightening of focal segment or segments of the coronary artery leading to functional obstruction of the blood flow causing myocardial ischemia. In this vasospastic syndrome, intracoronary acetylcholine induces spasm at high frequency without compromising hemodynamic status [42]. Usually acetylcholine expands blood vessels when the endothelium is normal, but it contracts blood vessels when there is endothelial detachment or injury. The potent smooth muscle relaxant, nitric oxide (NO), is secreted from the endothelial cells when the endothelium is normally intact [26, 44]. In healthy endothelium, NO is produced by reactive NO species (eNOS) when it is activated by various cellular signals. Elevation of intracellular calcium level due to mechanical shear stress in turn activates eNOS activity via calmodulin thereby promotes NO release and hence vasodilatation. This receptor mediated vasodilatation is induced by many vasoactive physiological mediators including acetylcholine, bradykinin, G proteins, and phospholipase C in vascular endothelium leading to inositol triphosphate production and release of stored ionic calcium in the cells. As a result of this receptor activation, cellular calcium inflow is promoted via the ionic channels. Further stimulation by physiological active substances like acetylcholine, insulin, and bradykinin coupling with the mechanical shear stress on vascular wall also enhance release of eNOS activity [45]. NO stimulates soluble guanylate cyclase in vascular smooth muscle to increase the level of cyclic guanosine monophosphate (cGMP) and hence vasodilatation. Under standard conditions, NO is produced and released from healthy endothelial cells. In vasospastic coronary arteries, this hypereactivity to nitroglycerine is perhaps owing to the absence of baseline production and release of NO from the healthy endothelium present in the arteries [46].
The mechanism of vascular smooth muscle cells contraction has recently been described [47, 48]. Under the influence of vasoconstrictive substance, G protein coupling with phospholipase C (PLC) in smooth muscle cells is stimulated to release inositol triphosphate (IP3). IP3 opens calcium ion channel in the sarcoplasmic reticulum. Ionic calcium is released into the cytoplasm resulting in increased intracellular calcium concentration. In addition, calcium channels are also present on the cell membrane that opens under various physiological stimuli. The additive effect is the sudden surge of intracellular calcium concentration. Intracellular calcium ions bind to calmodulin to form Calcium/calmodulin complexes. These complexes will then bind to the catalytic subunit of myosin light chain kinase (MLCK) converting it to its active form. Phosphorylation of the myosin light chain (MLC) by the active MLCK activates Magnesium-ATPase in the head of myosin by actin. This results in vascular smooth muscle cells contraction. Following that, the intracellular calcium level falls, calcium/calmodulin complex dissociates and MLCK becomes inert. The activity of myosin light chain phosphatase (MLCPh) become predominant, MLC will undergo phosphorylation and resulting in vascular smooth muscle relaxation [49]. MLC phosphorylation is encouraged by MLCK but suppressed by MLCPh. Rho-kinase has been shown to suppress MLCPh activity [50]. Rho-kinase controls the balance between contraction and relaxation of the vascular smooth muscle. Its action is not dependent on intracellular calcium level. Once activated by vasoactive substances, Rho, a low molecular weight G protein, is activated by the G-protein-coupling receptor. At the same time, the target protein, Rho-kinase, is activated. Rho-kinase inhibits the phosphorylation of MBS, rendering MLCPh inactive. This produces imbalance between MLCK’s and MLCPh’s activity therefore promoting phosphorylation of MLC and resulted in exaggerated contraction of vascular smooth muscle [50, 51].
Therefore, the cellular basic of hyperactive coronary vascular smooth muscle cell consist of the end substrate for the evolution of coronary artery spasm. The pathogenesis of this condition is likely to be multifactorial and heterogeneous among different population. Several other pathophysiological factors also have an important role in the genesis of this vasospastic entity including the autonomic nervous system, endothelial dysfunction, systemic inflammation, oxidative stress and genetics. Other environmental factors, for instance, smoking, metabolic abnormalities, and alcohol consumption may too contribute to its genesis. Racial variations in the incidence have been reported with higher prevalence in Korea and Japan suggesting a genetic influence [52]. Lastly, the interplay among these factors in the evolution of coronary spasm is rather elusive and poorly defined.
