Previous studies have shown that tissue Doppler imaging (TDI) is able to identify mutation carriers of hypertrophic cardiomyopathy (HC) before the development of the clinical phenotype. However, data are scarce and have sometimes been controversial. We performed a systematic study that included conventional echocardiography, TDI, and plasma NT-probrain natriuretic peptide (NT-proBNP) measurement to evaluate the parameters that could identify HC mutation carriers. A total of 138 genotyped subjects were included and divided into 3 groups: group 1, those with HC (n = 62); group 2, mutation carriers (first-degree relatives with a positive genotype but negative phenotype; n = 34); and group 3, controls (first-degree relatives with a negative genotype and phenotype; n = 42). An echocardiographic study, including TDI, was performed on all subjects, and a TDI-derived index (global function index) was also determined. The age-adjusted mean differences in the echocardiographic and TDI parameters and NT-proBNP levels were compared among the 3 groups. Compared with the HC group, the carriers had significantly higher mean E′ velocities, lower mean E/E′ ratio, higher mean S′ velocities, and lower mean global function index and NT-proBNP values. The carriers and controls did not differ significantly either in the echocardiographic parameters studied or in the NT-proBNP levels. In conclusion, the echocardiographic and TDI parameters and NT-proBNP levels cannot be used to identify the HC mutation carrier state and therefore do not appear to be reliable for the purpose of making a preclinical diagnosis of the disease.
Sarcomeric hypertrophic cardiomyopathy (HC) is a relatively common cardiomyopathy with an estimated prevalence of 1:500 in the general population according to the presence of the clinical phenotype. The early diagnosis of HC mutation carriers—relatives with a positive genotype but with no detectable left ventricular (LV) hypertrophy (LVH) found on imaging studies—is important, because this population is at risk of developing cardiac fibrosis and hypertrophy and, therefore, requires periodic clinical follow-up. Furthermore, genetic counseling is compulsory, and studies are ongoing of pharmacologic interventions for the future prevention of hypertrophy. Genetic testing is the ideal strategy for the identification of mutation carriers, but it requires the identification of the causal mutation in the index patient. Moreover, this process remains expensive, is not always available, and will fail to identify causal mutations in 30% to 40% of probands. The early identification of HC mutation carriers using standardized echocardiographic indexes is an attractive prospect for exploration. In addition, plasma NT-probrain natriuretic peptide (NT-proBNP) levels were thought to be potentially useful for the detection of early ventricular changes in carriers before the identification of conventional echocardiographic abnormalities. Early reports with small sample sizes suggested that the systolic and early diastolic peak myocardial velocities, measured using tissue Doppler imaging (TDI), could accurately identify HC before the development of LVH. However, subsequent studies challenged these results, suggesting that the TDI velocities were not accurate enough to be used as a sole diagnostic criterion. The global functional index, a TDI-derived index, has been described as high in patients with HC, identifying regional systolic and diastolic dysfunction ; however its usefulness in the detection of mutation carriers has never been studied. Because of to the conflicting data in this important area, we performed a prospective observational study to investigate the parameters that could routinely identify HC mutation carriers, including conventional and TDI echocardiographic indexes, the global functional index, and the biomarker NT-proBNP, in genotyped adults and children from families with HC.
Methods
The population included 138 subjects of Caucasian origin recruited from families with HC and followed up in an outpatient setting at the Cardiomyopathy Unit of a University Hospital. The participants were assigned to 3 groups. Group 1 included 62 patients with HC according to major echocardiographic criteria, previously reported, and defined by a maximal LV end-diastolic wall thickness of ≥15 mm. These patients (32 females and 30 males, aged 48.7 ± 16.9 years [age cutoff 13 and 82 years]) had all undergone genotyping, and 46 (74%) had pathogenic mutations identified in the sarcomeric genes. Group 2 included the mutation carriers, 34 genotyped first-degree relatives of patients with HC with identified mutations (21 females and 13 males, aged 26.8 ± 15.5 years (age cutoff 4 and 68 years). The mutation was present in their families but neither LVH detected by echocardiography nor electrocardiographic abnormalities were present. Group 3, the control group, included 42 negative phenotype and genetically unaffected first-degree relatives of patients with HC (19 females and 23 males, aged 30.1 ± 12.9 years (age cutoff 12 and 59 years).
