INTRODUCTION
- ▪
The optimal treatment for patients with end-stage organ failure is transplantation. This is especially true for patients who are candidates for lung, heart, and heart-lung transplantation because no long-term life support system such as dialysis is available.
- ▪
The study of transplantation has played a critical role in advancements in our understanding of immunology.
- ▪
Organ donors and recipients are genetically distinct. This factor results in a genetically encoded immunologic barrier to transplantation that was first recognized in experiments performed in the early 20th century.
- ▪
The first successful kidney transplant was performed in 1954 between identical twins. However, the true implementation of transplantation as a reasonable therapy for end-stage organ failure awaited advancements in understanding the immunologic basis of allograft rejection and advancements in immunosuppressive therapy.
- ▪
The surgical techniques for kidney transplantation and heart transplantation were developed in the 1950s and 1960s, but the success of these organ transplants were dismal due to the severe rejection that often accompanied these procedures.
- ▪
For heart transplant especially, the ultimate graft failure and death of most patients led to a feeling that the fledgling transplant enterprise was a failure and could offer little hope for anyone. This was best depicted in a feature article that appeared in the September 17, 1971 issue of LIFE . The article describes “The Tragic Record of Heart Transplants: A New Report on an Era of Medical Failure” ( Fig. 41-1 ).
Figure 41-1
A feature article that appeared in the September 17, 1971 issue of LIFE . The article describes “The Tragic Record of Heart Transplants: A new report on an era of medical failure.”
- ▪
Clearly, the rapid advancements in surgery could not compensate for the poor and incomplete understanding of the allograft-directed immune responses and how to deal with them. Fortunately, a few years later Dr. Borel, a biochemist working at Sandoz in Switzerland, discovered a novel fungal derivative drug with immunosuppressive properties (cyclosporine A [CsA]) that would eventually revolutionize the field of transplantation.
- ▪
The field of clinical transplantation developed alongside clinical immunology.
- ▪
The rejection of allograft tissue was found to be a result of an active immunologic process in which antigens of the allograft are processed and an acquired immune response developed with multiple effectors that evolve to destroy the transplant.
- ▪
This knowledge was augmented by the finding that the primary target for the allograft-directed immune response was the major histocompatibility complex (MHC). Genes in this region encode dominant transplant antigens that were first identified serologically and defined as human leukocyte antigens (HLA).
- ▪
These HLA antigens were not created as transplant antigens by nature and were ultimately found to be responsible for self-restriction of immunologic responses. These fundamental observations represent the cornerstone of the scientific basis for the practice of transplantation.
- ▪
Further developments in immunology have revealed a high degree of complexity of the immune response to allografts and cellular events that lead to the loss or acceptance of an organ transplant. These events are shown in Figure 41-2A and B .
Figure 41-2
A , The initiation of acute rejection in an unmodified allograft lies in events related to procurement and surgery. Once the organ is removed, perfused, and transplanted, cytokines such as TNF-α, IL-1a and IL-6 are generated and activate the endothelium of the allograft. This allows infiltrating T-cells to bind and migrate from the endothelium to the interstitium of the allograft ( inset photo ). These migrating T cells move into the host lymphoid tissues and process alloantigens that result in T cell activation and allograft rejection, as shown in B . B shows that resting dendritic cells (DC) or antigen presenting cells (APCs) become activated in the cytokine-rich environment of an ischemic-reperfused organ. This activates the DC and allows it to interact directly with the activated T cells that have migrated through the endothelium. This allows interaction of the T-cell receptor (TCR) with the MHC class II antigen presenting machinery on the DC/APC. This is called Signal 1. With signal 1 only, the T cell would receive incomplete activation and die. However, the DC contains other molecules called costimulatory molecules (CD80/86, CD40/CD40L) that are responsible for delivering a full activation signal to the T cell (Signal 2). Once activated, T cells produce cytokines such as interleukin-2 (IL-2), IL-15, and interferon-γ (IFN-γ) that drive T-cell proliferation and cytotoxic effector functions (Signal 3).
HISTOCOMPATIBILITY AND ALLORECOGNITION
- ▪
Humans and all higher animals evolved histocompatibility molecules (HLA system in humans) with matching receptors to allow them to distinguish their cells and tissues as “self.”
