Background
In patients with chronic aortic regurgitation (AR), systolic wall stress and volume overload affects left ventricular (LV) systolic function and remodeling. The aim of this study was to assess transmural rearrangements of myocardial deformation to preserve LV ejection performances using speckle-tracking echocardiography in patients with chronic AR.
Methods
Ninety patients with AR were enrolled. On LV short-axis images, total, inner, and outer radial strain and circumferential strain at the inner, mid, and outer layers were calculated. On apical four-chamber images, endocardial longitudinal strain was calculated. End-systolic wall stresses were calculated using previous methods.
Results
AR severities were classified as moderate in 31 patients, severe and preserved LV ejection fraction (LVEF) (≥50%) in 42 patients, and severe and reduced LVEF (<50%) in 17 patients. Longitudinal strain was decreased even in the moderate AR group, despite normal end-systolic wall stress. Inner radial strain progressively decreased with increasing end-systolic wall stress, whereas outer radial strain in the moderate and severe AR and preserved LVEF groups was higher than in the control group. Consequently, total radial strain was preserved even in the severe AR and preserved LVEF groups with increased end-systolic wall stress. Similarly, despite reduced inner circumferential strain, outer circumferential strain was higher in the severe AR and preserved LVEF group than in the control group. All strain parameters were lower in the severe AR and reduced LVEF group with dramatically increased end-systolic wall stress than in other groups.
Conclusions
Transmural strain analysis revealed that subendocardial dysfunction accompanied by increased wall thickening at the subepicardium may be a compensatory mechanism of wall thickening to preserve LVEF in patients with chronic AR.
In chronic aortic regurgitation (AR), adaptation to increased preload and afterload results in left ventricular (LV) enlargement and increased myocardial mass. LV eccentric hypertrophy inhibits the increase of wall stress, and patients with chronic AR remain asymptomatic with preserved LV ejection fractions (LVEFs) for a considerable period. The development of afterload mismatch eventually causes LV dysfunction. Therefore, previous studies have focused on the relation between LV ejection performance and LV wall stress. As pathologic findings in the course of adaptation and failure to increased wall stress, rearrangement and slippage of myocardial fibers, the addition of new sarcomeres, hypertrophy, and interstitial fibrosis have been observed in chronic AR. These myocardial structural changes affect myocardial function. However, how myocardial changes preserve global LV ejection performance has not yet been identified. Previous experimental studies have shown different changes between endocardial and epicardial deformation. Recent clinical studies have demonstrated changes in myocardial function in patients with AR through regional myocardial deformation imaging, including speckle-tracking echocardiography (STE).
Software developed for STE allows the assessment of intramural myocardial strain measurements with an intramural speckle-tracking system in addition to the widely used standard strain analysis consisting of circumferential strain (CS), radial strain (RS), and longitudinal strain (LS). The advantage of the system is the ability to separately measure endocardial, mid, and epicardial wall strain.
In the present study, we aimed to assess whether rearrangements of myocardial deformation occurred as an adaptation and failure of myocardial function against LV wall stress to preserve LVEF in patients with AR through the detailed analysis of transmural myocardial strain with this advanced speckle-tracking echocardiographic system.
Methods
Ninety patients (48 men, 42 women; mean age, 54 ± 17 years) with AR and 53 age-matched normal control subjects (37 men, 16 women; mean age, 51 ± 15 years) were enrolled in this study. The following exclusion criteria were applied: combined valvular disease, cardiomyopathy, coronary artery disease, and atrial fibrillation. The etiology of AR was a pure aortic valve deficiency in 70 patients, aortitis in 10, annuloaortic ectasia in six, and thoracic aortic aneurysms in four. The study was approved by the local research ethics committee, and all patients gave written informed consent.
Echocardiographic Examination
Echocardiographic examinations were performed using an Artida system (Toshiba Medical Systems Corporation, Tochigi, Japan). All imaging data for three cardiac cycles were digitized and stored on a hard disk on the ultrasound machine and then transmitted to a personal computer with a Pentium 4 processor (Intel Corporation, Santa Clara, CA) running at 1.7 GHz (Dell Computer, Tokyo, Japan) for further analysis.
On M-mode images at the mid-LV level, LV end-diastolic dimension, LV end-systolic dimension, end-diastolic interventricular septal thickness, and end-diastolic posterior wall thickness were measured. LVEF was calculated using the modified Simpson’s rule. LV mass was calculated as formula 1.04[(LV end-diastolic dimension + end-diastolic interventricular septal thickness + end-diastolic posterior wall thickness) 3 + (LV end-diastolic dimension) 3 ] − 13.6.
