Fig. 4.1
Hypothetical depiction of survival benefit margin for a stable recipient in good condition (Recipient A) and a seriously ill recipient with multiple risk factors (Recipient B) (Kirklin et al. [1] with permission)
Recipient Evaluation, Indications and Listing for Transplantation
The evaluation process for patients with end-stage heart disease integrates the goals of avoiding cardiac transplantation either prematurely or too late in the natural history of the disease, considering alternative therapies to cardiac transplantation, and detecting comorbidity that would contraindicate transplantation. Therefore, the approach to patients with end-stage heart disease [1] is to (a) detect potentially reversible causes of heart failure, (b) evaluate severity of heart failure and functional capacity, (c) tailor medical therapy to relieve symptoms, (d) assess risks of rapid progression of heart failure or sudden death, (e) identify indications for transplantation, (f) exclude contraindications to transplantation, (g) and throughout the evaluation and listing process, continue to manage heart failure and regularly reevaluate the patient to ensure appropriateness of the therapeutic strategy.
A critical part of the evaluation process is the identification of processes for which there may be an alternative to transplantation. For example, it is important in patients with ischemic heart disease to determine the extent of myocardial viability since the disease may be amenable to percutaneous or surgical options. Similarly in patients with end stage valvular heart disease an assessment must be made of the likelihood that the patient may achieve equivalent or better survival with conventional valvular surgery as compared with cardiac transplantation. Non-surgical therapy [2] involves a number of possible strategies. Pharmacologic therapy with vasodilator agents, diuretics and beta blockers must be optimized and it is important that the results of maximal medical therapy should be observed for several months if possible before proceeding with consideration of cardiac transplantation. Control of arrhythmias [2] is an important issue – ventricular arrhythmias should be managed with anti-arrhythmic therapy, catheter ablation and device implantation or some combination of these treatments. In patients with atrial fibrillation, restoration of sinus rhythm or rate control is required. It is important that counterproductive agents be discontinued such as alcohol, illicit drugs and non-steroidal anti-inflammatory agents. Biventricular pacing should be considered in patients with prolonged QRS [2].
Implicit in the timing for listing for cardiac transplantation is the assumption that the natural history of end-stage heart disease can be predicted, but unfortunately this information is still quite imperfect. There have been a number of attempts made to develop predictive equations for survival with advanced heart failure, and this information is important to incorporate into the decision-making process; for example, the Heart Failure Survival Score (HFSS) from Columbia-Presbyterian Medical Center [3, 4]. This score was developed using multivariable methods to determine both noninvasive and invasively derived variables that predict either death without transplantation or the need for urgent cardiac transplantation.
The Seattle Heart Failure Model [5] is a widely used prediction model of survival of heart failure patients with the advantage that it uses clinical characteristics that are available in essentially all patients (as opposed to models that require invasively derived variables). An attractive feature of the Seattle Heart Failure Model is its ability to predict survival and the impact on survival of adding medications and/or devices in an individual patient (Fig. 4.2), although it has been suggested that this model overestimates survival in patients with implanted devices [6]. Other models (such as the MUSIC Risk Score) [7] incorporate biomarkers, in this case troponin and amino-terminal pro-brain natriuretic peptide. A predictive model of heart failure mortality [8] that incorporates multiple time related cytokine (tumor necrosis factor and interleukin-6) levels as well as “ensemble modeling”, a technique developed from statistical machine learning that combines the results of multiple statistical models, (by adjusting for the biological variability inherent in clinical studies) has improved the accuracy of these predictive models.
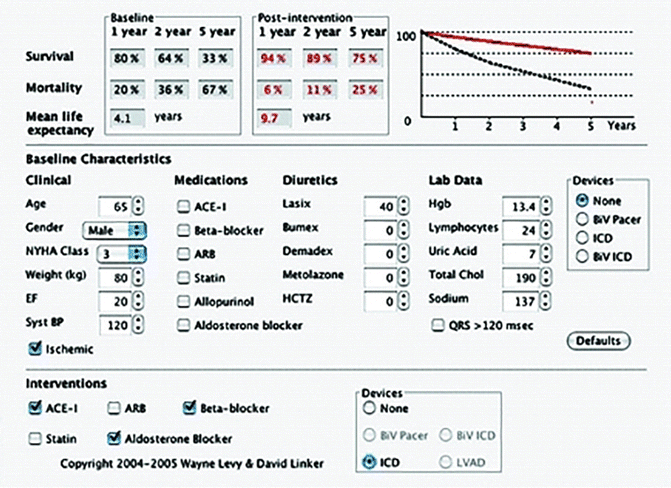
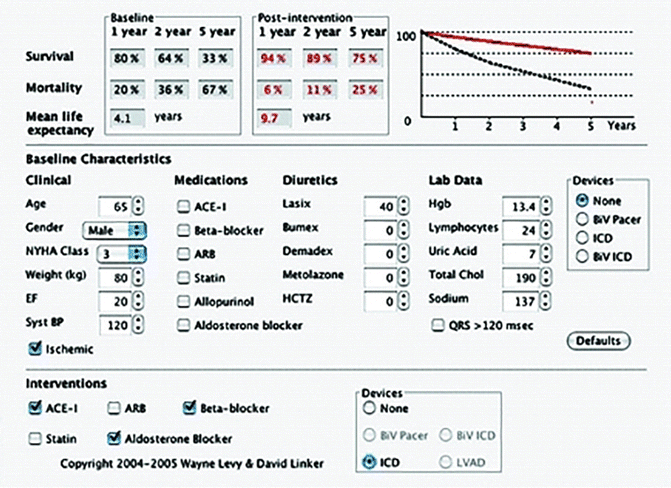
Fig. 4.2
An example of the output from the Seattle Heart Failure Model for a patient with the specified characteristics with the predicted survival before and after the listed interventions. The baseline survival curve is the straight line and the dotted line is the survival after intervention (Levy et al. [5] with permission. Copyright 2004–2005 Wayne Levy & David Linker)
A protocol for potential recipient evaluation is outlined in Table 4.1. Part of the evaluation includes determination of the immunologic status for the purpose of recipient-donor matching and assessment of the adequacy of the patient’s social support and financial resources since deficiencies in these areas may be just as threatening to survival and quality of life as any comorbid medical condition.