- ▪
These molecules represent a system of diversification and amplification that allows different organisms among the same species to preserve individual immunologic integrity.
- ▪
Clearly, allotypic differences between donor and recipients of transplanted tissues can simulate immune responses that are characterized as rejection. This arises, in large part, because of the extreme polymorphism of the HLA system and the role of these molecules in antigen recognition.
- ▪
Although other minor histocompatibility differences have been identified in humans, the MHC is the major target for allotypic responses in transplant rejecton.
T-CELL ACTIVATION AND ALLOGRAFT Rejection
- ▪
The structure of the human MHC class I and class II molecules is shown in Figure 41-3 .
Figure 41-3
This figure is a schematic diagram of the human MHC class I and class II molecules. MHC class I consist of three a chains bound to β2 microglobulin (not membrane bound). Foreign peptides are bound in the peptide groove. This APC then interacts with CD8+ T cells from the host to allow activation of peptide-specific CD8 response (cytotoxicity). The major histocompatibility complex (MHC) class II consists of two alpha chains and two beta chains both tethered to the cell membrane. Peptides bound to the MHC class II interact with the individual CD4 cells only. Activation of CD4 T cells interacting with this peptide results in helper CD4 T-cell functions (regulation of T-cell effectors, activation of B cells and initiation of antibody production by B cells).
- ▪
MHC class I proteins
- ○
Cell-surface glycoproteins that are highly polymorphic and composed of a heavy chain and a less variable light chain (b2 microglobulin).
- ○
MHC class 1 proteins are expressed on most nucleated cells and interact exclusively with CD8+ T-cells through the T-cell receptor (TCR) complex.
- ○
The interaction of CD8+ T cells with the MHC class I peptide complex is “self-restricted,” meaning that CD8+ T cells from another individual would not recognize this individual’s MHC class I peptide complex. When CD8+ T cells interact with sel-MHC class I/peptide complex, the CD8+ T cell becomes activated and generates effector CD8+ T cells directed at this target (see Fig. 41-3 ).
- ○
- ▪
MHC class II proteins
- ○
Consist of two membrane anchored glycoproteins (a chain approximately 35 kD; b-chain, approximately 28 kD). The tissue distribution of MHC class II molecules is much more restricted than that of MHC class I.
- ○
MHC class II is constitutively expressed on B-lymphocytes and dendritic cells, which have very specialized functions as antigen-presenting cells (APCs). MHC class II/peptide-bearing cells generally interact with CD4+ T cells only.
- ○
Again, these interactions are self-restricted and result in the activation of the CD4+ T cell with designated effector functions aimed at the presented peptide (see Fig. 41-3 ).
- ○
ALLOANTIGEN RECOGNITION AND EFFECTOR FUNCTIONS
- ▪
Because the graft is infiltrated by T cells, alloantigen recognition can occur in one of two ways: direct allorecognition and indirect allorecognition.
- ▪
Direct allorecognition:
- ○
Is of particular importance for lung and heart lung allografts because this is the direct interaction of infiltrating T cells with allogenic MHC molecules present on the dendritic cells present in the allograft and adjacent lymphoid tissue.
- ○
The frequency of alloreactive lymphocytes stimulated through this process is high and likely accounts for most cases of early allograft rejection.
- ○
Although direct allorecognition would appear to violate the “self-restriction” principle of T-cell activation, it is likely that the TCR of infiltrating lymphocytes can discriminate between subtle differences in MHC structure and peptides binding in their grooves. Because the infiltrating lymphocytes are not from naïve animals, there is likely a large repertoire of T-cell memory that constitutes infiltrating cells that have the capacity to become activated more easily and can result in allograft directed T-cell responses. This is shown in Figure 41-4 .
Figure 41-4
Allorecognition pathways for allograft infiltrating lymphocytes. T cells infiltrating the allograft can be activated by direct antigen recognition (interaction of host T cells with alloantigen present on antigen-presenting cells [APCs] present in the allograft). This is likely to be associated with early acute rejection (AR). Second, long-term indirect allorecognition can occur (interaction of self APCs processing donor antigens and presenting to self–T cells). This is a more traditional form of immune activation and results in more chronic immune responses to the allograft.