Patients with previous myomectomy, septal alcohol ablation, atrial fibrillation, or an implanted pacemaker were not included. A complete clinical assessment of all the participants was performed, including a detailed familial history (with analysis of the pedigree back ≥3 generations), standard physical examination, and 12-lead electrocardiographic and echocardiographic studies. On the same day, laboratory testing was performed, including renal function and muscular enzymes (for the exclusion of unrecognized renal or muscular involvement), plasma NT-proBNP, and the extraction of blood for genetic studies.
A systematic mutation screening of 5 sarcomeric genes, those most frequently associated with sarcomeric HC ( MYBPC3, MYH7, TNNT2, TNNI3, and MYL2 ), was performed on all the subjects using polymerase chain reaction followed by direct sequencing on an ABI 3100 DNA sequencer, using the BigDye terminator mix (Applied Biosystems, Lisbon, Portugal). When a sequence variant that had not been previously reported (mutation databases: http://genepath.med.harvard.edu and http://www.hgmd.org/ ) was identified in the index patient and was considered as a possible pathogenic mutation, the first-degree relatives were tested for the identified variant to analyze co-segregation. Echocardiographic transthoracic studies were performed using a Vivid I ultrasound system (GE Medical Systems, Waukesha, Wisconsin), and all standard M-mode, 2-dimensional mode, and Doppler, including TDI, measurements were evaluated according to the American Society of Echocardiography guidelines. LV end-diastolic wall thickness measurements were obtained at the septum and posterior wall (parasternal long-axis view); anterior and posterior septum and lateral and posterior walls (parasternal short-axis view), just below the mitral valve and at the papillary muscle levels; and apical wall (4-chamber apical view). The maximum LV end-diastolic wall thickness was considered the maximum thickness found in any of the segments considered. A LVH score, defined as the sum of the 8 “short-axis” segments’ thicknesses plus the apical segment thickness divided by 9, was used as an overall benchmark for the extent of LVH. The global functional index, defined as [(E:E′):S′], was determined in the 3 groups at the 4 levels (septal, lateral, anterior, and inferior). All echocardiographic studies were performed by 1 cardiologist and reviewed by another, both of whom were experienced in the technique and unaware of the results of the genetic status of the participants.
The institutional ethics committee approved the study, and all participants provided written informed consent. In the case of children, their parents provided the written informed consent.
Age, echocardiographic parameters (M-mode, 2-dimensional mode, and TDI), and the logarithm of the plasma NT-proBNP levels are reported as measures of central tendency (mean ± SD), and gender is reported through absolute frequencies. One-way analysis of variance and the chi-square test were used to compare the control, mutation carrier, and HC groups for mean age and gender homogeneity, respectively. A post hoc test was conducted (least significant difference test) to account for the 2-by-2 group comparison regarding age. The echocardiographic and TDI parameters and the logarithm of the plasma NT-proBNP levels were analyzed using a multiple linear regression model with 1 term for age and 2 for groups, coded by reference and omitting carriers to control for the age differences among the groups. Before the execution of the multiple linear regression models, R 2 values were measured to evaluate the linear and quadratic trends between the echocardiographic and TDI parameters and the logarithm of the plasma NT-proBNP levels and age. When an improvement of ≥50% was found in the R 2 value, from a linear to a quadratic trend, a quadratic term for age was introduced into the multiple linear regression model. We chose 50% improvement in the R 2 value, because it was the minimum percentage found to offset the complexity introduced to the multiple linear regression model by the quadratic age term. Also, before the execution of the multiple linear regression models, the interaction between age and group was analyzed, introducing an interaction term to the multiple linear regression model. If this term or terms were statistically significant, we considered that an interaction existed between age and group, and a final multiple linear regression model (without interaction terms) was not executed, because it would not have been meaningful. When the interaction-free multiple linear regression model was statistically significant, the unstandardized coefficients (and their 95% confidence intervals) of the 2 dummy variables for the groups were evaluated. These represent the estimated age-adjusted mean differences in echocardiographic and TDI parameters and logarithm of the NT-proBNP levels between the control or carrier group (depending on the dummy variable considered) and the HC group. The negative and positive predictive values, specificities, and sensitivities were computed for E′ velocities (lateral and septal) and E/E′ ratio. All the necessary assumptions for the conducted statistical tests were met. The significance level for all the statistical tests was 5%. The statistical analysis was conducted using PASW Statistics, version 20 (SPSS, Chicago, IL).