Table 4.1
Evaluation protocol for cardiac transplantation
General |
Complete history and physical examination |
Nutritional status evaluationa |
Blood chemistries including liver and renal profiles (bilirubin, SGOT[AST], alkaline phosphatase, BUN, creatinine, calcium, phosphorus, magnesium) |
Hematology and coagulation profile (complete blood cell count, differential, platelet count, prothrombin time [or International Normalized Ratio], partial thromboplastin time, fibrinogen) |
Serum electrolytes |
Lipid profilea |
Urinalysis |
24-h urine for creatinine clearance (and protein if diabetic or urinalysis positive for proteina |
Nuclear renal scan with measurement of effective renal plasma flowa (ERPF) |
Pulmonary function testing with arterial blood gases |
Ventilation-perfusion scana |
Stool for heme |
Mammographya |
Prostate-specific antibody (PSA)a |
Abdominal ultrasound study (liver, pancreas, gall bladder, and kidney evaluation) |
Carotid ultrasound |
Social evaluation |
Psychiatric evaluation |
Neuropsychiatric evaluation (neurocognitive evaluation)a |
Dental evaluation |
Sinus filmsa |
Cardiovascular |
Electrocardiogram |
Chest radiograph, (PA and lateral) |
Two-dimensional echocardiogram with Doppler study |
Exercise test with oxygen consumption (peak Vo2) |
Right-heart catheterization with detailed hemodynamic evaluation |
Shunt seriesa |
Left-heart catheterization with coronary angiographya |
Myocardial biopsya |
Radionuclide angiogram (gated blood pool study)a |
Nuclear imaging study for myocardial viability (thallium-201 or positron emission tomography)a |
Holter monitor for arrhythmias (if ischemic cardiomyopathy)a |
Immunology |
ABO blood type and antibody screen |
Panel reactive antibody (PRA) screen |
Human leukocyte antigen (HLA) typing (if to be listed for transplantation) |
Infectious disease screening |
Serologies for hepatitis A, B, and C; Herpes virus, human immunodeficiency virus (HIV), cytomegalovirus (CMV), toxoplasmosis, varicella, rubella, Epstein-Barr virus, veneral disease research laboratory (VDRL), Lyme titersa, histoplasmosis and coccidioidomycosis complement-fixing antibodiesa |
Throat swab for viral cultures (CMV, adenovirus, Herpes simplex virus)a |
Urine culture and sensitivitya |
Stool for ova and parasitesa |
It is important to remember that advanced heart failure may result in organ injury that may become irreversible and either compromise survival after cardiac transplantation or may contraindicate cardiac transplantation. It is implicit that consideration be given to cardiac transplantation before irreversible secondary changes become established.
General guidelines have been established as indications for cardiac transplantation [1] (Table 4.2) although increasing knowledge of the results of alternative therapies for patients with heart failure, improving results of cardiac transplantation with the seemingly immutable shortage of donor hearts, and the specific criteria that precipitates listing for transplantation are still very much in flux. However, it is generally accepted that patients should be considered for cardiac transplantation when they have New York Heart Association (NYHA) class III-IV heart failure, severe reduction in the quality of life due to their symptoms, and a predicted 2-year survival of less than approximately 60 %.
Table 4.2
General indications for cardiac transplantation
Criteria for consideration of heart transplantation in advanced heart failure |
Significant functional limitation (NYHA Class III-IV heart failure) despite maximum medical therapy that includes digitalis, diuretics, and vasodilators, preferably angiotensin-converting enzyme inhibitors, at maximum tolerated doses |
Refractory angina or refractory life-threatening arrhythmia |
Exclusion of all surgical alternatives to transplantation, such as the following: |
Revascularization for significant reversible ischemia |
Valve replacement for severe aortic valve disease |
Valve replacement or repair for severe mitral regurgitation |
Appropriate ventricular remodeling procedures |
Indications for cardiac transplantation determined by severity of heart failure despite optimal therapy |
Definite indications |
Vo2 max <10 ml/kg/min |
NYHA Class IV |
History of recurrent hospitalization for congestive heart failure |
Refractory ischemia with inoperable coronary artery disease and left ventricular ejection fraction <20 % |
Recurrent symptomatic ventricular arrhythmias |
Probable indications |
Vo2 max <14 mg/kg/min (or higher with multiple other risk factors) |
NYHA Class III–IV |
Recent hospitalizations for congestive heart failure |
Unstable angina not amenable to coronary artery bypass grafting, percutaneous transluminal coronary angioplasty with left ventricular ejection fraction <0.25 |
There are a number of contraindications to cardiac transplantation [2] (Table 4.3). Although there would be general agreement that many of these contraindications are absolute, some would be regarded as relative, based on programmatic experiential reasons or risk/benefit considerations in an individual patient. For example, in many programs renal dysfunction (due to intrinsic renal disease or associated with heart failure) would not be regarded as a contraindication as excellent results can be achieved with combined heart-kidney transplantation.
Table 4.3
Contraindications to heart transplantation
Absolute contraindications |
Systemic illness with a life expectancy of less than 2 years despite heart transplant, including |
Active or recent solid organ or blood malignancy within 5 years (e.g. Leukemia, low-grade neoplasms of prostate with persistently elevated prostate-specific antigen) |
AIDS with frequent opportunistic infections |
Systemic lupus erythematosus, sarcoid, or amyloidosis that has multisystem involvement and is still active |
Irreversible renal or hepatic dysfunction in patients considered for only HT |
Significant obstructive pulmonary disease (FEV1 <1 L/min) |
Fixed pulmonary hypertension |
Pulmonary artery systolic pressure >60 mmHg |
Mean transpulmonary gradient >15 mmHg |
Pulmonary vascular resistance >6 Wood units |
Relative contraindications |
Age >72 years |
Any active infection (with exception of device-related infection in VAD recipients) |
Active peptic ulcer disease |
Severe diabetes mellitus with end-organ damage (neuropathy, nephropathy, or retinopathy) |
Severe peripheral vascular or cerebrovascular disease |
Peripheral vascular disease not amenable to surgical or percutaneous therapy |
Symptomatic carotid stenosis |
Ankle brachial index <0.7 |
Uncorrected abdominal aortic aneurysm >6 cm |
Morbid obesity (body mass index >35 kg/m2) or cachexia (body mass index <18 kg/m2) |
Creatinine >2.5 mg/dL or creatinine clearance <25 mL/mina |
Bilirubin >2.5 mg/dL, serum transaminases >3× normal, INR >1.5 off warfarin |
Severe pulmonary dysfunction with FEV1 <40 % normal |
Recent pulmonary infarction within 6–8 weeks |
Difficult-to-control hypertension |
Irreversible neurological or neuromuscular disorder |
Active mental illness or psychosocial instability |
Drug, tobacco, or alcohol abuse within 6 months |
Heparin-induced thrombocytopenia within 100 day |
The etiologies of heart failure most frequently considered for transplantation are ischemic heart disease and dilated cardiomyopathy. A much smaller proportion of adult patients undergo transplantation for heart failure associated with previous correction of congenital heart disease such as a failing Fontan procedure, end-stage valvular heart disease, and retransplantation.
