© Springer International Publishing Switzerland 2016
Rosalinda Madonna (ed.)Stem Cells and Cardiac RegenerationStem Cell Biology and Regenerative Medicine10.1007/978-3-319-25427-2_44. Tissue Specific Progenitors/Stem Cells for Cardiac Regeneration
(1)
Institute of Cardiology, Department of Neurosciences and Imaging Laboratory of Experimental Cardiology, Center of Excellence on Aging—CESI, “G. d’Annunzio” University, Chieti Scalo, Italy
Keywords
Tissue specific progenitors cellsBone marrow cellsAdipose tissue-derived stem cellsCardiac stem cells4.1 Bone Marrow-Derived Stem Cells
Bone marrow-derived stem cells are currently the most commonly used cells in cell transplantation therapy. Strauer et al. (2002) treated 10 patients with acute ST elevation myocardial infarcts using autologous mononuclear bone marrow cells through a balloon catheter placed into an infarct-related coronary artery (IRA) following percutaneous transluminal coronary angioplasty (PTCA) . Three months later, the infarct region in the BMC-treated group was decreased compared with that at baseline (from 30 ± 13 to 12 ± 7 %, p = 0.005); segmental wall movement velocity increased significantly only in the BMC-treated group (from 2.0 ± 1.1 to 4.0 ± 2.6 cm/s, p = 0.028). Ejection fraction increased in both groups, albeit not significantly. Dobutamine stress echocardiography, radionuclide ventriculography, and right heart catheterization of the BMC-treated group showed improved stroke volume index, left ventricular end diastolic volume, and contractility. These results demonstrated that intracoronary injection of autologous mononuclear BMCs is safe and apparently effective under clinical conditions. The TOPCARE-AMI trial led by Zeiher et al. included 20 patients with acute myocardial infarction (AMI) who received intracoronary infusion of bone marrow derived progenitor cells (n = 9) and blood-derived progenitor cells (n = 11) (Assmus et al. 2002). This treatment resulted in significantly increased global LVEF, improved regional wall motion in the infarct zone, and profoundly reduced end-systolic left ventricular volumes at 4 months follow-up. Compared with a non-randomized matched control group, LVEF only slightly increased and end-systolic volume remained unchanged. These preliminary data demonstrated the feasibility, safety, and potentially beneficial effects of intracoronary autologous progenitor cells in AMI patients. Another study from the same trial included 28 patients who received circulating blood- or bone marrow-derived progenitor cells (Britten et al. 2003). The results demonstrated that progenitor cell transplantation played an important role in improving LVEF and ameliorating ventricular remodeling after infarction. Although these two studies did not reveal the cellular mechanisms associated with the improvement of LVEF after cell therapy, they did provide preliminary evidence that autologous progenitor cells can migrate into damaged myocardium and exert beneficial effects on heart function. Fuchs et al. (2003) injected bone marrow cells in 10 patients with end-stage heart failure by a strategy of endocardial delivery that utilized left ventricular electromechanical guidance. The mean time for bone marrow aspiration and processing was 2.5 h, and among the CD45/CD34 double positive cells injected, 85 ± 14 % co-expressed stem cell factor receptor CD117. Three months later, there were no reports of arrhythmia, infection, myocardial inflammation, or fibrosis. Although there was no change in EF, 8 of the 10 patients had less severe angina symptoms, and 9 patients showed prolonged exercise duration times on a treadmill test. Despite not showing efficacy, this study did demonstrate the possibility of transendocardial administration of freshly aspirated autologous bone marrow cells. Perin et al. (2003) have demonstrated the safety and efficacy of transendocardial injection of autologous bone marrow mononuclear cells in patients with end-stage ischemic heart disease. Fourteen patients were successfully injected with such cells. At 2 months follow-up, their symptoms of heart failure and angina had improved, when compared with those in the control group. METs and VO2-max on a treadmill test also improved in the cell-treated group. LVEF by echocardiography increased in both the treated and control groups. However, 4 months later, after repeat left ventriculography and electromechanical mapping were performed in the cell-treated group, the results showed increased LVEF (from 20 to 29 %, p = 0.0003) and improved contraction at the injection site. Also, in an experimental ischemic canine model, bone marrow-derived mesenchymal stem cells (BMM-SCs) have been shown to differentiate into endothelial cells and enhance neovascularization after myocardial injection via the NOGA system (Perin et al. 2002). The results of these studies indicate that bone marrow-derived stem cells can beneficially increase perfusion in ischemic myocardium. In addition, transendocardial injection of bone marrow cells is a safe and effective treatment option for end-stage heart failure patients.
