
Section I: Mitral Stenosis
Introduction
Normal Mitral Valve Anatomy
To appreciate the complexity of the mitral valve is to understand its intricate anatomical and physiologic properties. The mitral valve can be divided into several components, each one important in maintaining proper valve functionality. The mitral valve consists of two leaflets, a posterior leaflet with three distinct scallops and a corresponding, but much larger, anterior leaflet. The two mitral valve leaflets are continuous with each other and maintain a connection at the commissures. The anterior leaflet is continuous with the ascending aorta and the membranous ventricular septum. The leaflets anchor to the myocardium by way of two papillary muscles, the anterolateral and posteromedial. The anterolateral papillary muscle is usually the larger of the two structures. The attachment between the papillary muscle and the valve leaflets occurs by way of chordae tendinae.
With systolic contraction of the left ventricular (LV) cavity, the resultant traction of the papillary muscles leads to valve closure by transmission of the force through the chordae. The chordae tendinae insert on the underside of the leaflet tissue. Blood in the ventricular chamber passes through interchordal spaces on its route from the left atrium.
The two mitral valve leaflets attach to the base of the left atrium to a thin ovoid-shaped membrane—the mitral valve annulus. The annulus itself is a nonplanar saddle-shaped structure, which functions to influence valve competency. In close proximity to the anterior portion of the mitral valve annulus are two fibrous trigones, which are separated by the fibrous intertrigonal region. The posterior portion of the annulus is not surrounded by any fibrous tissue, however, and serves to separate the ventricular tissue from the atria.
The coronary sinus generally lies close to the posterior side of the mitral valve annulus. This anatomic relationship allows for the use of percutaneous annuloplasty techniques. There is variability in the relationship between the annulus and the coronary sinus and is likely influenced by the size of the left atrium and other factors. For the purpose of percutaneous interventions on the mitral valve apparatus, it is important to consider the relationship between the coronary sinus and the course of the left circumflex coronary artery. Cardiac computed tomography has been used to demonstrate that the left circumflex artery crosses between the mitral annulus and the coronary sinus in the vast majority of patients with significant variability in the location of crossover.
Disease of the mitral valve can be primarily valvular, or secondary to other processes, notably those affecting the myocardium. The standard of care for treatment of mitral valve disease has been primarily surgical. Several percutaneous approaches to treating mitral valve disease have emerged and continue to evolve as possible alternatives to surgical treatment, particularly in those patients not considered appropriate surgical candidates. As such, an appreciation for the complexity of mitral valve anatomy is necessary to understand how, and why, percutaneous approaches work in treatment of mitral valve disease.
Mitral Valve Disease States: Mitral Stenosis
Etiologies of Mitral Stenosis
The primary disturbance in mitral stenosis is an obstruction to the inflow of blood from the left atrium into the left ventricle during diastole. Worldwide, the primary etiology of mitral stenosis is rheumatic heart disease. Rheumatic changes can affect the mitral valve itself and the subvalvular apparatus as well. Less common etiologies for mitral stenosis include congenital abnormalities such as cor triatriatum and parachute mitral valve, and noncongenital etiologies such as left atrial (LA) myxoma, endocarditis, carcinoid, mucopolysaccharidosis, rheumatoid arthritis, and systemic lupus erythematosus, among others.
Lutembacher syndrome denotes the presence of a secondary atrial septal defect and rheumatic mitral stenosis. In the elderly population, severe mitral annular calcifications can lead to varying degrees of mitral stenosis and mitral regurgitation. For the remainder of the discussion on mitral stenosis and its treatment by percutaneous balloon mitral valvuloplasty, we will focus only rheumatic mitral stenosis.
Pathology of Rheumatic Mitral Stenosis
As mentioned, the most common etiology for mitral stenosis, worldwide, is rheumatic heart disease. In patients with rheumatic mitral stenosis, as many as half of patients are not aware of a prior history of rheumatic fever. Following an episode of acute rheumatic fever, it may take several years prior to the development of mitral stenosis and many more years until the development of symptoms. Progression of disease and development of clinical symptoms correlate with episodes of rheumatic fever. Ongoing inflammatory insults affecting the valve may result in eventual clinical manifestations.