26.5 Transradial Provocation Testing
Transradial route has been increasingly used as the preferred access route for coronary intervention. The most compelling reason for adopting transradial access is the increased patient safety that results from virtually elimination of access site vascular complications and bleeding [53]. Besides, transradial route has the advantage of early sheath removal, faster recovery, patient’s comfort and lower cost compared to femoral access [54]. With that in mind, we have been performing vasospasm provocation test in our center since the initial inception of the invasive cardiovascular center in 2004. To date, we have been performing more than 6000 patients for the past 10 years.
In our usual workflow for intracoronary spasm provocation test, the patient with chest pain syndrome will undergo a routine coronary angiography via the transradial route as mentioned in the prior chapter. All vasodilators or vasoconstrictor are withheld for at least 72 h prior to coronary angiography. Routine coronary angiography is performed early in the morning and if the angiography is normal or near-normal; Acetylcholine provocation test ensues immediately after the arteriography via the 4 F transradial diagnostic catheter. Control angiography of the left and right coronary arteries is performed. The best angiography projection is taken to ensure separation of each of the branches of the vascular trees. The angiography is then repeated after acetylcholine injection at the same projection again. Upon completion of the procedure, nitrate is administered and angiography is performed at the same projection again while the coronary is maximally dilated. RAO cranial or AP cranial view was routinely evaluated for the observation of combined myocardial bridge (MB).
Currently, we are using 4 F transradial diagnostic catheters for this purpose. There are three different types of 4 F single catheters for bilateral engagement (both left and right coronary arteries) in our center that can be used for the provocation test either with ergonovine or acetylcholine. They are 4 F RM (Rha-Moon) catheter (Jung Sung Medical, JSM, Korea), 4 F CR (Cheon-Rha) catheter (Jung Sung Medical, JSM, Korea), 4 F Tiger catheter (Terumo, Japan). The descriptions for the procedure are illustrated in Figs. 26.1, 26.2 and 26.3.





Fig. 26.1
Acetylcholine provocation test with 4 F RM catheter. Coronary Arteriogram of the left coronary artery was performed in right anterior oblique projection via the right radial route with 4 F RM catheter. A control angiography was taken at baseline. Subsequently, an incremental dose of intracoronary Acetylcholine was administered. In this patient, the third dose (100 ug) of acetylcholine induced left anterior descending artery spasm. A completion angiography was repeated with administration of nitroglycerine 200 mcg at the same projection. Interpretation was performed by comparing the A3 to the final angiography after nitrate administration. (A1 Acetylcholine 20 ug, a2 Acetylcholine 50 ug, A3 Acetylcholine 100 ug, N nitroglycerine 200 ug)

Fig. 26.2
Acetylcholine provocation test with 4 F CR catheter. Coronary Arteriogram of the left coronary artery was performed in right anterior oblique projection via the right radial route with 4 F CR catheter. A control angiography was taken at baseline. Subsequently, an incremental dose of intracoronary Acetylcholine was administered. In this patient, the mid-distal portion of the left anterior descending artery looked vasospastic after the first dose of acetylcholine but this was not clinical significant without angina or dramatic electrocardiographic changes. After the third dose (100 ug) of intracoronary acetylcholine injection, this induced a significant left anterior descending artery spasm. A completion angiography was repeated with administration of nitroglycerine 200 mcg at the same projection which showed maximal vasodilatation. Interpretation was performed by comparing the A3 to the final angiography after nitrate administration. (A1 Acetylcholine 20 ug, A2 Acetylcholine 50 ug, A3 Acetylcholine 100 ug, N nitroglycerine 200 ug)


Fig. 26.3