There are disease processes that were previously regarded as absolute contraindications to cardiac transplantation that are now being considered under experimental protocols. For example, amyloidosis has previously been regarded as an absolute contraindication to cardiac transplantation, but a protocol of orthotopic cardiac transplantation using extended-donor organs followed by high dose chemotherapy and stem cell transplantation in patients with primary amyloidosis, reported by Maurer and colleagues resulted in survival that was significantly better than medical treatment alone (75 % versus 23 % p < 0.0006). Furthermore the survival of these patients was similar to that of patients receiving cardiac transplantation on the alternate as well as the standard list [9].
Information [10] from the registry of the International Society for Heart and Lung Transplantation illustrates the dramatic impact that mechanical circulatory support has had on the management of heart failure in cardiac transplantation. The proportion of patients receiving heart transplantation who were bridged with mechanical circulatory support (left ventricular assist device, right ventricular assist device, biventricular assist device support or total artificial heart) is now just over 30 %. The landscape of mechanical circulatory support is continually changing with improvements in the engineering aspects of the devices, evolution from pulsatile to continuous flow devices and the improvement in their reliability, as well as increasing clinical information that is providing some clarity to the appropriate situations where mechanical circulatory support may be of benefit. The use of mechanical circulatory support, particularly as a bridge to transplantation, represents a balance between the probability of survival to transplantation versus the serious potential complications associated with implantation of a mechanical circulatory support device including operative risk, infection, sensitization with allo-antibodies, stroke, acute and chronic bleeding episodes and device failure. In order to better refine the indications for mechanical circulatory support as a bridge to transplantation the NIH-funded Interagency Registry for Mechanical Assist Devices (INTERMACS) was initiated [11]. An important component of the appropriate application of mechanical circulatory support is a system of classification based on the severity of heart failure (Table 4.4). It is the application of mechanical circulatory support to patients with the highest acuity of illness (INTERMACS level 1 and 2) where the greatest amount of controversy exists, but current opinion is that in patients in cardiogenic shock, their best chance of survival is a temporary support device with stabilization and recovery of the inevitable organ dysfunction before proceeding with a more definitive device to bridge the patient to transplantation [12].
Table 4.4
INTERMACS levels of limitation at the time of implantation and the time frame of need for consideration of MCS
INTERMACS profile level | Status | Time frame |
---|---|---|
1 | Critical cardiogenic Shock | Hours |
2 | Progressive decline | Days to week |
3 | Stable but inotrope Dependent | Weeks |
4 | Recurrent advanced HF | Weeks to few months if baseline restored |
5 | Exertion intolerant | Weeks to months |
6 | Exertion limited | Months, if nutrition and activity maintained |
7 | Advanced NYHA class III |
It is important to be aware of the time course of events after implantation of mechanical circulatory support devices as a bridge to transplantation (Fig. 4.3). By 12 months after implantation of a device as a bridge to transplantation 32 % have undergone transplantation and 48 % still have the device, so durability of the device is essential.
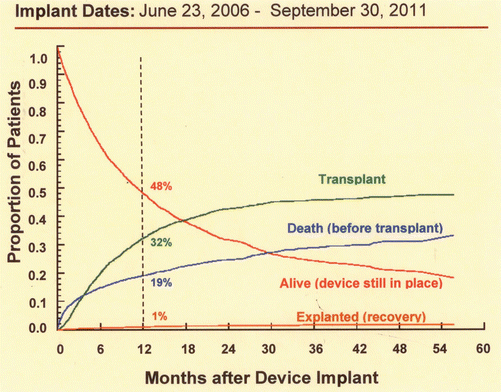
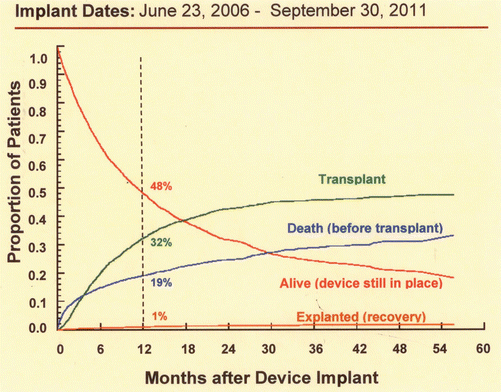
Fig. 4.3
Competing outcomes depiction for primary continuous flow device implant as a bridge to transplantation. The sum of the percentages of any time point equals 100 %. The depicted percentage is referred to the 12-month time point (Permission from: Interagency Registry for Mechanically Assisted Circulatory Support, National Heart Lung and Blood Institute. Contract Award HHSN268201100025C. http://www.uab.edu/intermacs. 5/12/15)
It has been well demonstrated that cardiac retransplantation for early graft failure or intractable acute cardiac rejection has poor survival (Fig. 4.4) [13] and has been mostly discouraged. However, retransplantation for the indications of non-specific graft failure or coronary allograft vasculopathy has survival that is very similar to that of patients undergoing primary cardiac transplantation (Fig. 4.5) [14], although contrary information has suggested [15] that long-term survival after retransplantation may not be universally similar to that of primary transplantation.