Severities of AR were identified by the ratio of the maximal proximal jet width to the LV outflow tract diameter, and severe AR was defined as a ratio > 65%. However, if the ratio was <65%, patients who had AR jets of until exceeding the cardiac apex and fulfilled at least one of the following criteria were classified as having severe AR: (1) pressure half-time of the AR jet < 200 msec and (2) the presence of abdominal aortic flow reversal throughout diastole.
Myocardial Strain Measurements
Speckle-tracking echocardiographic analysis for myocardial strain measurements was performed on LV short-axis images at the basal to mid ventricular level and on apical four-chamber images using speckle-tracking echocardiographic software (Toshiba Medical Systems Corporation). RS of the total, inner half, and outer half of the LV wall; CS of the inner, mid, and outer layers; and LS were automatically calculated ( Figure 1 ).

LV End-Systolic Wall Stress (ESS)
LV ESS in three directions was measured to assess the effect of wall stress on LVEF and myocardial strain; circumferential ESS (ESSc) and meridional ESS (ESSm) were calculated using Mirsky’s formula, and subendocardial radial ESS (ESSr) was calculated from the modified formula of Hittinger et al. The details of wall stress calculations are summarized in the Appendix .
Reproducibility of Strain Measurements
Two observers independently assessed myocardial strain and strain rate using STE. To test intraobserver variability, a single observer (N.I.) analyzed the data twice on occasions separated by an interval of 1 month. To test interobserver variability, a second observer (H.N.) analyzed the data without knowledge of the first observer’s measurements. Reproducibility was assessed as the mean percentage error (absolute difference divided by the mean of the two observations).
Statistical Analysis
Results are expressed as number (percentage) or as mean ± SD. One-way analysis of variance with post hoc Fisher’s test was used to compare variables among three or more groups. Chi-square tests were used for categorical variables. Comparisons between two groups were performed using Student’s t tests for continuous variables. Correlations between two variables were evaluated using Pearson’s rank correlation coefficient or exponential regression analysis. Multiple comparisons among regression lines were made using analysis of covariance. Because CS, LS, and ESSr were calculated as negative values, these parameters were multiplied by −1 and converted to positive values in some analyses. P values < .05 were considered to indicate statistical significance. All calculations were performed using SPSS version 18 for Windows (SPSS, Inc., Chicago, IL).
Results
Clinical Characteristics and Echocardiographic Measurements
Patients with AR were classified into the moderate AR group ( n = 31), the severe AR with preserved LVEF (ARpEF; ≥50%) group ( n = 42), and the severe AR with reduced LVEF (ARrEF; <50%) group ( n = 17). As summarized in Table 1 , LV mass was significantly larger in the severe ARpEF and ARrEF groups than in the control and moderate AR groups. LVEF even in the severe ARpEF group was lower than in the control and moderate AR groups.
Variable | Control ( n = 53) | Moderate AR ( n = 31) | Severe ARpEF ( n = 17) | Severe ARrEF ( n = 42) |
---|---|---|---|---|
Age (y) | 51 ± 15 | 56 ± 15 | 54 ± 15 | 51 ± 23 |
Men | 20 (37%) | 12 (38%) | 26 (61%) | 10 (58%) |
Heart rate (beats/min) | 64 ± 11 | 62 ± 14 | 66 ± 12 | 80 ± 23 ∗ |
SBP (mm Hg) | 114 ± 22 | 126 ± 20 | 129 ± 21 § | 130 ± 22 § |
DBP (mm Hg) | 68 ± 10 | 67 ± 13 | 55 ± 14 ‡ | 57 ± 13 ‡ |
LVDd (mm) | 46 ± 3.7 | 50 ± 4.5 § | 62 ± 6.8 ‡ | 64 ± 11 ‡ |
LVDs (mm) | 28 ± 3.3 | 31 ± 4.3 § | 41 ± 5.2 ‡ | 51 ± 8.0 ∗ |
LVEF (%) | 70 ± 5.3 | 68 ± 7.5 | 62 ± 5.0 ‡ | 42 ± 8.1 ∗ |
WTd (mm) | 8.1 ± 1.1 | 9.0 ± 1.4 § | 9.2 ± 1.6 § | 9.8 ± 1.7 ‡ |
WTs (mm) | 12.0 ± 1.7 | 13.3 ± 1.4 § | 13.5 ± 1.8 § | 13.0 ± 2.1 § |
LV mass (g) | 224 ± 52 | 294 ± 78 § | 465 ± 148 ‡ | 476 ± 211 ‡ |
AR jet width (%) | — | 37 ± 7.8 | 51 ± 12 † | 50 ± 13 † |
AR PHT (msec) | — | 505 ± 125 || | 330 ± 102 | 339 ± 130 |
AAFR | — | 0 | 29 (69%) | 11 (65%) |
‡ P < .01 versus control and moderate AR.