Intracoronary Ergonovine provocation test (Panel a and b). Coronary angiogram of the left coronary artery was performed in right anterior oblique projection via the right radial route with 4 F Tiger catheter. A control angiography was taken at baseline. Subsequently, an incremental dose of intracoronary Ergonovine was administered. In this patient, the Ergonovine challenge test failed to induce vasospasm of the left anterior descending artery (Panel a) and right coronary artery (Panel b) despite repeated at incremental maximal doses. Nevertheless, due to high clinical suspicious, a repeat test was performed using Acetylcholine in Panel c. (E1 Ergonovine 10 ug, E2 Ergonovine 20 ug, E3 Ergonovine 50 ug). Subsequent intracoronary acetylcholine provocation test after negative ergonovine provocation test (Panel c). An immediate repeat test was performed with Acetylcholine. Baseline control coronary arteriogram of the left coronary artery was performed in right anterior oblique projection via the right radial route with 4 F Tiger catheter. An incremental dose of intracoronary Acetylcholine was administered after failing Ergonovine challenge test. In this patient, a diffuse left anterior descending artery spasm was induced with 50 ug of intracoronary Acetylcholine (A2 dose). The test was aborted when coronary spasm was induced and intracoronary nitroglycerine 200 ug was administered to reverse the vasospastic effect. A completion angiography was repeated with administration of nitroglycerine 200 mcg at the same projection which showed maximal vasodilatation. Interpretation was performed by comparing the A2 to the final angiography after nitrate administration. (A1 Acetylcholine 20 ug, A2 Acetylcholine 50 ug, N nitroglycerine 200 ug)
Coronary artery spasm is prompted by intracoronary injection of acetylcholine after diagnostic angiography. Acetylcholine is injected by 20 (A1), 50 (A2) and 100 (A3) μg/min into the left coronary artery over a 1 min period with 5 min intervals to the maximum tolerated dose under constant monitoring of electrocardiogram (ECG) and blood pressure. Angiography is then repeated after each acetylcholine dose until significant vasoconstriction (70 %) response. Intracoronary infusion with 0.2 mg nitroglycerine is given after completing the acetylcholine provocation test. Angiography is then performed 2 min later. If focal or diffuse significant vasoconstriction of coronary arteries is induced with any dose of acetylcholine by visual assessment, the procedure is stopped. End systolic images for each segment of the left coronary artery are chosen according to the corresponding points on the electrocardiographic trace (QRS onset or end of T wave) and analysed using the proper quantitative coronary angiographic (QCA) system of the catheterization laboratory (FD-20, Phillips, Amsterdam, The Netherlands). The coronary artery diameters are measured with QCA before and after the administration of acetylcholine at the site that show the greatest changes following drug administration. Reference diameter is measured at the proximal and distal portion of each artery before and after the intracoronary acetylcholine infusion and the mean reference diameter is used to assess the diameter narrowing.
During the coronary angiography and acetylcholine provocation test, significant CAS is defined as focal or diffuse severe transient luminal narrowing (>70 %) by visual assessment with or without chest pain or ischemic ECG change such as ST-T segment elevation, depression (≥1 mm) or T wave inversion. The normal coronary appearance is defined as less than 20 % luminal narrowing on coronary angiogram that is measured with QCA. Multi-vessel spasm (MVS) is defined as significant CAS of ≥2 major epicardial coronary arteries. The presence of baseline spasm is defined as focal or diffuses narrowing more than 30 % in diameter on the angiogram before the acetylcholine provocation test in comparison to final angiogram after intracoronary nitroglycerine infusion. Diffuse CAS is defined as significant CAS site length ≥30 mm.
In our center, intracoronary ergonovine provocation test is rarely performed nowadays in our patients due to its adverse effect and safety issues as described earlier. An example of ergonovine challenge test is illustrated in Fig. 26.3 in which it failed to induce vasoconstriction in one such patient and subsequent acetylcholine provocation test performed was positive.
26.5.1 Safety and Practice Guidelines
From our experience, transradial intracoronary spasm provocation test is generally safe and feasible. We have not encountered any untoward conventionally described detrimental effects in our patients such as prolonged ischemia, refractory ventricular tachyarrhythmia, myocardial infarction or even death. This is consistent with the contemporary reports that provocation testing is relatively safe [41]; though prolonged ischemia, MI, tachyarrhythmia and death has been reported in the older scientific literatures as a result of prolonged refractory spasm or recurrent spasm after testing [55, 56]. The safer results in our center coming from the modified (+) provocation test criteria as transient luminal narrowing greater than 70 % instead of traditional transient total or subtotal occlusion.