The basis for the development of mitral stenosis following streptococcal infection is believed to be molecular mimicry. The similarity between streptococcal bacterial M protein and human cardiac proteins (myosin and others) is likely the reason for molecular mimicry between the two organisms. Humoral and cellular responses are important in the pathogenesis, and the CD4+ T cell response is the main culprit of the molecular mimicry response affecting cardiac tissue.
In rheumatic mitral stenosis, there is diffuse fibrous thickening of the mitral valve leaflets with calcific deposits. In addition to fibrous thickening, there is fusion of the leaflet commissures along with shortening and fusion of the subvalvular chordae tendinae. The amount of calcification of the valve leaflets itself is variable. This process is not, however, limited to the valves as it affects the three layers of the heart as well with increased amounts of fibrous tissue.
Pathophysiology of Rheumatic Mitral Stenosis
In addition to the characteristic findings of mitral stenosis upon the valve, these structures can also be affected by concomitant calcification. In combination, these changes result in significant restriction in the opening of the mitral leaflet. As such, there is severe reduction in size of the mitral valve orifice leading to a funnel-shaped orifice often described as “fish mouth” in appearance. Also, there can be associated mitral regurgitation due to leaflet malcoaptation arising from fibrosis and shortening of the subvalvular apparatus, including the chordae.
The end result of these changes upon the mitral valve complex is a restriction of blood flow across the mitral valve orifice. The normal mitral valve has an orifice area of between 4 cm 2 to 6 cm 2 . Mitral stenosis is a slowly progressive disease that worsens over the course of many years. With a reduction in mitral valve orifice area, a higher transvalvular pressure gradient may develop, leading to increased LA pressure. There is then transmission of elevated LA pressure into the pulmonary vasculature, eventually leading to the development of pulmonary venous pressures. Long standing mitral stenosis and increased LA pressures can lead to severe and irreversible pulmonary hypertension both from elevated LA pressures, pulmonary vascular constriction, and obliterative changes to the vasculature.
With chronically elevated pulmonary arterial pressures in long-standing and untreated mitral stenosis, patients can develop right ventricular dilation and dysfunction over time. With development of severe tricuspid valve regurgitation as a consequence, signs and symptoms of right heart failure can develop. Most patients will start to develop symptoms when the mitral valve area (MVA) is between 1.0 cm 2 and 1.5 cm 2 in area. It is important to understand that the process can affect other valves and the myocardium, leading to ventricular dysfunction, which can contribute to symptoms.
Clinical Presentation of Mitral Stenosis
Clinical Manifestations
Patients will often present with symptoms related to mitral stenosis several years to decades after their initial bout of rheumatic fever. In developing countries this may occur sooner due to repeat bouts of rheumatic fever. With development of symptoms, long-term survival is severely compromised.
According to the World Health Organization, reliable statistics on the incidence and prevalence of rheumatic fever are not easily available. However, the annual incidence in developing countries has been reported to vary between 1 per 100,000 school-age children to over 150 per 100,000 school-age children. The prevalence of rheumatic heart disease varies from between 0.5 per 1000 school-age children in developed countries to over 70 per 1000 school-age children in other developing countries. It is reported that rates vary even within developing countries, and hospital admissions for rheumatic heart disease vary between 12% and 65%.
The diagnosis of rheumatic fever is made based on the Jones Criteria, first published by Dr. T. Duckett Jones in 1944, and since revised. The presence of two major, or one major and two minor, criteria must be met. The major criteria include carditis, polyarthritis, erythema marginatum, chorea, and subcutaneous nodules. The minor criteria include fever, arthralgias, elevated sedimentation rate, elevated C reactive protein, and a prolonged PR interval. These findings are used in the context of a documented Group A β-hemolytic streptococcal throat infection.
Although the progression in the rate of mitral valve stenosis is variable, it is estimated to be about a 0.1 cm 2 reduction in valve area per year. The onset of clinical manifestations is also variable. The primary symptom in mitral valve stenosis is exertional dyspnea secondary to elevated LA pressures and elevated pulmonary venous and arterial pressures. This is often accompanied by orthopnea, coughing, and wheezing. Even when mitral stenosis is less advanced, co-existent conditions such as anemia, pregnancy, infection, atrial fibrillation, exertion, or fever can precipitate symptoms by decreasing diastolic filling time and increasing blood flow across the stenotic mitral orifice.