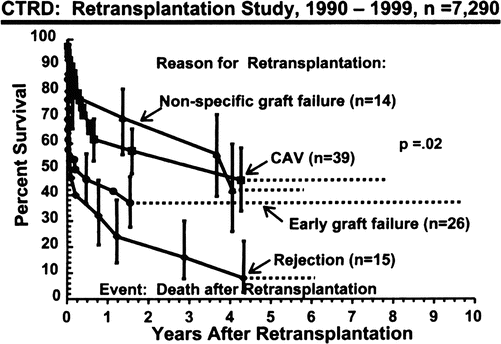
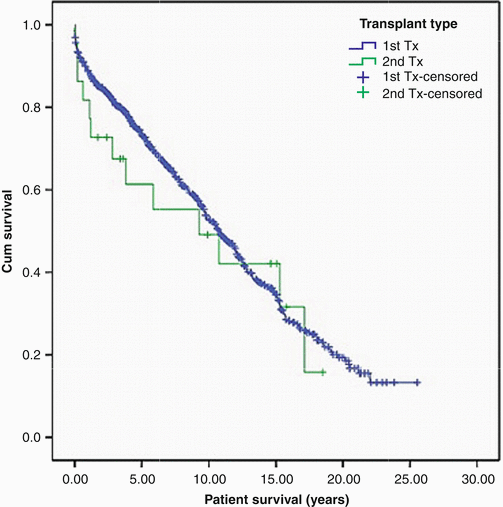
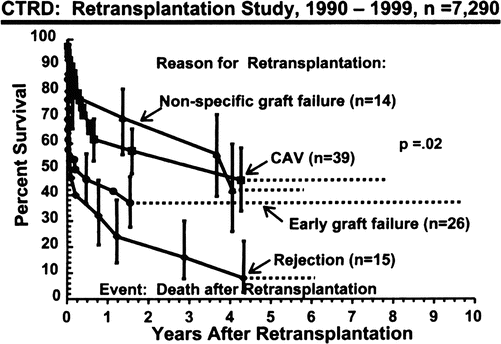
Fig. 4.4
Actuarial survival (Kaplan-Meier) stratified by reason for cardiac retransplantation. Vertical bars represent the standard error (Radovancevic et al. [13] with permission)
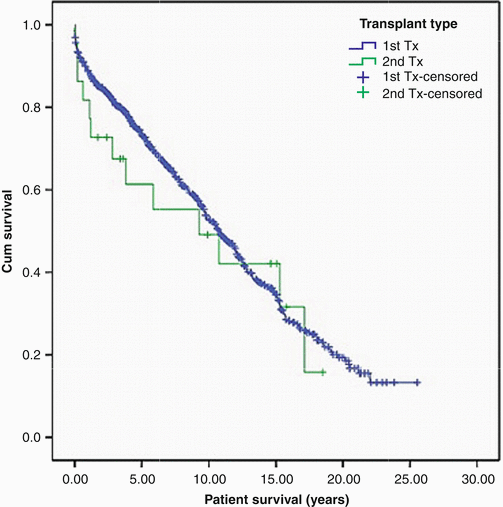
Fig. 4.5
Patient survival: 818 patients undergoing primary transplantation are shown in blue, and 23 patients undergoing elective retransplantation are shown in green. Tx transplantation (Copeland et al. [14] with permission)
In the United States, patients are listed for cardiac transplantation with United Network for Organ Sharing (UNOS) which is responsible for the donor organ distribution system. The algorithm for allocation of donor hearts is based on the severity of illness and medical urgency reflected by the need for interventions such as mechanical circulatory support, intra-aortic balloon pump, extracorporeal membrane oxygenation, mechanical ventilation and high dose inotropic drugs. A similar allocation system exists for pediatric patients with the inclusion of the presence in patients less than 6 months of age who are demonstrating reactive pulmonary hypertension [16]. Similar organ allocation systems exist around the world.
Cardiac Donor Evaluation
Cardiac transplantation is possible only through unselfish organ donation by families at the worst possible time of their lives. The procurement of hearts from heart-beating donors is predicated upon the worldwide acceptance of brain death criteria. The volume of cardiac transplantation is limited by donor heart availability and there will never be enough donor hearts available to fill the need that currently exists. The immutable shortage persists despite intensive educational programs, “presumed consent” laws in some European countries and “required request” protocols in the United States which mandate that hospitals approach families of brain dead patients regarding organ donation.
Effects of Brain Death on Cardiac Function
Brain death is a hostile environment for the heart and consequent donor heart dysfunction may preclude use of the donor heart or contribute to the 20 % incidence of primary graft failure following cardiac transplantation. The causes of donor heart dysfunction can be conceptualized into two phases: those occurring with the Cushing response and those following dissipation of the Cushing response (Fig. 4.6).
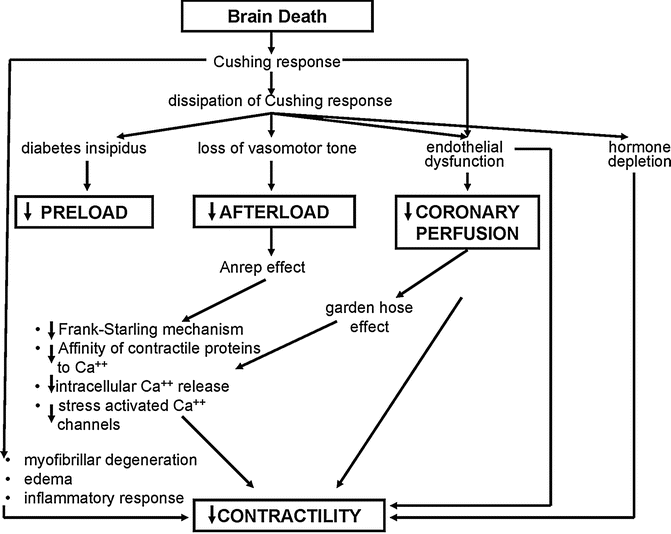
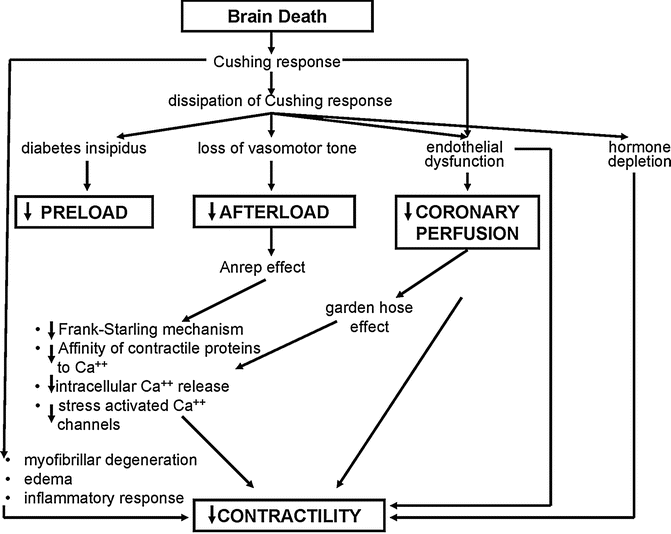
Fig. 4.6
Pathophysiological effects of brain death on the cardiovascular system following brain death (Based in part on Szabo) [17]
The Cushing response is a “catecholamine storm” inducing hypertension, tachycardia, intense vasoconstriction, and an increase in myocardial oxygen demand, resulting in myocardial ischemia. The injury to the myocardium results in a phenomenon known as myofibrillar degeneration (which is also known as contraction band necrosis or coagulative myocytolysis) which is distinct from the coagulation necrosis seen in acute myocardial infarction. Myofibrillar degeneration is characterized histologically by the death of myofibers in a hypercontracted state with obvious contraction bands. The other important histological feature that may be seen is a mononuclear cellular infiltrate, which is evidence of the intense inflammatory response known to be activated with brain death. There are experimental studies [18, 19] that have detected upregulation of cytokines, chemokines, adhesion molecules and immunoregulatory molecules after brain death, which occur along with leukocyte activation. All of these likely have a role in endothelial cell injury and activation. When this period of intense sympathetic activity is dissipated, there is loss of sympathetic tone with a massive reduction in systemic vascular resistance contributing to the second phase of potential myocardial injury. This phase of injury can be conceptualized as abnormal loading conditions and coronary perfusion [20]. Intervention and donor management in this phase, offers some hope of retrieving donor hearts that would ordinarily have been lost. There is experimental evidence that if coronary perfusion pressure is decoupled from aortic pressure and returned to pre-brain death levels, coronary blood flow and myocardial contractility can also be restored [20]. The exact mechanisms of how the loading conditions and coronary perfusion down-regulate myocardial contractility are not completely clarified, but may occur through the Frank-Starling mechanism or other less well-characterized mechanisms such as the Anrep effect [21, 22] (maintenance of optimal stroke work over a range of afterload conditions controlled through cellular mechanism) and the garden hose effect [23, 24], which has been described in isolated heart preparations. This effect refers to the relationship between increased coronary perfusion pressure and increased contractility by direct intramyocardial vessel stretch and this mechanism may possibly come into play at a level of coronary perfusion pressure below which autoregulation is no longer operational.
Screening for Cardiac Donation