Results
Group 1 (HC group) included 62 patients, 46 (74%) with identified pathogenic mutations in the screened sarcomere genes. These patients came from 43 unrelated pedigrees (30 with familial disease and 13 with sporadic HC). Mutations were identified in 23 pedigrees with familial disease (41 patients) and 5 patients with sporadic HC. Of the 41 patients with familial HC, the MYBPC3 gene was affected in 18 patients (11 different mutations), the MYH7 gene in 13 patients (5 different mutations), the TNNT2 gene in 8 patients (4 different mutations), and the TNNI3 gene in 1 patient. In those with sporadic HC, 4 patients had mutations in the MYBPC3 gene and 1 had a mutation identified in the MYH7 gene. No mutations were found in the MYL2 gene. Group 2 included 34 carriers. Most of these carriers (n = 28) were first-degree relatives of the patients included in group 1, and 6 belonged to families with HC whose affected parents (also with identified pathogenic mutations) were not included in the present study. Of the 34 subjects in the carrier group, 27 had mutations in the MYBPC3 gene (16 different mutations), 4 had mutations in the TNNT2 gene (3 different mutations), 2 had a mutation in MYH7 gene, and 1 had a mutation in the TNNI3 gene. All carriers were heterozygous for the identified mutations. Group 3 (control group) included 42 subjects, all of whom were asymptomatic, with no LVH, no mitral systolic anterior movement, and no identified mutations. They were first-degree relatives of 21 of the 23 HC families with sarcomere-identified mutations in group 1. The mean age in the HC group was significantly older (p <0.001) than in the other 2 groups. The mean age did not differ significantly between the carrier and control groups (p = 0.357). The proportion of males and females was not significantly different statistically across the 3 groups (p = 0.355).
The mean values for the septal and posterior LV end-diastolic wall thickness, maximal LV end-diastolic wall thickness in a segment, and LVH score were significantly higher in the HC group than in the carrier group (age-adjusted p <0.001 for all comparisons). However, no significant differences were found the between carriers and controls for these parameters. The LV end-systolic diameter and shortening fraction were also not significantly different statistically between the carrier and control groups or between the carrier and HC groups. The LV end-diastolic diameter and left atrial diameter were not compared because of the group–age interactions ( Table 1 ).
M-Mode/2D Data | Group Unadjusted Mean ± SD | Age-Adjusted Mean Difference | |||||||||
---|---|---|---|---|---|---|---|---|---|---|---|
HC (n = 62) | Carriers (n = 34) | Controls (n = 42) | Sample Total (n = 138) | p Value ∗ | |||||||
Controls vs Carriers | HC vs Carriers | ||||||||||
Mean Difference | 95% CI | p Value | Mean Difference | 95% CI | p Value | ||||||
Interventricular septal thickness (mm) | 19.92 ± 5.23 | 9.40 ± 2.04 | 9.34 ± 1.69 | 14.11 ± 11.85 | <0.001 | −0.241 | −1.995 to 1.512 | 0.786 | 9.800 | 7.945 to 11.656 | <0.001 |
Left posterior wall thickness (mm) | 10.67 ± 1.87 | 8.03 ± 1.50 | 8.11 ± 1.24 | 9.24 ± 2.06 | <0.001 | −0.034 | −0.770 to 0.703 | 0.928 | 2.133 | 1.354 to 2.913 | <0.001 |
LV end-diastolic diameter (mm) | 44.80 ± 6.46 | 42.69 ± 5.93 | 47.70 ± 4.52 | 45.16 ± 6.06 | NA | — | — | — | — | — | — |
LV end-systolic diameter (mm) | 24.91 ± 5.24 | 25.01 ± 4.61 | 27.84 ± 3.46 | 25.83 ± 4.77 | 0.002 | 2.343 | 0.202 to 4.485 | 0.320 | −1.451 | −3.705 to 0.804 | 0.205 |
Left atrial diameter (mm) | 44.46 ± 7.74 | 33.74 ± 5.15 | 34.35 ± 4.16 | 38.74 ± 8.07 | NA | — | — | — | — | — | — |
LV shortening fraction (%) | 44.76 ± 7.48 | 41.19 ± 7.14 | 41.41 ± 4.45 | 42.86 ± 6.79 | 0.004 | 0.136 | −2.931 to 3.204 | 0.930 | 2.155 | −1.074 to 5.384 | 0.189 |
Maximum LV wall hypertrophy (mm) | 22.41 ± 5.50 | 10.69 ± 1.69 | 10.67 ± 1.25 | 15.95 ± 6.99 | <0.001 | −0.141 | −1.920 to 1.638 | 0.876 | 10.752 | 8.870 to 12.634 | <0.001 |
LVH score (9 segments) | 14.45 ± 2.81 | 8.62 ± 1.33 | 8.85 ± 1.18 | 11.31 ± 3.53 | <0.001 | 0.085 | −0.849 to 1.019 | 0.857 | 4.845 | 3.857 to 5.833 | <0.001 |
∗ p Value for interaction between age and group (multiple linear regression analysis of variance).