With progression of disease, patients can experience pulmonary edema, atrial fibrillation, pulmonary hemorrhage from rupture of dilated bronchial veins, and chest pain. Other less common symptoms include systemic embolization, clinical signs and symptoms of right heart failure, and hoarseness due to compression of the left recurrent laryngeal nerve by dilated pulmonary artery, enlarged lymph nodes, or an enlarged left atrium (Ortner’s syndrome).
Clinical Assessment
History and Physical Examination
Oftentimes, obtaining a history in a patient with rheumatic mitral stenosis will not reliably reveal a prior history of rheumatic fever. Furthermore, given the slow progression of the disease, many patients will report being asymptomatic and deny frank exertional dyspnea. It is important to note that given the slow progression, patients will reduce their level of activity to remain without symptoms.
The patient with severe mitral stenosis will demonstrate purple and pink patches on the cheeks termed “mitral facies.” It is important to remember that in severe mitral stenosis, LA function may be normal and LA filling pressures may be low or normal. As such, palpation of the left ventricle will likely reveal a normal apical impulse. With palpation, a left parasternal right ventricular heave can sometimes be appreciated in the setting of pulmonary hypertension. Jugular venous pressure can be elevated in the setting of right ventricular dysfunction.
Auscultation of the patient with severe mitral stenosis will reveal several characteristics about the mitral valve itself. The characteristic findings on auscultation are that of a loud S1, an opening snap (OS), and a mid-diastolic murmur, described as a rumble. The opening snap follows A2 and can be best heard at the left lower sternal border. The pliability of anterior mitral leaflet is the source of the sound for the OS. The opening snap usually follows the A2 sound by 0.04 seconds to 0.12 seconds. During exhalation, it is possible to differentiate the physiologic splitting of the second heart sound from the OS, as the two sounds are that of the S2 followed by the OS. The duration of time between the A2 and the OS is also a marker of severity of disease. Specifically, increased pressure gradient or elevated LA pressure leads to faster opening of the mitral valve in diastole. As such, the time duration between A2 and OS will be markedly shorter in the setting of higher LA pressure and severity of mitral stenosis. Maneuvers that decrease venous blood return will serve to increase the A2 to OS interval.
The diastolic murmur of mitral stenosis is best appreciated with the patient in the left lateral decubitus position and is often described as a low pitch rumble at the apex. The duration of the murmur is a marker for severity of disease as it is driven by the gradient across the left atrium and ventricle. Maneuvers such as exhalation can accentuate the MS murmur and Valsalva can serve to decrease the intensity of the diastolic murmur due to a reduction in flow across the mitral valve.
Clinical Assessment with Diagnostic Tools
The diagnostic workup of the patient with mitral stenosis relies heavily on the echocardiographic findings. The electrocardiogram (ECG) and chest x-ray are at times helpful, but the diagnosis squarely rests on the results of noninvasive imaging. The ECG will often reveal findings consistent with LA enlargement and the chest x-ray can demonstrate LA enlargement as well as enlargement of the pulmonary vasculature and pulmonary edema, on occasion.
Echocardiography
Transthoracic (TTE) or transesophageal (TEE) echocardiography can be used to diagnose and to determine severity of mitral stenosis ( Figure 30-1AB ). TTE can be helpful in the assessment of the morphology, mobility, and function of the mitral valve itself. The characteristic thickening and doming, or “hockey stick” appearance, of the anterior mitral leaflet can be appreciated in the parasternal long axis on TTE. There is a reduction in leaflet mobility noted and TTE can also be used to quantify the degree of mitral regurgitation, if any. In addition, TTE can be useful in the assessment of the subvalvular apparatus, the degree of calcification of the valve leaflets, commissures and the subvalvular apparatus, pulmonary arterial pressures, and concomitant valvular disease.

Determining the morphology of the mitral valve and the subvalvular apparatus is important in preprocedural planning for balloon mitral valvuloplasty. The suitability of a valve for balloon mitral valvuloplasty can be determined using the Wilkins criteria, which scores four features of the valve including leaflet mobility, leaflet thickening, leaflet calcification, subvalvular calcification, and thickening. Each feature is assigned a score from 1 to 4 and higher valve scores (maximum of 16) predict unfavorable outcomes with mitral valvuloplasty.