- ○
This is why the achievement of tolerance in humans is much more difficult than in inbred mouse strains that have limited pathogen exposure and T-cell repertories.
- ○
- ▪
Indirect allorecognition:
- ○
Is a more traditional process of T-cell activation.
- ○
In allogenic transplantation, alloantigens of the graft are processed by self-MHC–bearing APCs and presented to self T cells. Thus effector T cells are generated and respond to the allograft in a more chronic manner.
- ○
The importance of indirect presentation is underscored by the intense immune responses generated by skin grafts from MHC class II–deficient mice transplanted into normal mice. Rejection occurs as a result of indirect presentation only and results in long term memory.
- ○
In human transplantation, the indirect pathway is likely responsible for chronic allograft rejection and stimulation of antibody responses to the allograft. Established immune responses initiated via the indirect pathway are long lasting and difficult to suppress. This is summarized in Figure 41-4 .
- ○
COSTIMULATION IN T-CELL ACTIVATION
- ▪
The fate of T cells after engagement of the TCR with cognate MHC/peptide complex varies widely. Outcomes include activation, proliferation, effector functions, apoptosis, anergy and evolution to memory cells.
- ▪
Clearly, the various outcomes of T-cell activation depend on costimulation and soluble cytokines. This has been an area of great interest in transplantation because manipulation of costimulatory molecule interaction with T cells has the potential to delete alloreactive T-cell clones and mute alloreactive T-cell responses.
- ▪
Many experiments have detailed the importance of costimulation. If T cells engage antigen through the TCR/MHC peptide complex alone (Signal 1), T cells either die or become anergic ( Fig. 41-5 ).
Figure 41-5
T cells interacting with cognate receptors on APCs can result in different “fates” for the T cell. Signal 1 only (TCR/MHC) results in apoptosis/anergy of the T cell. A full “immunizing” signal of the T-cell is seen when Signal 1 (TCR/MHC) and Signal 2 (CD80/86-CD28) occur together. This process results in cell proliferation, differentiation and effector functions. Finally, when T cells express CTLA4 as a costimulatory molecule with TCR/MHC interactions, cell cycle arrest and apoptosis can occur. CTLA4 conveys a negative, regulatory signal to the T cell when it interacts with CD80/86 compared with the positive signal transmitted by CD28/CD80/86 interactions.
- ▪
Costimulation occurs through numerous molecules and cognate receptors on T cells and APCs. However, of considerable importance is the CD40/CD40L(CD154) and CD28/CD80/86 molecules.
- ▪
Once the TCR/MHC-peptide complex is engaged, engagment of costimulatory molecules (Signal 2) results in a full “immunizing” signal to the T cell with resultant activation.
- ▪
Engagement of CD40/CD40L results in the upreguation of CD80/86 on APCs, thus allowing for more interactions with CD28 on T cells. This interaction results in increased cytokine synthesis and cell proliferation with cytotoxic effector functions.
- ▪
After initial immunizing signals through the CD28/CD80/86 pathway, T cells begin to express another costimulatory molecule (CTLA4 or CD152).
- ▪
This molecule has 100-fold higher avidity for CD80/86 and results in termination of T-cell effector responses. This negative signaling costimulatory pathway has also shed light on how manipulation of this regulatory pathway could be used to regulate T-cell immune responses to allografts.
- ▪
Multiple experimental data has shown that blockade of the CD28/CD80/86 pathway with the synthetic peptide CTLA4-Ig results in prolonged allograft survival and termination of alloimmune responses.
- ▪
Recent data in human kidney transplantation have shown that a genetically modified CTLA-4 Ig (Belatacept) can prevent allograft rejection and result in long-term rejection-free survival.
- ▪
However, there is concern that costimulatory blockade may not be efficacious in the modification of patients with T-cell memory. The various fates of T cells after activation are shown in Figure 41-5 .