Myocardial Strain
The results of representative cases in the ARpEF group and in the control group are shown in Figure 1 . Strain data are summarized in Table 2 . LS was lower even in the moderate AR group as well as the severe ARpEF and ARrEF groups than in the control group. Inner CS was lower in the severe ARpEF and ARrEF groups than in the control and moderate AR groups. In contrast, mid CS and outer CS in the moderate AR group were significantly higher than in the control group. In addition, outer CS was higher even in the severe ARpEF group than in the control group. Total RS in the moderate AR group was significantly higher than in the control group. Total RS in the severe ARpEF group was lower than in the moderate AR group but did not differ from that in the control group. Inner RS progressively decreased with progression of AR severity, although the difference between the moderate AR and control groups was only slightly nonsignificant ( P = .052). In contrast, outer RS was higher even in the severe ARpEF group than in the control group. All strain parameters were lower in the severe ARrEF group than in the severe ARpEF group. The ratio of inner RS to outer RS was lower in all of the AR groups than in the control group. The ratio was higher in the severe ARrEF group than in the severe ARpEF group.
Variable | Control ( n = 53) | Moderate AR ( n = 31) | Severe ARpEF ( n = 17) | Severe ARrEF ( n = 42) |
---|---|---|---|---|
LS (%) | −16.3 ± 2.5 | −14.1 ± 2.7 || | −13.8 ± 3.1 || | −9.8 ± 3.2 ∗ |
Inner CS (%) | −27.8 ± 3.8 | −29.6 ± 5.1 | −25.4 ± 4.2 § | −16.7 ± 5.8 ∗ |
Mid CS (%) | −17.0 ± 2.8 ¶ | −19.5 ± 3.2 | −17.9 ± 3.0 ¶ | −11.9 ± 4.2 ∗ |
Outer CS (%) | −10.6 ± 2.6 ‡ | −11.7 ± 2.3 | −11.8 ± 2.3 | −8.3 ± 3.1 ∗ |
Total RS (%) | 43.6 ± 11.8 ¶ | 50.6 ± 18.8 | 42.7 ± 14.0 ¶ | 21.5 ± 10.6 ∗ |
Inner RS (%) | 52.9 ± 14.8 | 46.6 ± 17.3 | 38.3 ± 12.2 § | 21.4 ± 11.3 ∗ |
Outer RS (%) | 37.9 ± 11.8 ‡ | 60.1 ± 24.8 | 52.5 ± 19.4 | 22.9 ± 11.7 ∗ |
Inner RS/outer RS ratio | 1.43 ± 0.28 | 0.83 ± 0.25 || | 0.76 ± 0.19 † | 0.97 ± 0.35 || |
† P < .01 versus control and severe ARrEF.
‡ P < .01 versus moderate and severe ARpEF.
§ P < .01 versus control and moderate AR.
ESS and LVEF
Each ESS measurement was dramatically higher in the severe ARrEF group than even in the severe ARpEF group ( Table 3 ). ESSc and ESSm were higher in the severe ARpEF group than in the control and moderate AR groups, and ESSr was higher in the severe ARpEF than in the control group. In contrast, there were no significant differences in all stress parameters between the moderate AR and control groups. The analysis demonstrated significant inverse relations between ESS ( x ) and LVEF ( y ), as shown in Figure 2 . Correlations between LVEF and ESS were significant in the control group as well as the AR group. Comparison of the two-population regression by analysis of covariance showed a significant difference for slope in LVEF-ESSm ( P < .001) and LVEF-ESSc ( P = .002) relations. The y intercept in each relation was significantly higher than for the control group ( P < .001).