The patients with HC had significantly lower E′ velocities at the mitral annulus (age-adjusted p <0.001 for all comparisons) than the carriers (lateral, anterior, and inferior levels), but the A′ velocities at the mitral annulus did not differ significantly ( Figure 1 and Table 2 ). The E/E′ values were significantly higher in those with HC than in the carriers at all levels (septal, age-adjusted p = 0.001; lateral, age-adjusted p = 0.002; anterior, age-adjusted p = 0.005; inferior, age-adjusted p <0.001; Figure 2 and Table 2 ). Also, the S′ velocities at the mitral annulus were significantly lower in the HC group than in the carrier group (septal, age-adjusted p = 0.028; lateral, age-adjusted p = 0.045; anterior, age-adjusted p = 0.050; inferior, age-adjusted p = 0.043; Figure 3 and Table 2 ). The carrier and control groups did not have statistically significant difference in the E′, S′, and A′ velocities and E/E′ values, at all levels ( Table 2 and Figures 1 to 3 ). The HC group had higher global functional index mean values than the carrier group at the septal (age-adjusted p = 0.003), lateral (age-adjusted p = 0.012), and inferior (age-adjusted p = 0.001) levels of the mitral annulus. However, the global functional index did not differ significantly between the carrier and control groups ( Table 2 and Figure 4 ). Additionally, we tested the diagnostic accuracy of the 3 TDI parameters most widely used in clinical practice—the lateral and septal E′ velocities (cutoff value <10 cm/s and <8 cm/s, respectively) and the E/E′ ratio (cutoff value ≥8). We found that none should be used as a diagnostic tool for differentiating carriers from controls. A lateral E′ of <10 cm/s had a negative predictive value of 58%, a positive predictive value of 0%, specificity of 98%, and sensitivity of 0%. A septal E′ of <8 cm/s gave a negative predictive value of 58%, positive predictive value of 