4.2 Endothelial Progenitor Cells
Endothelial progenitor cells (EPCs) can be identified in adult peripheral blood, bone marrow, and human umbilical cord blood. Because EPCs have in common with hematopoietic stem cells certain cell surface antigens, they are considered to derive from a common precursor (Kalka et al. 2000). Current experiments suggest that EPCs play an important role in vasculogenesis by differentiating into vascular endothelial cells, inhibiting ventricular remodeling through improvement in myocardial blood supply instead of increasing the number of contracting muscle cells as skeletal myoblasts or ES cells do (Kamihata et al. 2001). Combination therapy with cardiogenesis and vasculogenesis by cell transplantation is now being considered as a novel promising strategy for repairing the ischemic damaged heart.
4.3 Adipose Tissue-Derived Stem Cells
There are major limitations in the use of adult stem cells harvested from essential organs such as muscle, skin, brain. liver and bone marrow. The pain and expense associated with the procedures, and the risk of donor site tissue morbidity are significant concerns. For the bone marrow, traditional tissue procurement procedure frequently requires general or spinal anesthesia and may yield low numbers of stem cells upon processing (approximately 1 bone marrow-derived stem cell per 105 adherent stromal cells) (Pittenger et al. 1999). From a practical standpoint, low stem cell numbers necessitate an ex vivo expansion step to obtain clinically significant cell numbers. Such a step is time consuming, expensive, and risks cell contamination and loss. Adipose tissue may represent an ideal source of autologous stem cells since easy to obtain by collagene digestion, results in minimal patient discomfort, yet capable of yielding cell number substantial enough to obviate extensive expansion in culture. Humans have abundant subcutaneous or abdominal fat deposits, and adipose tissue can be easily removed by aspiration from these locations. The adipose tissue, like the bone marrow, is derived from the embryonic mesoderm and contains a heterogeneous stromal cell population, including mesenchymal stem cells (Zuk et al. 2001) and endothelial cell progenitors (Miranville et al. 2004). Injection of ADSCs has been recently shown to improve neovascularisation in the ischemic hindlimb (Miranville et al. 2004) and the formation of osteoid matrix in immune-tolerant mice (Hicok et al. 2004). In the same way, ADSCs increased the functional capacity of damaged skeletal muscle in vivo (Bacou et al. 2004).
ADSCs have been shown to exhibit in vitro differentiation into the cardiomyocyte lineage (Rangappa et al. 2003). In these reports, different approaches have been used. Rangappa et al. described first the appearance of beating cells upon treatment with 5-azacytidine (Rangappa et al. 2003). They treated twice-passaged ADSCs with 5-azacytidine at 1, 3, 6, and 9 μmol/L and incubated for 12, 24, 48 or 72 h. After the respective incubation periods, the cells were washed and replaced with fresh RPMI medium (Gibco, St. Louis CA). The cells were observed daily, and the medium changed once every 3 days, until the experiment was terminated at 2 months. At 1 week, after treatment with 5-azacytidine, the cells began to change their morphology, by showing multinucleation. At 2 weeks, 30 % of the cells aggregated and formed a ball-like appearance. At 3 weeks, cell aggregates began to beat spontaneously. Immunostaining against cardiac-specific markers showed strong positivity for myosin heavy chain, α-sarcomeric actinin and troponin I in the beating cell aggregates. Planat-Benard and colleagues described spontaneous cardiomyocyte differentiation of ADSCs from primary culture, without any chemical treatment (Planat-Benard et al. 2004). In their protocol, isolated stromal vascular fraction (SVF) cells from the adipose tissue were directly plated in semisolid methylcellulose medium (MethoCult, Stem Cell Technologies, Vancouver CA) without previous cell expansion or culture selection. Beginning at 6 days of culture, the emergence of clones with rounded cells and small tube cells was identified. From these clones, some rounded cells independently started a contractile activity at days 11–14 after plating. After 20–30 days, cohesive groups of cells appeared, with the presence of branching fibers and sharing tight connections, all beating at a single rate. The percentage of beating clones counted at 20 days were from 0.02 to 0.07 % of plated SVF cells. The cardiomyocyte phenotype was confirmed by assessing the expression of specific cardiac markers, immunocytochemistry staining and ultrastructural analysis, revealing the presence of ventricular and atrial type of cells. Electrophysiological studies revealed a pacemaker activity of the cells. Functional studies showed that adrenergic agonists stimulated the beating rate, whereas cholinergic agonist decreased it.