Variable | Control ( n = 53) | Moderate AR ( n = 31) | Severe ARpEF ( n = 17) | Severe ARrEF ( n = 42) |
---|---|---|---|---|
ESSc (g/cm 2 ) | 124 ± 24 | 127 ± 24 | 137 ± 28 ‡ | 171 ± 29 ∗ |
ESSm (g/cm 2 ) | 58 ± 13 | 61 ± 14 | 72 ± 18 ‡ | 101 ± 27 ∗ |
ESSr (g/cm 2 ) | −131 ± 21 | −140 ± 32 | −149 ± 28 † | −192 ± 32 ∗ |

ESS and Strain Relations
The relations between ESS and strain were assessed in the interacting directions: ESSm and LS, ESSc and CS, and ESSr and RS ( Figure 3 ). The regression analysis demonstrated heterogeneous ESS-strain correlations for each strain parameter. The correlation between LS and ESSm was significant but weak, because LS was decreased even in the moderate AR group at normal ESS ( Table 2 ). Second, inner CS demonstrated an inverse relation with ESSc, which was similar to the correlation of LVEF with ESSc ( Figure 2 ). In contrast, the correlations with ESSc were gradually weak from the mid to the outer layer.

In analysis of RS, total, inner, and outer RS-ESSr correlations in the control group were significant, as well as in the AR group. Comparison of the two-population regression by analysis of covariance showed no significant difference for the slope in each RS-ESSr relation. However, in inner RS and ESSr relations, the y intercept for the AR group was significantly lower than for the control group ( P < .001). In contrast, in outer RS and ESSr relations, the y intercept for the AR group was significantly higher than for the control group ( P < .001).
Strain and LVEF Relations
The relations between strain and LVEF are shown in Figure 4 . The correlations between strain and LVEF in the control group were significant except for outer CS and inner RS.

Comparison of the two-population regression by analysis of covariance showed no significant difference for both the slope and the y intercept in each strain-LVEF relation. The LVEF-LS relation was the weakest; LS was reduced in patients with preserved LVEFs compared with that in the control group. The relations between LVEF and inner CS and between LVEF and mid CS were strongly positive.
Total RS in 16 patients (18%) and outer RS in 35 patients (39%) were greater than the 95% confidence interval for the LVEF-strain relation in control subjects. Compared with a linear regression model, the relations between total RS and LVEF and between outer RS and LVEF were better fitted to exponential regression models (total RS = 6.7 × 0.03 × LVEF, R = 0.65, P < .001; outer RS = 6.6 × 0.03 × LVEF, R = 0.67, P < .001); notably, outer RS markedly increased in patients with preserved LVEFs, and the relations between LVEF and outer RS in patients with preserved LVEFs were not significant ( Table 2 ). As a net result, total RS values were higher in the moderate AR group and were preserved in the severe ARpEF group with slightly but significantly reduced LVEFs compared with those of the control group ( Tables 1 and 2 ).
Reproducibility of Strain Measurements
As summarized in Table 4 , intraobserver and interobserver variability of all strain measurements was <10%. The data suggest that the reproducibility of strain measurements was in the clinically acceptable range.
Variable | Intraobserver variability (%) | Interobserver variability (%) |
---|---|---|
Total RS | 4.5 ± 4.1 | 6.1 ± 4.9 |
Inner RS | 5.1 ± 4.1 | 4.0 ± 4.3 |
Outer RS | 5.3 ± 4.3 | 4.1 ± 3.4 |
Inner CS | 5.2 ± 4.2 | 5.8 ± 2.8 |
Mid CS | 6.0 ± 4.8 | 7.8 ± 4.9 |
Outer CS | 6.0 ± 4.7 | 6.2 ± 4.4 |
LS | 2.5 ± 1.8 | 5.4 ± 3.7 |
Results
Clinical Characteristics and Echocardiographic Measurements
Patients with AR were classified into the moderate AR group ( n = 31), the severe AR with preserved LVEF (ARpEF; ≥50%) group ( n = 42), and the severe AR with reduced LVEF (ARrEF; <50%) group ( n = 17). As summarized in Table 1 , LV mass was significantly larger in the severe ARpEF and ARrEF groups than in the control and moderate AR groups. LVEF even in the severe ARpEF group was lower than in the control and moderate AR groups.