Following declaration of brain death and obtaining consent, the process of determining suitability for cardiac donation is allowed to proceed. The screening process [25] (Table 4.5) has a number of goals. The general organ donation screening is to prevent the transmission of malignancies or the transmission of viral disease such as hepatitis B and C and HIV infection. Although it is generally considered that transplantation of hearts from donors who have died of primary brain tumors is safe (based on the very low incidence of extra neural spread of these tumors), transmission of primary brain tumors to a recipient has indeed been reported and for that reason organs from donors with CNS tumors of high grade malignancy should probably not be used [26]. The current recommendation [27] for the use of hearts from donors with severe infection is that they can be used provided [1] the donor infection is community acquired and death occurs within 96 h of the onset of the infection [2], repeat blood culture before organ procurement are negative [3], pathogen-specific antimicrobial therapy is administered to the donor [4], donor myocardial function is normal [5], there is no evidence of endocarditis by direct inspection of the donor heart. Exclusion of coronary artery disease (CAD) is necessary in cardiac donor males older than 40 years and females older that 45 years, although these age criteria may vary depending on the presence or absence of risk factors for CAD.
Table 4.5
Screening criteria for cardiac donation
General organ donation screening |
Absence of infection |
Hepatitis B and C and HIV |
Negative blood cultures |
Psychosocial/lifestyle screening |
Absence of malignancy (exluding primary CNS tumors) |
Screening for coronary disease |
Age usually less than 55 |
Coronary angiography if possible: |
Men >40 years |
Women >45 years |
No pathologic Q waves on ECG |
No history of insulin-requiring diabetes |
No other prior cardiac history |
Screening for cardiac function |
No requirement for high-dose inotropic support (after volume replacement) |
No prolonged resuscitation |
Normal echocardiogram (mild segmental wall motion or mitral prolapse not a contraindication, expanded criteria using moderate wall motion abnormalities if normal coronaries) |
The determination of the adequacy of cardiac function should be delayed until hemodynamic stability has been obtained. Many donors have deranged loading conditions due to the very low systemic vascular resistance together with a low preload associated with the aggressive diuresis often used to control cerebral edema prior to progression to brain death and/or diabetes insipidus following brain death. As a consequence, these donor are often on high-dose adrenergic support, which, with optimization of loading conditions, can be rapidly weaned. At that stage, the echocardiogram has become the most useful test to evaluate left and right ventricular systolic function.
A number of biomarkers have been investigated as a means of discriminating donor hearts that should or should not be used for transplantation because of the possibility of post-transplant graft failure. Biomarkers that have been investigated include cardiac-specific troponins (cTnI and cTnT), TNF-α, IL-6, procalcitonin and B-Type naturetic peptide. These molecules reflect different aspects of the impact of brain death such as myocardial injury, myocardial wall stress and the pro-inflammatory environment of brain death but none have been found to be sensitive or specific enough to be useful [28].
Factors Effecting Post-transplant Donor Heart Function
Early graft failure represents an important cause of morbidity and mortality after cardiac transplantation and is impacted by a number of donor heart factors. However, when making a decision about the suitability of a particular donor these factors cannot be considered in isolation since they are all inextricably interrelated. Furthermore, the use of a donor heart with factors that may impair post-transplant function must always be considered in the context of the condition of the recipient and the urgency of transplantation.
A relationship exists between older donor age and the probability of graft failure and this risk is further exacerbated by increasing ischemic time (Fig. 4.7) [29]. This effect is no doubt related to the overall decline in myocardial reserves associated with increase in age. Even though this information is from an earlier era in transplantation, the same relationship between ischemic time and donor age and it’s impact on survival after cardiac transplantation has been consistently demonstrated [30]. In general, the ischemic time should be less than 5.5 h. Excessive inotropic support (dopamine at a dose of 20 mcg/kg/min or similar doses of other adrenergic agents) contraindicates the use of the heart if these inotropic drugs are required despite optimization of filling pressures. A discrete wall motion abnormality on echocardiography increases the risk of early graft failure and such a donor heart should not be used [31]. The effect of discrete wall motion abnormalities becomes increasingly pronounced with older donor age (Fig. 4.8) [31]. The development of severe concentric left ventricular hypertrophy following cardiac transplantation is a clear independent risk factor for cardiovascular and all cause mortality [32, 33], and this hypertrophy may develop for a number of reasons including post-transplant hypertension and immunosuppression. There is compelling evidence that commencing the post-transplant course with a hypertrophied donor heart also negatively impacts survival. A donor heart left ventricular wall thickness of greater than 1.4 cm is associated with reduced survival and this has been demonstrated both in a univariate analysis (Fig. 4.9) and in a multivariable model [34]. Furthermore, the impact of donor left ventricular hypertrophy on survival worsens with increasing donor age and increasing ischemic time [35].