33%, specificity of 96%, and sensitivity of 3%. An E/E′ ratio of ≥8 gave a negative predictive value of 60%, positive predictive value of 50%, specificity of 86%, and sensitivity of 20%. Thus, these parameters have the characteristics of a poor diagnostic test (relatively low negative and positive predictive values and very low sensitivity for high specificity).
Group Unadjusted Mean ± SD | Age-Adjusted Mean Difference | ||||||||||
---|---|---|---|---|---|---|---|---|---|---|---|
HC (n = 62) | Carriers (n = 34) | Controls (n = 42) | Sample Total (n = 138) | p Value ∗ | |||||||
Controls vs Carriers | HC vs Carriers | ||||||||||
Mean Difference | 95% CI | p Value | Mean Difference | 95% CI | p Value | ||||||
Septal S′ (cm/s) | 7.08 ± 1.87 | 8.38 ± 1.57 | 8.62 ± 2.06 | 7.86 ± 1.99 | <0.001 | 0.009 | −0.857 to 0.876 | 0.983 | −1.025 | −1.937 to −0.112 | 0.028 |
Septal E′ (cm/s) | 6.68 ± 2.62 | 12.09 ± 2.99 | 12.30 ± 2.90 | 9.68 ± 3.92 | NA | — | — | — | — | — | — |
Septal A′ (cm/s) | 7.86 ± 2.32 | 7.80 ± 1.68 | 8.30 ± 2.26 | 7.99 ± 2.17 | 0.011 | 0.100 | −0.919 to 1.120 | 0.846 | −0.771 | −1.844 to 0.303 | 0.158 |
Septal E/E′ | 12.35 ± 5.98 | 7.25 ± 2.28 | 6.95 ± 2.02 | 9.47 ± 5.05 | <0.001 | −0.307 | −2.347 to 1.733 | 0.766 | 3.878 | 1.723 to 6.033 | 0.001 |
Septal GFI | 2.01 ± 1.46 | 0.92 ± 0.44 | 0.84 ± 0.32 | 1.39 ± 1.17 | <0.001 | −0.090 | −0.577 to 0.397 | 0.714 | 0.782 | 0.269 to 1.295 | 0.003 |
Lateral S′ (cm/s) | 7.97 ± 2.63 | 10.09 ± 2.48 | 9.96 ± 2.07 | 9.08 ± 2.62 | <0.001 | −0.088 | −1.219 to 1.042 | 0.877 | −1.222 | −2.416 to −0.028 | 0.045 |
Lateral E′ (cm/s) | 8.98 ± 3.73 | 15.83 ± 3.74 | 16.27 ± 3.50 | 12.82 ± 5.09 | <0.001 | 0.518 | −0.982 to 2.019 | 0.496 | −4.425 | −6.010 to −2.840 | <0.001 |
Lateral A′ (cm/s) | 9.43 ± 3.09 | 8.68 ± 1.94 | 8.68 ± 2.39 | 9.02 ± 2.66 | 0.005 | −0.164 | −1.402 to 1.075 | 0.794 | −0.302 | −1.610 to 1.007 | 0.649 |