Variable | Control ( n = 53) | Moderate AR ( n = 31) | Severe ARpEF ( n = 17) | Severe ARrEF ( n = 42) |
---|---|---|---|---|
Age (y) | 51 ± 15 | 56 ± 15 | 54 ± 15 | 51 ± 23 |
Men | 20 (37%) | 12 (38%) | 26 (61%) | 10 (58%) |
Heart rate (beats/min) | 64 ± 11 | 62 ± 14 | 66 ± 12 | 80 ± 23 ∗ |
SBP (mm Hg) | 114 ± 22 | 126 ± 20 | 129 ± 21 § | 130 ± 22 § |
DBP (mm Hg) | 68 ± 10 | 67 ± 13 | 55 ± 14 ‡ | 57 ± 13 ‡ |
LVDd (mm) | 46 ± 3.7 | 50 ± 4.5 § | 62 ± 6.8 ‡ | 64 ± 11 ‡ |
LVDs (mm) | 28 ± 3.3 | 31 ± 4.3 § | 41 ± 5.2 ‡ | 51 ± 8.0 ∗ |
LVEF (%) | 70 ± 5.3 | 68 ± 7.5 | 62 ± 5.0 ‡ | 42 ± 8.1 ∗ |
WTd (mm) | 8.1 ± 1.1 | 9.0 ± 1.4 § | 9.2 ± 1.6 § | 9.8 ± 1.7 ‡ |
WTs (mm) | 12.0 ± 1.7 | 13.3 ± 1.4 § | 13.5 ± 1.8 § | 13.0 ± 2.1 § |
LV mass (g) | 224 ± 52 | 294 ± 78 § | 465 ± 148 ‡ | 476 ± 211 ‡ |
AR jet width (%) | — | 37 ± 7.8 | 51 ± 12 † | 50 ± 13 † |
AR PHT (msec) | — | 505 ± 125 || | 330 ± 102 | 339 ± 130 |
AAFR | — | 0 | 29 (69%) | 11 (65%) |
‡ P < .01 versus control and moderate AR.
Myocardial Strain
The results of representative cases in the ARpEF group and in the control group are shown in Figure 1 . Strain data are summarized in Table 2 . LS was lower even in the moderate AR group as well as the severe ARpEF and ARrEF groups than in the control group. Inner CS was lower in the severe ARpEF and ARrEF groups than in the control and moderate AR groups. In contrast, mid CS and outer CS in the moderate AR group were significantly higher than in the control group. In addition, outer CS was higher even in the severe ARpEF group than in the control group. Total RS in the moderate AR group was significantly higher than in the control group. Total RS in the severe ARpEF group was lower than in the moderate AR group but did not differ from that in the control group. Inner RS progressively decreased with progression of AR severity, although the difference between the moderate AR and control groups was only slightly nonsignificant ( P = .052). In contrast, outer RS was higher even in the severe ARpEF group than in the control group. All strain parameters were lower in the severe ARrEF group than in the severe ARpEF group. The ratio of inner RS to outer RS was lower in all of the AR groups than in the control group. The ratio was higher in the severe ARrEF group than in the severe ARpEF group.
Variable | Control ( n = 53) | Moderate AR ( n = 31) | Severe ARpEF ( n = 17) | Severe ARrEF ( n = 42) |
---|---|---|---|---|
LS (%) | −16.3 ± 2.5 | −14.1 ± 2.7 || | −13.8 ± 3.1 || | −9.8 ± 3.2 ∗ |
Inner CS (%) | −27.8 ± 3.8 | −29.6 ± 5.1 | −25.4 ± 4.2 § | −16.7 ± 5.8 ∗ |
Mid CS (%) | −17.0 ± 2.8 ¶ | −19.5 ± 3.2 | −17.9 ± 3.0 ¶ | −11.9 ± 4.2 ∗ |
Outer CS (%) | −10.6 ± 2.6 ‡ | −11.7 ± 2.3 | −11.8 ± 2.3 | −8.3 ± 3.1 ∗ |
Total RS (%) | 43.6 ± 11.8 ¶ | 50.6 ± 18.8 | 42.7 ± 14.0 ¶ | 21.5 ± 10.6 ∗ |
Inner RS (%) | 52.9 ± 14.8 | 46.6 ± 17.3 | 38.3 ± 12.2 § | 21.4 ± 11.3 ∗ |
Outer RS (%) | 37.9 ± 11.8 ‡ | 60.1 ± 24.8 | 52.5 ± 19.4 | 22.9 ± 11.7 ∗ |
Inner RS/outer RS ratio | 1.43 ± 0.28 | 0.83 ± 0.25 || | 0.76 ± 0.19 † | 0.97 ± 0.35 || |
† P < .01 versus control and severe ARrEF.
‡ P < .01 versus moderate and severe ARpEF.
§ P < .01 versus control and moderate AR.