Culture-expanded cells in control medium, isolated from the bone marrow (bone marrow-derived stromal cells, BMSCs), and from the adipose tissue (ADSCs) have been shown to give rise to apparently homogeneous populations with similar cell size and cell surface markers (Morizonoc et al. 2003). For the analysis at flow cytometry, primary cultures of BMSCs and ADSCs were expanded until passage 4 in control medium (Dulbecco’s modified Eagle’s medium, 10 % fetal bovine serum, 1 % antibiotic-antimycotic, all from Gibco) (Morizonoc et al. 2003). Both populations have been shown to express CD13, CD29 (β1-integrin), CD44, CD58, CD90, CD105 (endoglin) and CD166. SH-3, which recognizes an epitope present on CD73 (ecto-5′-nucleotidase), is uniformly positive for both ADSCs and BMSCs, and may act to mediate cell-cell interaction (Barry et al. 2001). STRO-1, a marker for cells with multi-lineage potential, is also expressed, albeit at low levels, by both ADSCs and BMSCs (Simmons and Torok-Storb 1991; Gronthos et al. 1994). Hovewer, certain key differences in surface marker expression between marrow and adipose-derived cells are present. Differences are in the expression of adhesion molecules with know function in the homing and mobilization of hematopoietic stem cells. For example, ADSCs express CD48d (α4 integrin, which forms a heterodimer with CD29 to create very late activation antigen-4, VLA-4), while cells derived from the bone marrow do not. The pattern of expression of CD106 (VCAM-1), a VLA-4 ligand, is reversed. Interestingly, it has been observed an inverse pattern of expression of VLA-4 and its cognate receptor VCAM-1 in adipose tissue and bone marrow-derived cells. Thus, ADSCs express CD106/VCAM-1 but not CD49d/VLA-4, while BMSCs express CD49d/VLA-4 but not VCAM-1 (Morizonoc et al. 2003). Similarly, ADSCs express high levels of CD54 (ICAM-1), while BMSCs have minimal expression of this molecule. This is an intriguing observation, given the important role of the interaction between these molecules in stem cell homing to and mobilization from the bone marrow (Papayannopoulou et al. 2001; Sudhoff and Sohngen 2002). By contrast, CD44, a hyaluronic acid/fibronectin receptor also implicated in hematopoietic stem cell adhesion, proliferation and mobilization, is expressed on both BMSCs and ADSCs (Kronenwett et al. 2000).
Yet, while the general the pattern of mesenchymal lineage differentiation in basal control medium of ADSCs and BMSCs is very similar, some differences have been observed upon incubation in differentiating medium. In osteogenic differentiation medium, alkaline phosphatase activity is significantly greater in ADSCs, while mineralization is more extensive within BMSCs. Both cell populations express mRNA specific for collagen type I, osteocalcin, osteonectin, osteopontin, bone morphogenetic protein(BMP)-1, parathyroid hormone receptor, retinoic acid X receptor(RXR)α, vitamin D and CBFA-1, a transcriptional factor that regulates multiple osteogenic genes (Zuk et al. 2001). The in vitro chondrogenesis from both ADSCs and BMSCs is very similar, with micromasses of cells from both deposits, producing a proteoglycan rich, collagen type II-containing extracellular matrix with chondroitin-4-sulfate and keratin sulphate, two predominant glycosaminoglycans expressed in cartilage proteoglycan (Zuk et al. 2001; Huang et al. 2002). Despite the differences listed above, the similarities between stem cells extracted from the bone marrow and the adipose tissue suggest the potential for adipose tissue to act as an alternate, perhaps preferred, cell source for clinical application (Fraser 2002). Therapeutic enhancement of neovascularization is one of the most important strategies needed to limit the complications of postischemic injury . Considerable efforts have focused on the development of therapeutic strategies designed to increase vessel growth in the setting of ischemia. Particularly, transplantation of bone marrow-derived mononuclear cells (BM-MNC ) has been shown to stimulate neovascularization after experimental ischemic injury, resulting in long-term salvage and survival of viable tissue (Mendez-Ferrer et al. 2006). The use of BM-MNC is now under intense investigation in humans, and the results of early small and uncontrolled studies point to a great potential for such therapy to limit disease