Assessment of mitral valve gradients is best performed with TTE in the apical-4 chamber view with the Doppler beam parallel to the mitral jet so as to obtain the most accurate gradients. Assessment of MVA with TTE can be performed with planimetry, continuity equation, or with pressure half-time (PHT) measurements. Planimetry of the mitral valve orifice is performed using the parasternal short axis view and it is important to start imaging at the apex and move upwards so as to identify the smallest mitral valve orifice for measurement. This method can at times lead to overestimation of the MVA if the imaging plane is proximal to the true orifice or due to irregularities in the orifice itself.
The PHT is a flow-dependent method that estimates MVA based on the time needed for the peak gradient across the valve to fall by half ( Figure 30-2 ). The slope derived from this decrease is related to the degree of stenosis. With more severe stenosis, there is a larger gradient and a smaller mitral orifice, which will result in a longer time for pressure decline and a longer PHT. With PHT, one can calculate MVA as follows:
M V A = 220 / P H T

In situations where there is an alteration in the LA or LV pressures, the PHT measurement can be unreliable. In the setting of LV dysfunction with increased LVEDP, the PHT will be shortened, thus overestimating the MVA. In patients with severe aortic regurgitation, the rise in LV end-diastolic pressure (EDP) can also lead to shortening of the PHT and an overestimation of the MVA.
TEE can be used to further assess the morphology of the mitral valve and the subvalvular apparatus. Prior to catheter-based intervention, TEE can be used to assess for LA, or LA appendage thrombus, in patients with atrial fibrillation. TEE can also be used to help guide catheter-based interventions including safely crossing the atrial septum into the left atrium and to assess mitral valve morphology post-balloon valvuloplasty.
Exercise stress echocardiography can also be utilized in the assessment of mitral valve stenosis severity. It can be used to evaluate exercise tolerance in mitral stenosis patients who are otherwise asymptomatic or to assess severity of otherwise mild mitral stenosis at rest in patients with clear symptoms. Exercise testing can also be performed with a pulmonary artery catheter. Significant increases in PA systolic pressures or mean mitral valve gradients can help identify patients who would benefit from mitral valuloplasty.
Cardiac Catheterization and Angiography
While echocardiography remains the mainstay of diagnosis, cardiac catheterization is not only a confirmatory diagnostic modality, as it serves to resolve discrepancies between clinical and noninvasive imaging findings, assess for coronary atherosclerosis as an etiology for symptoms, or prior to mitral valve surgery. It also serves to help determine the role of mitral stenosis in patients with concomitant lung disease.
Assessment of mitral valve gradients can be accomplished using an LV pigtail catheter and a PA catheter with pulmonary capillary wedge pressure (PCWP) measurements as a surrogate for LA pressure. The PCWP tends to overestimate the gradients across the mitral valve. The use of the PCWP may be unreliable in patients with pulmonary veno-occlusive disease. Direct measurement of the LA pressure can also be accomplished by crossing the atrial septum under fluoroscopic or echocardiographic guidance. Accurate gradients can be calculated using direct LA and LV measurements ( Figure 30-3AB ).