ESS and LVEF
Each ESS measurement was dramatically higher in the severe ARrEF group than even in the severe ARpEF group ( Table 3 ). ESSc and ESSm were higher in the severe ARpEF group than in the control and moderate AR groups, and ESSr was higher in the severe ARpEF than in the control group. In contrast, there were no significant differences in all stress parameters between the moderate AR and control groups. The analysis demonstrated significant inverse relations between ESS ( x ) and LVEF ( y ), as shown in Figure 2 . Correlations between LVEF and ESS were significant in the control group as well as the AR group. Comparison of the two-population regression by analysis of covariance showed a significant difference for slope in LVEF-ESSm ( P < .001) and LVEF-ESSc ( P = .002) relations. The y intercept in each relation was significantly higher than for the control group ( P < .001).
Variable | Control ( n = 53) | Moderate AR ( n = 31) | Severe ARpEF ( n = 17) | Severe ARrEF ( n = 42) |
---|---|---|---|---|
ESSc (g/cm 2 ) | 124 ± 24 | 127 ± 24 | 137 ± 28 ‡ | 171 ± 29 ∗ |
ESSm (g/cm 2 ) | 58 ± 13 | 61 ± 14 | 72 ± 18 ‡ | 101 ± 27 ∗ |
ESSr (g/cm 2 ) | −131 ± 21 | −140 ± 32 | −149 ± 28 † | −192 ± 32 ∗ |
ESS and Strain Relations
The relations between ESS and strain were assessed in the interacting directions: ESSm and LS, ESSc and CS, and ESSr and RS ( Figure 3 ). The regression analysis demonstrated heterogeneous ESS-strain correlations for each strain parameter. The correlation between LS and ESSm was significant but weak, because LS was decreased even in the moderate AR group at normal ESS ( Table 2 ). Second, inner CS demonstrated an inverse relation with ESSc, which was similar to the correlation of LVEF with ESSc ( Figure 2 ). In contrast, the correlations with ESSc were gradually weak from the mid to the outer layer.
In analysis of RS, total, inner, and outer RS-ESSr correlations in the control group were significant, as well as in the AR group. Comparison of the two-population regression by analysis of covariance showed no significant difference for the slope in each RS-ESSr relation. However, in inner RS and ESSr relations, the y intercept for the AR group was significantly lower than for the control group ( P < .001). In contrast, in outer RS and ESSr relations, the y intercept for the AR group was significantly higher than for the control group ( P < .001).
Strain and LVEF Relations
The relations between strain and LVEF are shown in Figure 4 . The correlations between strain and LVEF in the control group were significant except for outer CS and inner RS.
Comparison of the two-population regression by analysis of covariance showed no significant difference for both the slope and the y intercept in each strain-LVEF relation. The LVEF-LS relation was the weakest; LS was reduced in patients with preserved LVEFs compared with that in the control group. The relations between LVEF and inner CS and between LVEF and mid CS were strongly positive.
Total RS in 16 patients (18%) and outer RS in 35 patients (39%) were greater than the 95% confidence interval for the LVEF-strain relation in control subjects. Compared with a linear regression model, the relations between total RS and LVEF and between outer RS and LVEF were better fitted to exponential regression models (total RS = 6.7 × 0.03 × LVEF, R = 0.65, P < .001; outer RS = 6.6 × 0.03 × LVEF, R = 0.67, P < .001); notably, outer RS markedly increased in patients with preserved LVEFs, and the relations between LVEF and outer RS in patients with preserved LVEFs were not significant ( Table 2 ). As a net result, total RS values were higher in the moderate AR group and were preserved in the severe ARpEF group with slightly but significantly reduced LVEFs compared with those of the control group ( Tables 1 and 2 ).
Reproducibility of Strain Measurements
As summarized in Table 4 , intraobserver and interobserver variability of all strain measurements was <10%. The data suggest that the reproducibility of strain measurements was in the clinically acceptable range.
Variable | Intraobserver variability (%) | Interobserver variability (%) |
---|---|---|
Total RS | 4.5 ± 4.1 | 6.1 ± 4.9 |
Inner RS | 5.1 ± 4.1 | 4.0 ± 4.3 |
Outer RS | 5.3 ± 4.3 | 4.1 ± 3.4 |
Inner CS | 5.2 ± 4.2 | 5.8 ± 2.8 |
Mid CS | 6.0 ± 4.8 | 7.8 ± 4.9 |
Outer CS | 6.0 ± 4.7 | 6.2 ± 4.4 |
LS | 2.5 ± 1.8 | 5.4 ± 3.7 |
Discussion
Despite subendocardial dysfunction with increased ESS, subepicardial deformation increased in patients with preserved LVEFs, suggesting that increased subepicardial deformation compensates for reduced subendocardial deformation and preserves total wall thickening. Thus, rearrangement of myocardial deformation may be an underlying mechanism to preserve LVEF.