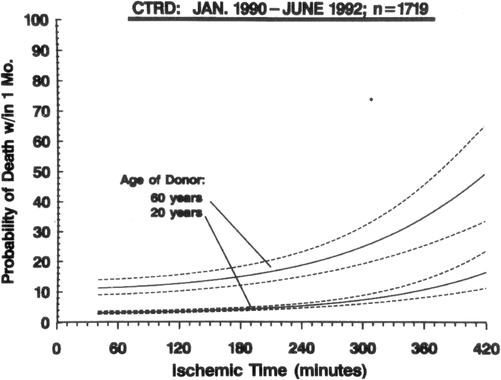
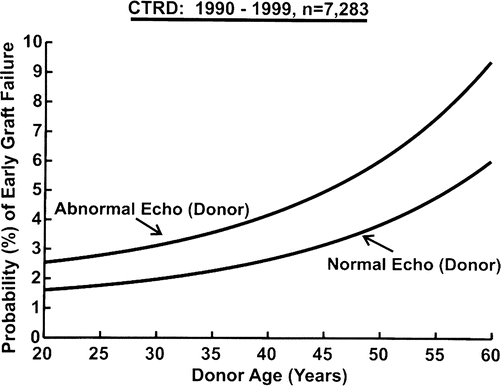
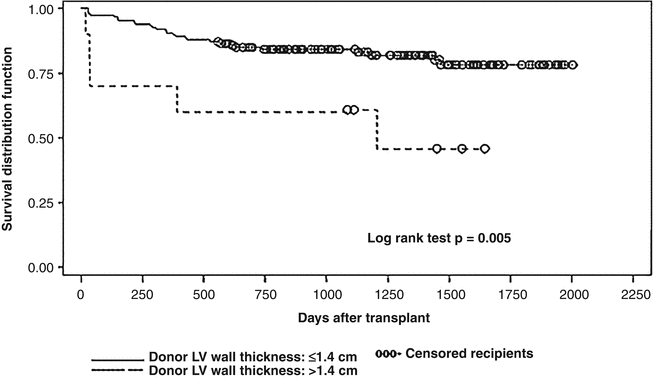
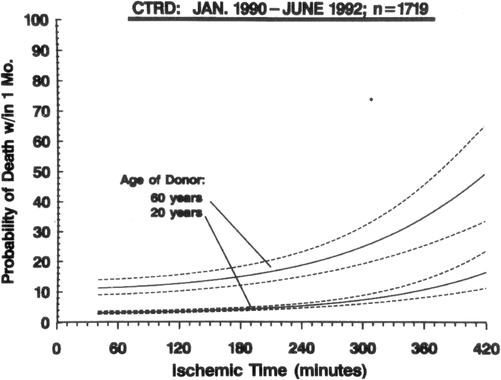
Fig. 4.7
Nomogram from donor/recipient multivariable analysis in hazard function domain depicts varying ischemic time for two donors, age 20 and 60 years. Equation is solved for 50-year-old male recipient who is not on a ventilator, not on a ventricular assist device, has not undergone previous sternotomy, and has a pulmonary vascular resistance of 2.2 Wood Units and male donor who is not diabetic, not receiving inotropic support, did not die of a cardiac arrest, and has no diffuse wall motion abnormalities. Dashed lines represent 70 % confidence limits around parametric curves (Young et al. [29] with permission)
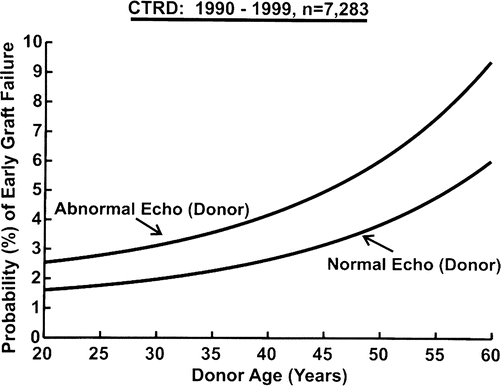
Fig. 4.8
Nomogram from Cardiac Transplant Research Database (CTRD) multivariable analysis depicting the effect of donor age and left ventricular function by echocardiography (echo) on fatal early graft failure (Young et al. [31] with permission)
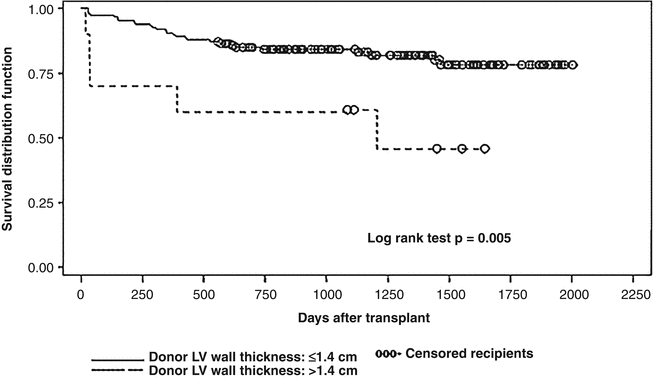
Fig. 4.9
Comparison of survival in recipients of donor hearts with left ventricular wall thickness of <1.4 cm versus >1.4 cm (log-rank test) (Kuppahally et al. [34] with permission)
Donor hearts with potential toxicity have been considered for transplantation. Cocaine abuse has direct effects on the heart, which include vasoconstriction, endothelial dysfunction, and myocardial toxicity. These effects have been principally related to the intravenous use of cocaine and hearts from such donors are currently regarded as unsuitable for transplantation. However, donors with a history of non-intravenous cocaine abuse who have normal left ventricular systolic function with no evidence of left ventricular hypertrophy are currently considered suitable [36].
There are direct toxic effects of alcohol on the myocardium and recipients who have received hearts from donors with a history of significant alcohol abuse have inferior survival. Hence, if a strong history of chronic alcohol abuse can be elicited, currently hearts from these donors are not used for cardiac transplantation [37]. However, it should be mentioned that there is not unanimity in the information on this issue and there is evidence [38] that suggests the exact opposite, that donor chronic alcoholism may, in fact, have a protective effect on donor hearts. Because of the finding that this protective effect may translate into superior recipient survival to that over recipients receiving hearts from non-alcoholic donors, it has been suggested that it is safe to use donor hearts regardless of a history of alcoholism.