progression (Chien 2006; Bartunek et al. 2007). Several researchers have showed that the administration of adipose lineage cells can potentially affect revascularization to a similar extent as BM-MNC administration (Miranville et al. 2004; Planat-Benard et al. 2004; Nakagami et al. 2006). Nakagami and collegues pointed out on the capability of ADSCs to secrete multiple angiogenic growth factors, such as VEGF and hepatocyte growth factor (HGF) (Nakagami et al. 2005). They showed that ADSCs secrete angiogenic growth factors that strongly induce endothelial growth, migration and tube formation. Transplantation of these cultured ADSCs to ischemic limbs accelerated angiogenesis mainly caused by secretion of growth factors, rather that participation in vessel formation by differentiation. Recent reports demonstrated that these ADSCs can be induced to differentiate into cardiac myocytes, which could be excellent for regenerative cell therapy for severe heart failure (Rangappa et al. 2003; Planat-Benard et al. 2004). The first and consistent report so far on the in vivo use of ADSCs in cardiac cell transplantation is from Miyahara et al. (2006). Miyahara and collegues (2006), using cell sheet technology , demonstrated that monolayered adipose tissue derived mesenchymal stem cells have multipotent and self-propagating properties after transplantation into infarcted rat hearts. Four weeks after coronary ligation, they transplanted the monolayered mesenchymal stem cells (MSCs) onto the scarred myocardium. After transplantation, the engrafted sheet gradually grew to form a thick stratum that included newly formed vessels, undifferentiated cells and few cardiomyocytes. The mesenchymal stem cell sheet also acted through paracrine pathways to trigger angiogenesis. They have also shown that transplantation of the monolayered MSCs significantly increased left ventricule maximum diastolic velocity (−dp/dt max), decreased the left ventricule end-diastolic pressure (LVEDP) and inhibited the development of left ventricle enlargement in rats with chronic heart failure secondary to myocardial infarction. These results suggest that transplantation of adipose tissue-derived monolayered MSCs improves cardiac function. However in this study the presence of cardiomyocytes within the MSC tissue seemed to be rare. Thus, the improvement of left ventricule function described here may be explained mainly by growth factor-mediated paracrine effects of the MSC sheet and a decrease in left ventricle wall stress resulting from the thick MSC. Although all these numerous studies have provided evidence that adipose tissue-derived stromal cells (ADSCs) contain a population of adult multipotent mesenchymal stem cells capable to offer a therapeutical potential for repairing damaged tissues, such as the ischemic myocardium , a number of fundamental issues need to be addressed before this approach can be considered for translational studies. First, although the majority of patients with acute myocardial infarction (MI) undergo spontaneous or iatrogenic reperfusion, the ability of ADSCs to improve cardiac function in the setting of a reperfused infarction has never been tested. The literature is replete with examples of therapies that work in the presence of a transient but not permanent coronary occlusion. Consequently, it is of utmost importance to determine whether ADSCs are effective when coronary occlusion is followed by reperfusion, an event that dramatically alters the milieu of the myocardial interstitium and of the myocardium itself. Second, improvement in left ventricular function has been shown when stem cells are injected intramyocardially in the peri-infarct area, an approach that would be difficult in patients. Clinically, the most practical route for ADSC administration is intravascular delivery, but it is unknown whether ADSCs injected into the coronary circulation can cross the vessel wall, translocate to the infarcted region, and initiate effective myocardial regeneration. Third, the mechanism whereby ADSCs improve cardiac function (differentiation into cardiac cells vs. fusion vs. paracrine effects on preexisting cells) remains poorly understood. Finally, the advantage of using total unfractionated ADSCs—compared with purified populations of ADSCs, such as the CD34+-purified ADSCs, remains to be determined.