Early Longitudinal Contraction Abnormality in AR
The present study showed significant decreases of LS even in the compensated stage. In recent studies, longitudinal dysfunction has been reported in patients referred for aortic valve replacement. In contrast, long-axis dysfunction in patients with AR has been observed in the compensated stage. Olsen et al. recently confirmed that LS measured by STE was associated with disease progression even in early compensated AR. In addition, Mizariene et al. reported that subclinical longitudinal dysfunction was manifested in patients with moderate AR and hypertension compared with those without hypertension. Because subendocardial myocardium fiber direction depends on longitudinal direction, long-axis contraction abnormalities may be related to early myocardial dysfunction caused by subendocardial afterload mismatch, subendocardial ischemia, and fibrosis in patients with AR.
Transmural Compensation
In addition to long-axis contraction abnormalities, inner-half wall thickening was impaired, which was related to subendocardial higher wall stress. In contrast, mid CS was normal, and outer-half wall thickening was increased. These findings suggest that wall thickening can be generated by outer-half fibers if subendocardial deformation is impaired. The increased outer-half wall thickening may be explained as follows. First, normalized mid CS in the severe ARpEF group suggests preserved myocardial fiber shortening in the mid LV layer. Accordingly, disturbance of the mid to subepicardial myocardial fibers was thought to be less than that of the subendocardial myocardial fibers. In addition, the cross-fiber shortening theory supports compensated wall thickening by outer-half fibers. Fiber strain is not significantly different at the epicardial, midwall, or endocardial layers. However, the deformation perpendicular to fiber direction increases from the epicardium toward the endocardium. The transmural heterogeneous deformations correspond to larger circumferential and radial deformation in the endocardium compared with those in the epicardium. Previous studies demonstrated the underlying mechanism using the cross-fiber shortening theory.
Namely, myocardial fiber shortening in one layer generates extensive cross-fiber shortening in other layer that is located in the perpendicular or cross-fiber direction. Because the fibers in the intact LV wall change from an oblique orientation at the epicardium to a circumferential orientation at the midwall to a reverse oblique direction at the endocardium, epicardial and endocardial fiber directions are closer to perpendicular. Therefore, epicardial fiber shortening generates extensive endocardial cross-fiber shortening through rearrangement of fibers. Thus, wall thickening is mainly passive deformation through the interaction of layers and rearrangement of fibers. Rearrangements of myocardial fiber orientation in outer-half myocardium may contribute to increased wall thickening. Such “transmural compensation” by less impaired epicardial fibers has been suggested in previous studies. Chan et al. reported preserved transverse wall thickening despite long-axis myocardial dysfunction with subendocardial infarction. The underlying mechanism is similar to our findings in AR. Accordingly, the normal LVEF despite increased ESS in patients with severe AR may be maintained by the transmural rearrangement of wall thickening.
Myocardial Decompensation for Marked Increased Wall Stress
Another supporting argument of transmural compensation is an exponential regression between RS, in particular outer RS, and LVEF ( Figure 4 ), suggesting loss of subepicardial compensation under markedly increased wall stress. Because it was clearly observed in patients with LVEFs < 50%, patients with AR may be vulnerable at an LVEF of about 50%. The findings support a cutoff point for LVEF of 50% in patients with asymptomatic AR as being adequate to determine timing of aortic valve replacement.
Study Limitations
First, severities of AR were not assessed by quantitative methods, including proximal isovelocity surface area or flow volume measurement techniques. The technical limitations might affect our classifications of severities of AR, although it was assessed by comprehensive use of AR-specific signs.
Similar to other automated image-tracking modalities, the quality of our tracking algorithm was dependent on the two-dimensional echocardiographic image. Although we carefully obtained images that clearly visualized the myocardium during the whole cardiac cycle, image quality may affect speckle-tracking precision. These imaging limitations may affect the strain data.
The present study does not clarify the clinical implications of strain analysis in patients with AR. In the future, research is needed to assess the relation of our data with prognosis in patients with AR.