MVA can be calculated using the Gorlin formula. Cardiac output can be calculated using right heart catheterization with the thermodilution, or Fick measurements. In patients with low output or concomitant regurgitant valvular lesions, Fick measurements of cardiac output may be more accurate. The Gorlin formula is as follows:
M V A ( c m 2 ) = [ S V / D F P ] / [ K * ( s q r t m e a n M V g r a d i e n t ) ]
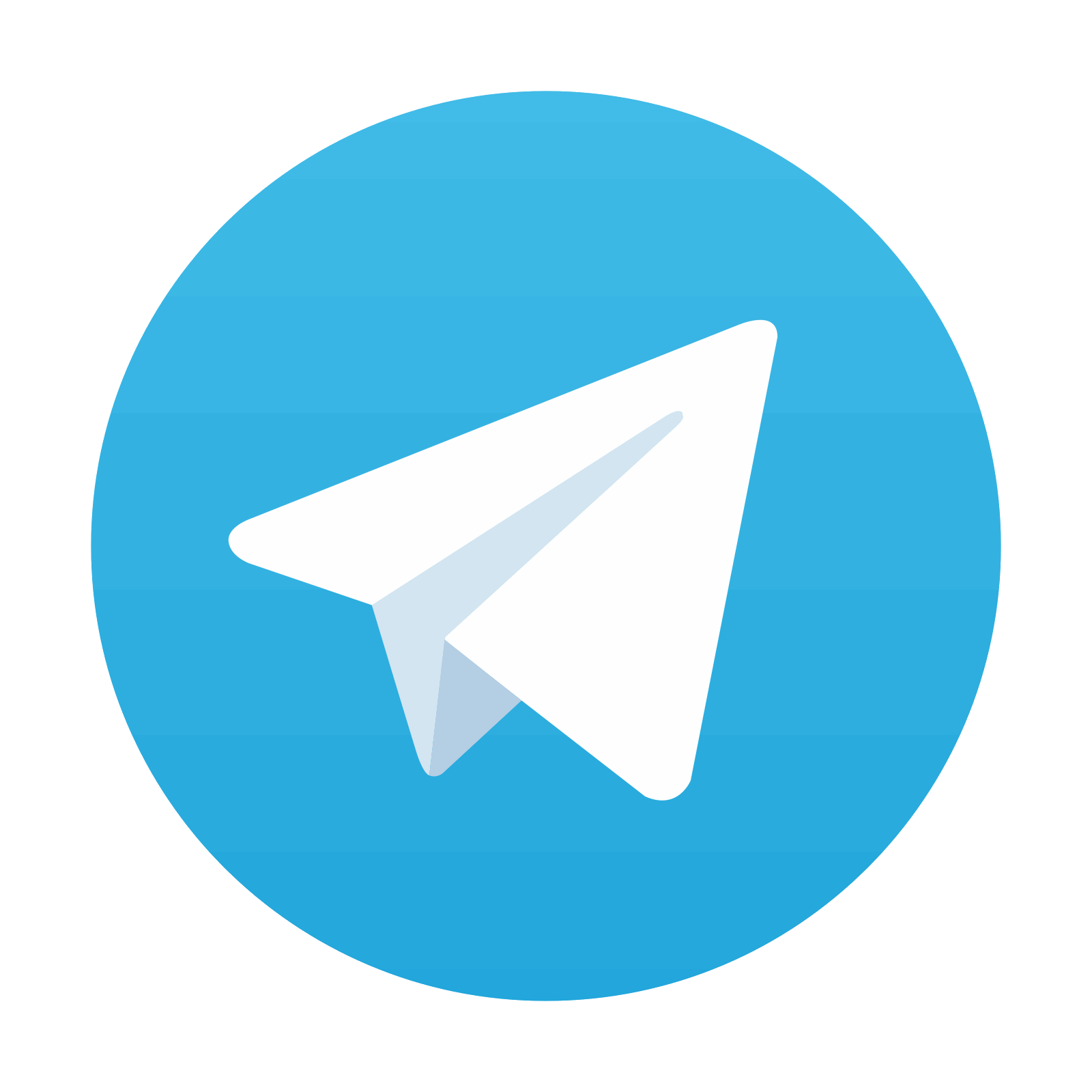
Stay updated, free articles. Join our Telegram channel

Full access? Get Clinical Tree
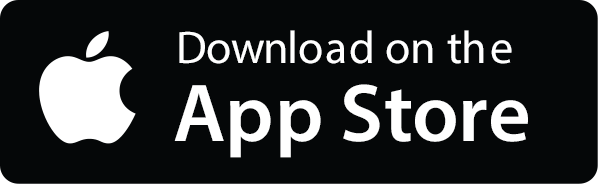
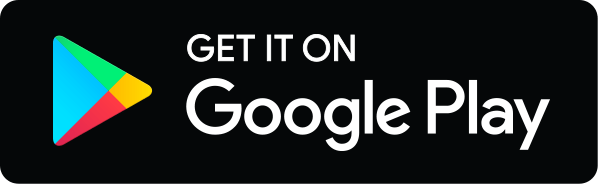