< div class='tao-gold-member'>
Only gold members can continue reading. Log In or Register a > to continue
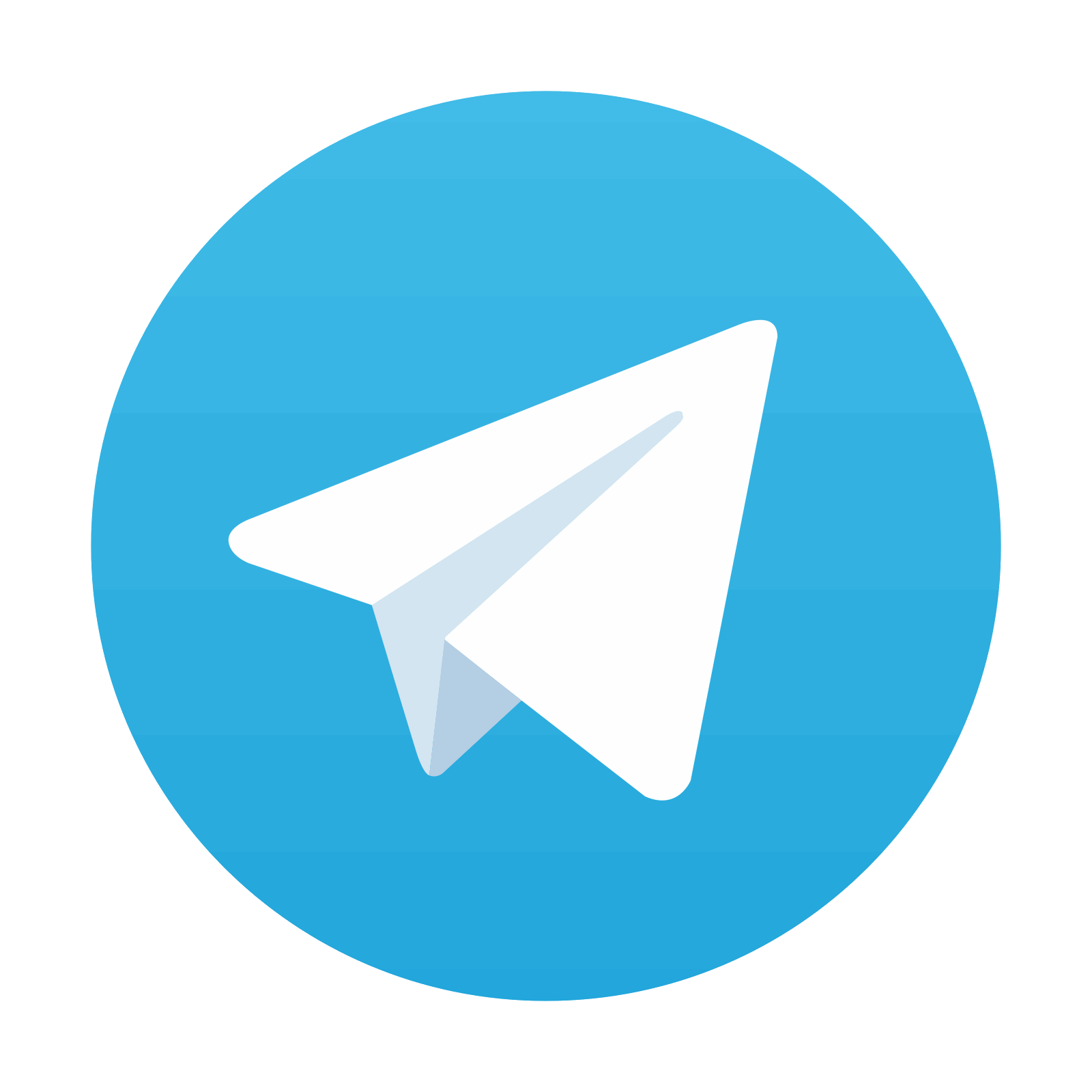
Stay updated, free articles. Join our Telegram channel

Full access? Get Clinical Tree
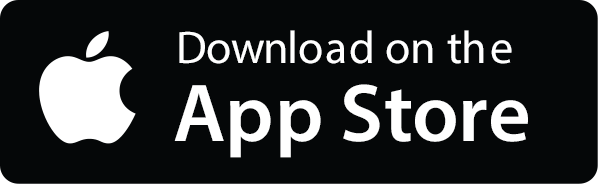
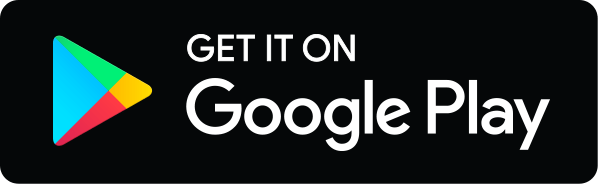