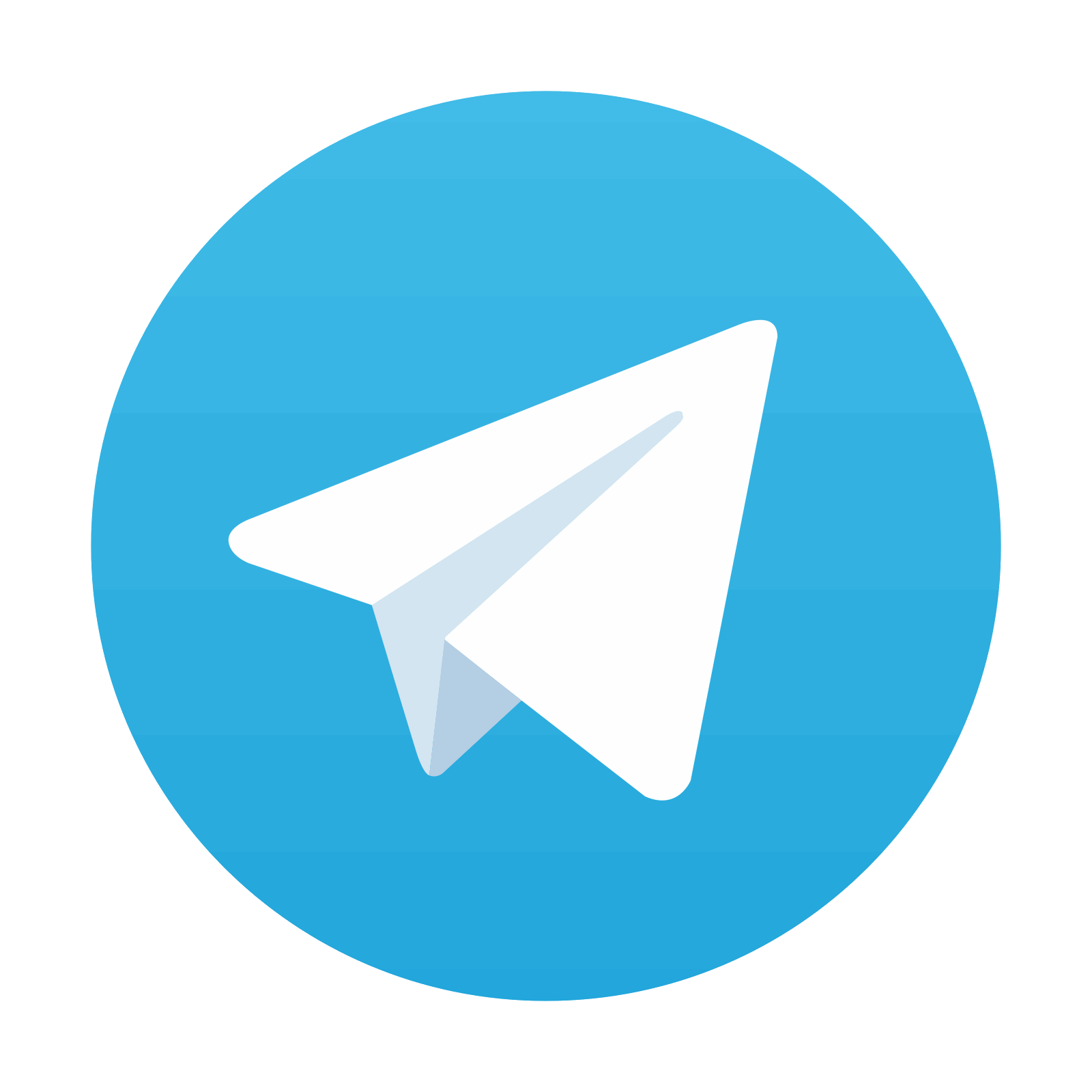
Stay updated, free articles. Join our Telegram channel

Full access? Get Clinical Tree
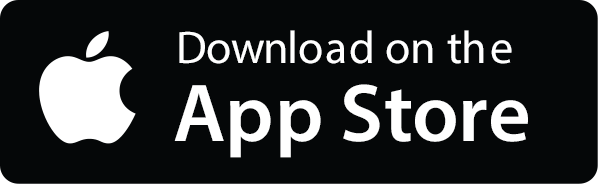
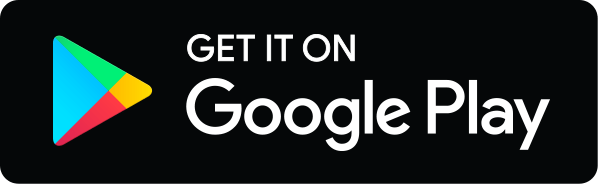