Lateral E/E′ | 9.43 ± 4.94 | 5.47 ± 1.54 | 5.40 ± 2.06 | 7.25 ± 4.10 | <0.001 | −0.111 | −1.812 to 1.590 | 0.898 | 2.878 | 1.081 to 4.675 | 0.002 |
Lateral GFI | 1.50 ± 1.36 | 0.59 ± 0.26 | 0.57 ± 0.31 | 1.00 ± 1.05 | <0.001 | −0.034 | −0.486 to 0.417 | 0.880 | 0.610 | 0.134 to 1.085 | 0.012 |
Anterior S′ (cm/s) | 7.28 ± 2.43 | 9.59 ± 2.14 | 9.70 ± 2.39 | 8.54 ± 2.71 | <0.001 | 0.191 | −0.978 to 1.360 | 0.747 | −1.244 | −2.487 to 0.000 | 0.050 |
Anterior E′ (cm/s) | 7.77 ± 3.41 | 14.49 ± 4.12 | 15.11 ± 2.85 | 11.53 ± 4.92 | <0.001 | 0.792 | −0.711 to 2.295 | 0.299 | −4.395 | −5.993 to −2.797 | <0.001 |
Anterior A′ (cm/s) | 8.61 ± 3.28 | 8.78 ± 2.14 | 8.14 ± 2.02 | 8.51 ± 2.69 | 0.076 | — | — | — | — | — | — |
Anterior E/E′ | 10.93 ± 5.84 | 6.21 ± 2.14 | 5.68 ± 1.53 | 8.22 ± 4.86 | <0.001 | −0.665 | −2.690 to 1.359 | 0.516 | 3.144 | 0.981 to 5.307 | 0.005 |
Anterior GFI | 2.01 ± 2.16 | 0.73 ± 0.47 | 0.63 ± 0.29 | 1.30 ± 1.63 | <0.001 | −0.158 | −0.883 to 0.568 | 0.667 | 0.658 | −0.115 to 1.431 | 0.094 |
Inferior S′ (cm/s) | 7.67 ± 2.13 | 9.23 ± 1.50 | 9.40 ± 1.59 | 8.57 ± 2.01 | <0.001 | 0.207 | −0.674 to 1.087 | 0.643 | −0.970 | −1.911 to −0.029 | 0.043 |
Inferior E′ (cm/s) | 7.47 ± 3.17 | 14.57 ± 3.12 | 13.90 ± 2.69 | 11.10 ± 4.50 | <0.001 | −0.470 | −1.806 to 0.867 | 0.488 | −5.349 | −6.778 to −3.921 | <0.001 |
Inferior A′ (cm/s) | 8.72 ± 2.33 | 8.90 ± 2.00 | 8.97 ± 1.95 | 8.84 ± 2.13 | NA | — | — | — | — | — | — |
Inferior E/E′ | 11.31 ± 5.70 | 5.95 ± 1.61 | 6.11 ± 1.57 | 8.43 ± 4.78 | <0.001 | 0.017 | −1.936 to 1.970 | 0.986 | 4.388 | 2.300 to 6.475 | <0.001 |
Inferior GFI | 1.73 ± 1.30 | 0.66 ± 0.24 | 0.68 ± 0.24 | 1.15 ± 1.05 | <0.001 | −0.018 | −0.453 to 0.417 | 0.936 | 0.775 | 0.311 to 1.239 | 0.001 |
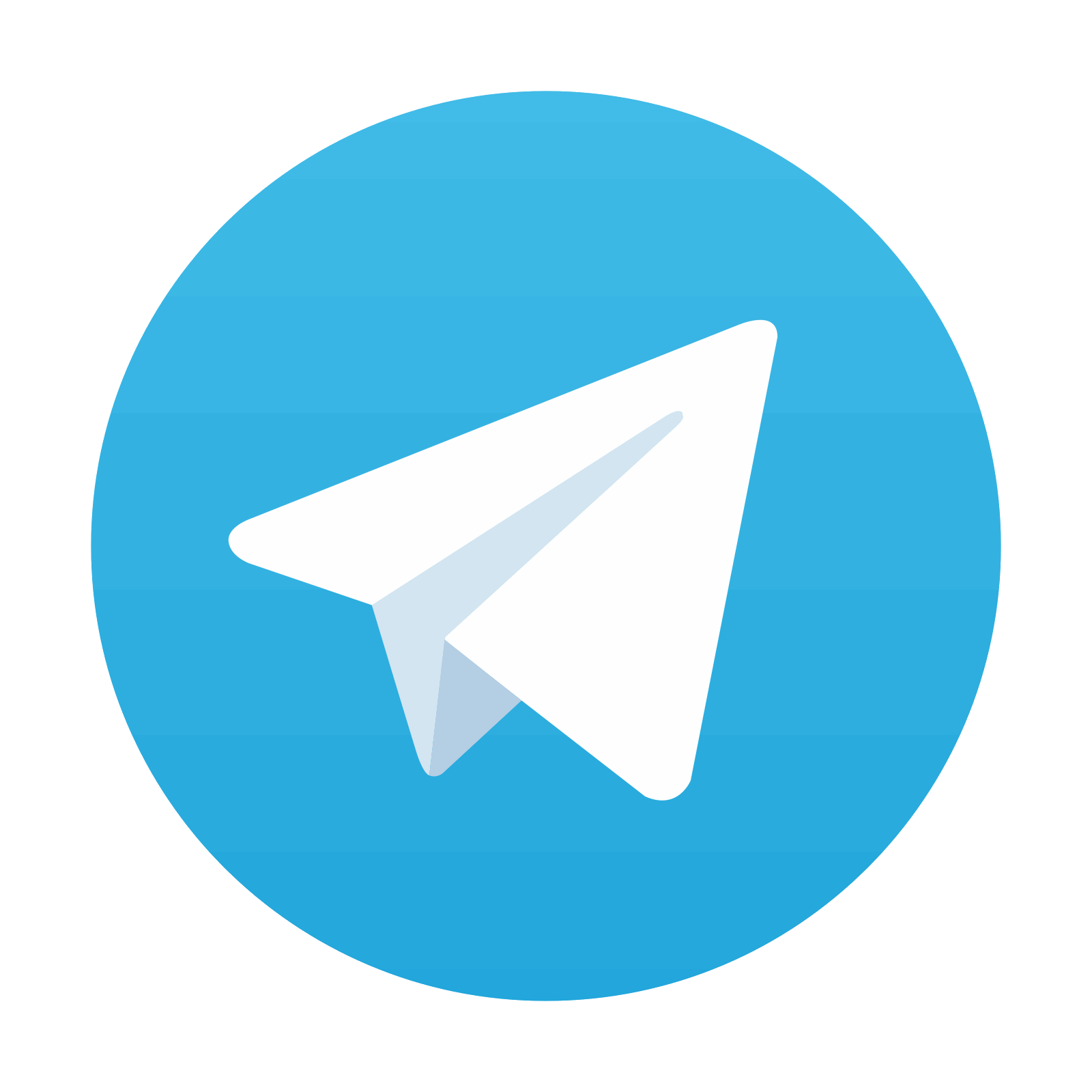
Stay updated, free articles. Join our Telegram channel

Full access? Get Clinical Tree
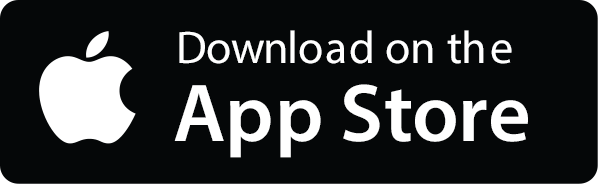
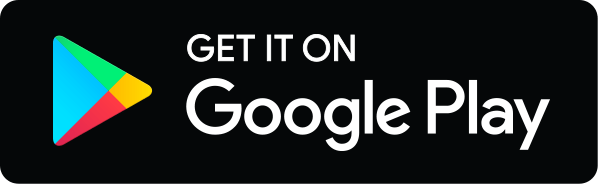