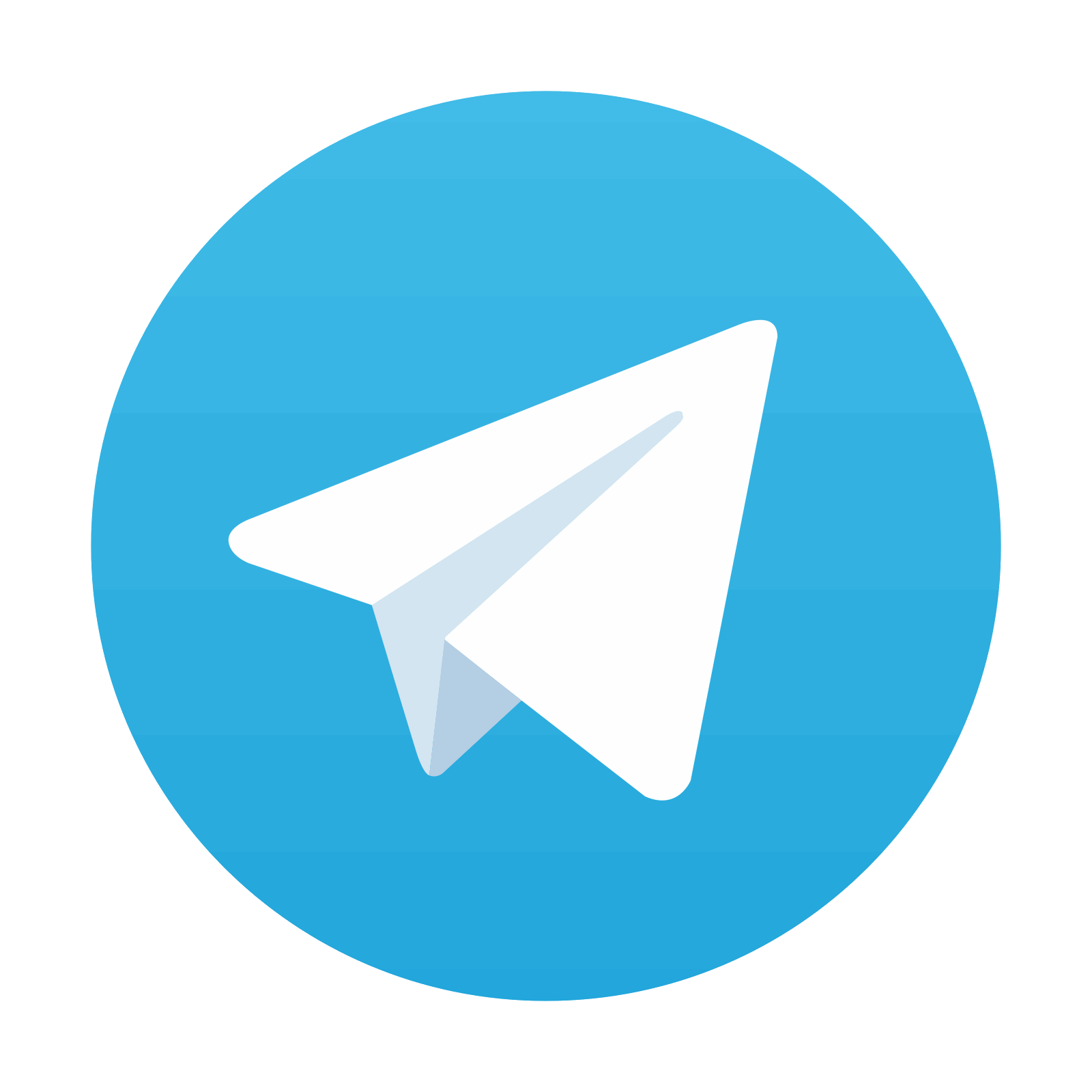
Stay updated, free articles. Join our Telegram channel

Full access? Get Clinical Tree
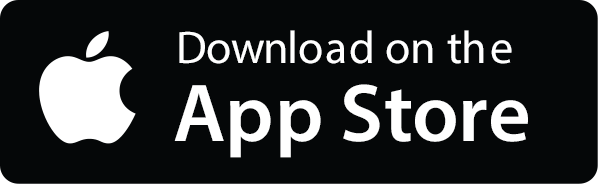
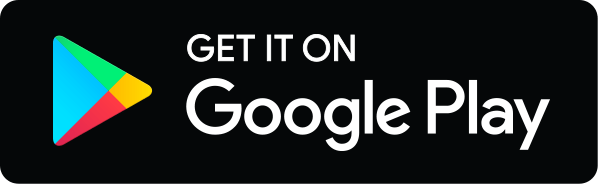