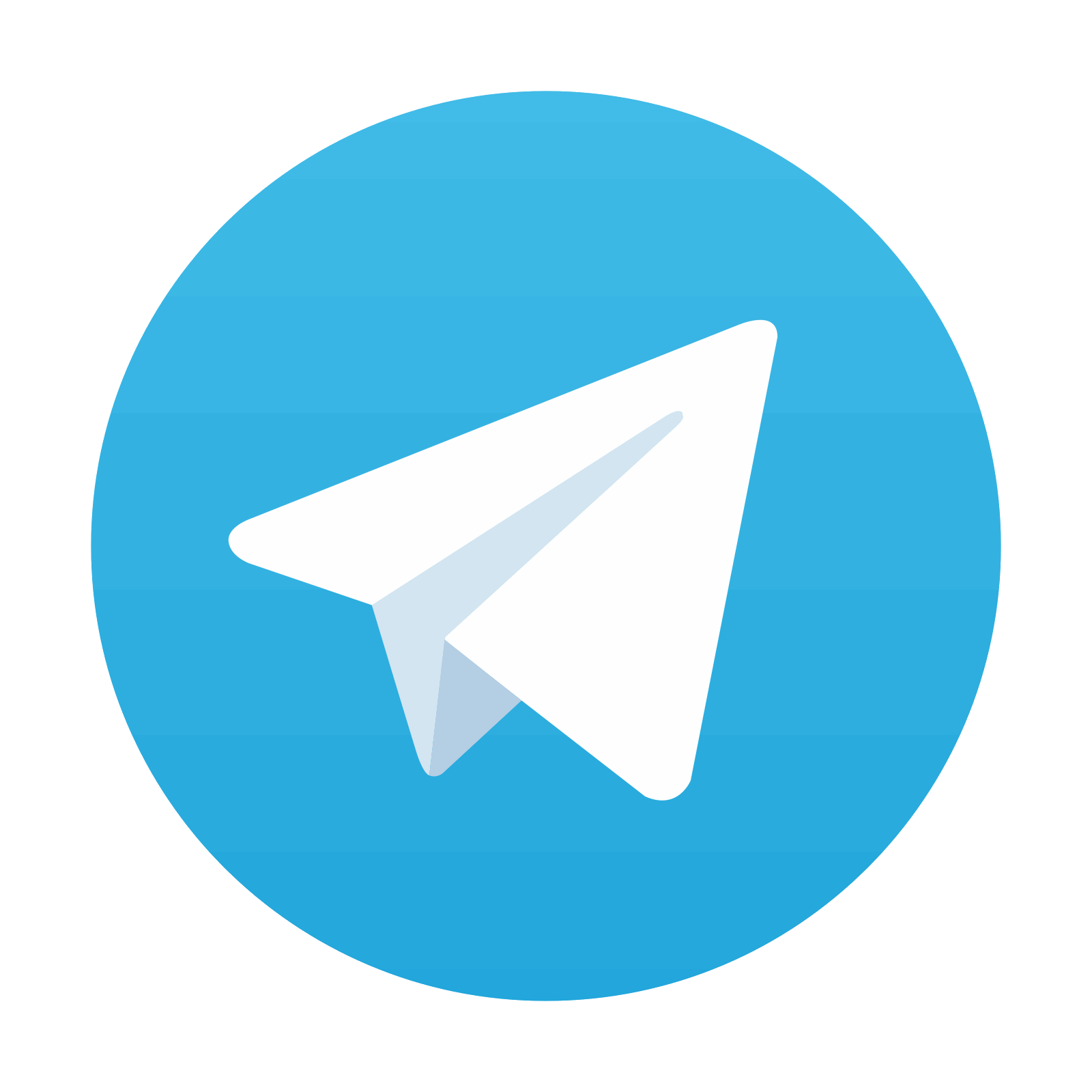
Stay updated, free articles. Join our Telegram channel

Full access? Get Clinical Tree
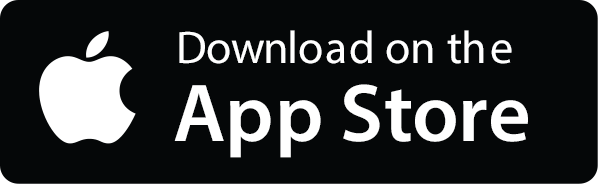
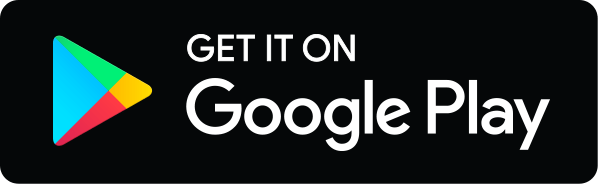