Use of hearts from donors dying from carbon monoxide poisoning is still contentious [39]. However, use of hearts from such donors is probably safe, provided the electrocardiogram and echocardiogram are normal with minimal elevation of cardiac markers, and minimal inotropic requirements.
Transplantation of hearts from hepatitis C positive donors to hepatitis C negative recipients is associated with substantially inferior survival and this strategy cannot be recommended. However, transplantation of hearts from hepatitis C positive donors to hepatitis C recipients may be considered.
Special Situations
In order to increase the number of available donor hearts, a number of donor conditions may still be considered compatible with successful transplantation.
Donor CAD is not necessarily a contraindication to transplantation and coronary bypass surgery has been utilized on donor hearts. This concept of matching “recipients who would not ordinarily meet criteria for cardiac transplantation or retransplantation” to “marginal” donor hearts has given rise to the concept of the “alternate list” [40]. Survival of recipients with donors hearts that have undergone coronary bypass surgery appears to be very acceptable [41].
Although supplying only a very small number of donor hearts, the “domino procedure” (the use of a heart from a heart/lung transplant recipient) appears to provide just as good survival as the use of cadaveric donors [42].
Donor-Recipient Matching
Donor–recipient size matching should not be considered in isolation from other factors such as donor age and acuity of the transplant procedure. The use of hearts from donors whose body weight is no greater that 30 % below that of the recipient is generally considered safe. The heart from a male donor of average weight (70 kg) may be considered for any recipient of equivalent or greater weight, provided donor age is taken into account. Undersizing with older donors age is clearly associated with an increased risk [43], and certainly this information would argue against the use of small hearts from an older donor to “rescue” a critically ill recipient.
Sensitization due to the development of antibodies directed against human leukocyte antigens (HLA) is a major problem for heart transplantation and is associated with an increased risk for hyperacute rejection, acute rejection (cellular and antibody mediated), graft dysfunction, graft loss and development of coronary allograft vasculopathy. Sensitization may occur because of previous pregnancy, transfusion, previous transplantation and the use of homograft tissue that may be employed in correction of, for example, congenital heart disease. Desensitization strategies have been used with mixed results and the removal of donor specific antibodies at the time of transplantation does not necessarily prevent a memory immune response. There are a number of factors that dictate the impact of donor specific HLA antibodies on the transplanted heart (Fig. 4.10). However, there seems to be little doubt that if HLA donor specific antibodies activate complement that it is potentially very damaging to the transplanted heart. There are a number of ways of avoiding a cardiac transplant in the presence of donor specific antibodies. The complement-dependent cytotoxicity (CDC) crossmatch is the classic test which involves mixing potential recipient serum with donor T and B lymphocytes together in the presence of complement. The T-cell crossmatch generally reflects antibodies to HLA class I (expressed on all nucleated cells) and the B-cell crossmatch reflects HLA class I and II (class II expression is restricted to dendritic cells, macrophages and B-cells). There has been a move away from the CDC crossmatch to flow cytometry crossmatching which is more sensitive for detecting donor specific antibodies compared with the CDC crossmatch. A positive flow crossmatch in the presence of a negative CDC crossmatch suggests a non-complement fixing antibody, a non-HLA antibody or a low level antibody [45]. The development of solid phase matrices (beads) coated with HLA antigens has allowed the detection and identification of HLA-specific antibodies in a potential recipient. These HLA-specific antibodies can then be compared with the HLA typing of the prospective donor, a strategy known as the virtual crossmatch. Virtual crossmatching is of particular importance to cardiac transplantation where donor lymphocytes may not be available for a CDC or flow crossmatch because of time constraints and the donor being at a remote location. The accuracy of virtual crossmatching and its ability to increase the access of sensitized patients awaiting heart transplantation to potential donors has been demonstrated [46–48].
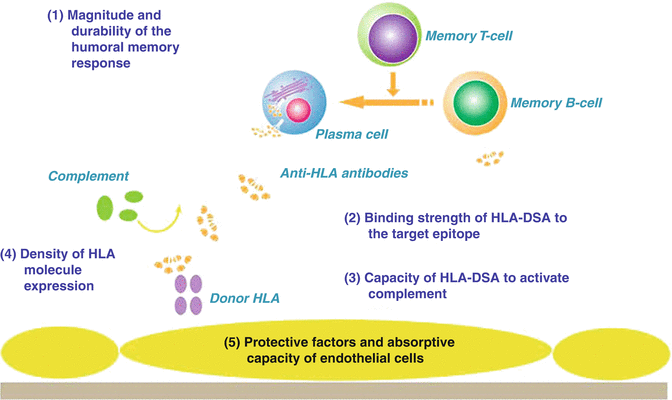
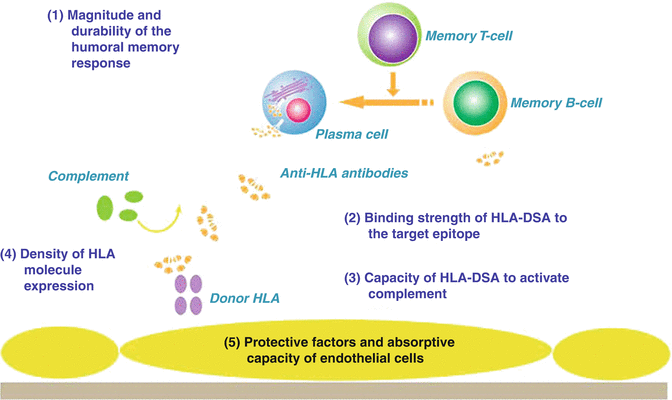
Fig. 4.10
Many factors on different levels might contribute to the pathogenicity of donor-specific HLA antibodies (HLA-DSAs): (1) the magnitude and durability of the humoral memory response upon rechallenge; (2) the binding strength of the HLA-DSA to the recognized epitope. Potentially, there might be a link between the immunogenicity of HLA epitopes evoking an antibody response and the magnitude of the immunologic memory response upon rechallenge; (3) the capacity of the HLA-DSA to activate the complement system; (4) the density of HLA molecules on the endothelial cell surface; (5) protective factors and the ‘absorptive capacity’ of endothelial cells that might modulate the clinical impact of HLA-DSA (i.e. accommodation) (Amico et al. [44] with permission)
Cardiac Transplant Procedure
Myocardial Preservation
Myocardial preservation during cardiac transplantation is vitally important. Biochemical derangements induced during the ischemic process may contribute to primary graft failure, and preservation strategies that improve post-transplant graft function may allow longer ischemic times and the use of marginal donor hearts, with greater confidence that post-transplant function will be satisfactory. Biochemical processes involved in myocardial preservation are complex, but the primary methods of achieving cellular and functional integrity of the myocardium are through hypothermia and mechanical arrest of the heart. The role of hypothermia is based on the observation that in mammalian enzyme systems there is an approximately 50 % reduction in enzymatic reactions for every 10 °C decline in cardiac temperature, which decreases but does not eliminate cellular activity completely [49]. Even though the heart is mechanically arrested, ATP is still being consumed at a low level to allow breaking of actin-myosin cross-brides. If ATP depletion continues below a threshold level, irreversible contracture will occur. Although the myocardium can use stored glycogen to produce ATP by anaerobic glycolysis to maintain an ATP level above the critical threshold, once contracture commences ATP consumption markedly increases. The maintenance of ion homeostasis is also an important requirement of preservation. Even though with hypothermia Na+/K+ ATPase activity is markedly reduced, passive ion movement will still occur down a concentration gradient. As a result, intracellular H+ ions are exchanged for extracellular Na+ ions, and Ca2+ ions are also exchanged for Na+. This produces an increased intracellular solute accumulation with water entering the cell down an osmotic gradient, and this intracellular edema may result in disruption of structural integrity. It is the accumulation of Ca2+ in the cytoplasm that may, on reperfusion, result in hypercontracture [50].
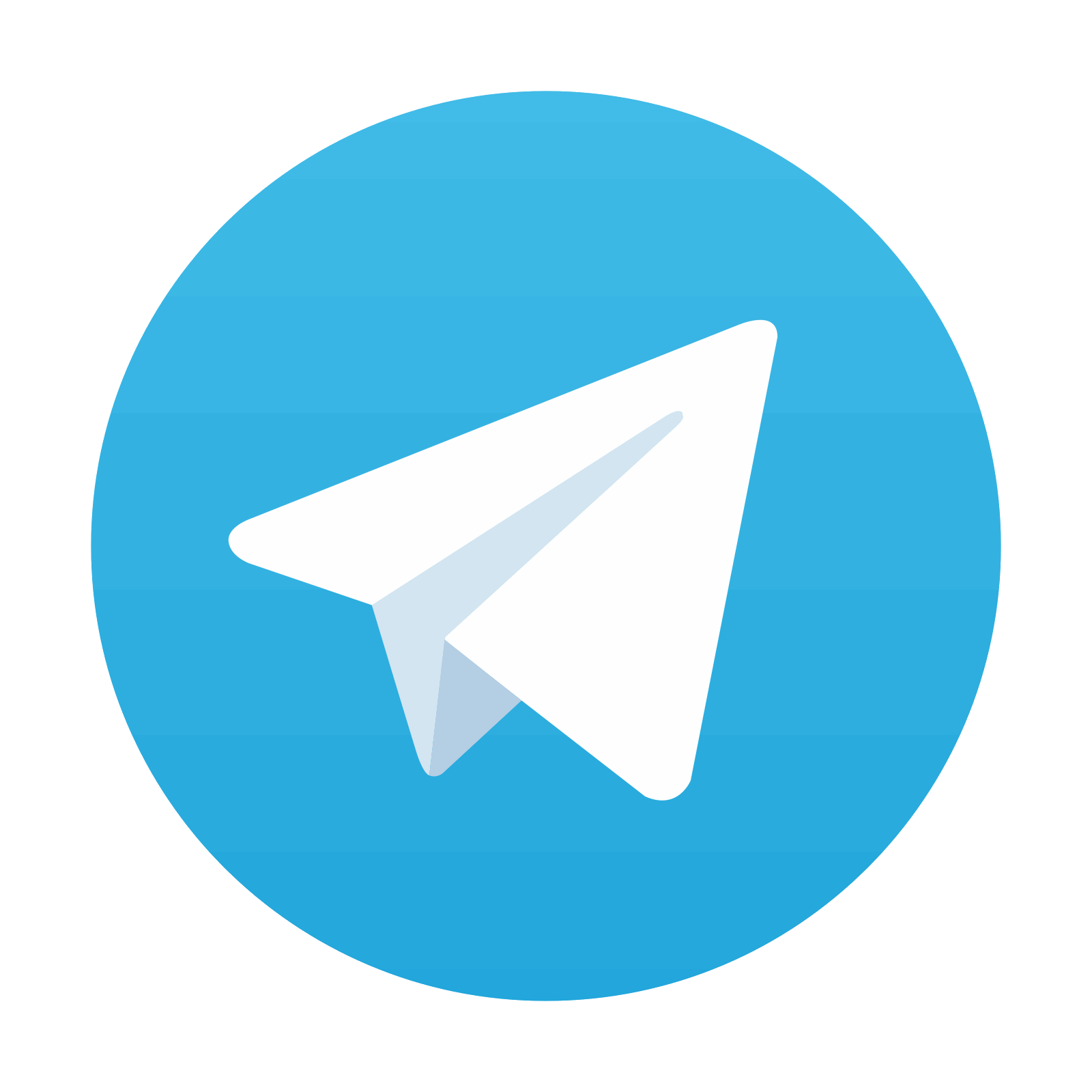
Stay updated, free articles. Join our Telegram channel

Full access? Get Clinical Tree
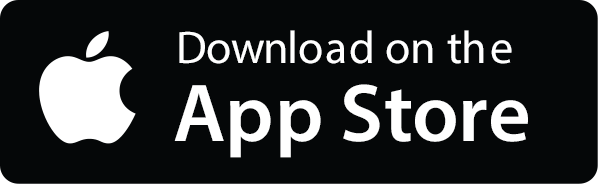
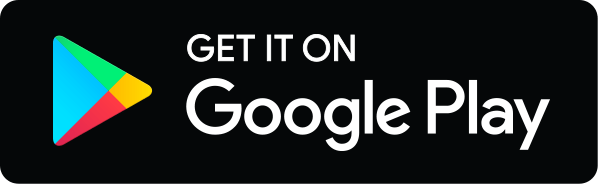