Summary
Three-dimensional (3D) echocardiography has improved dramatically due to technical advances in probe design and computer processing. Congenital heart disease demands a detailed understanding of the spatial relationships of cardiac structures to plan treatment, making 3D echocardiography highly attractive. Novel projections of cardiac structures can be achieved that are impossible by two-dimensional methods, and high frequency probes are now available to allow better 3D imaging in small children. The introduction of a 3D transoesophageal echo probe has extended the applications to real-time guidance of catheter procedures. All of these developments mean that 3D echocardiography is now an accepted complementary imaging technique to conventional cross-sectional echocardiography in congenital heart disease. In addition to morphology, 3D echocardiography can analyse ventricular volumes and function with fewer geometric assumptions than cross-sectional techniques. Analysis of myocardial motion, including 3D tracking of wall motion, is an emerging technique that may become important, particularly in long-term follow-up of operated congenital heart disease. Normal ranges of ventricular volumes and synchrony remain to be established in children. Further improvements in image processing, including automation of analyses and tailoring of software to ventricles of abnormal shape, may move such techniques from a research setting into more mainstream clinical practice.
Résumé
L’échographie tridimensionnelle (3D) a progressé de façon très importante, du fait de progrès techniques tant dans les sondes que dans le traitement informatique du signal. L’exploration d’une cardiopathie congénitale exige une compréhension approfondie des relations spatiales des structures cardiaques, faisant de cette approche échographique une technique particulièrement intéressante dans ce contexte. Les nouveaux plans d’exploration des structures cardiaques peuvent être obtenus, jusque-là inacceptables en échographie bidimensionnelle conventionnelle et des sondes de haute fréquence, actuellement disponibles permettent une évaluation échographique tridimensionnelle chez le petit enfant. L’apport des sondes transoesophagiennes 3D a permis d’étendre les applications de cette approche lors des procédures interventionnelles. Tous ces développements ont permis à l’échographie tridimensionnelle d’atteindre la maturité, et cette approche est maintenant considérée comme une technique d’imagerie complémentaire à l’évaluation conventionnelle 2D dans les cardiopathies congénitales. Outre, l’exploration morphologique, l’échographie 3D permet d’analyser et d’évaluer les volumes ventriculaires, la fonction ventriculaire, sans qu’il soit nécessaire de considérer les hypothèses géométriques qu’imposait la méthode conventionnelle. L’analyse de la cinétique segmentaire, y compris l’évaluation 3D automatique de celle-ci, est une technique émergente dont la place est croissante, en particulier dans le suivi au long cours des cardiopathies congénitales opérées. La définition des valeurs normales des volumes ventriculaires demande cependant à être confirmée chez l’enfant. Des améliorations dans le traitement de l’image, incluant leur caractère automatique, ainsi que le développement de logiciels d’évaluation de la géométrie ventriculaire devraient permettre à cette méthode d’exploration de passer naturellement du stade de la recherche aux applications cliniques en pratique quotidienne.
Background
Advances in ultrasound probe technologies, image processing and computing power now mean that three-dimensional (3D) echocardiography is technically feasible with spatial and temporal resolution that was previously unachievable. Treatment of congenital heart defects, whether by surgical or interventional means, demands an understanding of the cardiac lesion to plan the optimal approach. 3D echocardiography has the potential to display normal and abnormal cardiac morphology as a true reflection of the actual anatomy rather than depicting user-defined cross-sectional images. The application of 3D echocardiographic techniques extends beyond cardiac morphology and is being used increasingly to evaluate cardiac function in terms of ventricular volumes, ejection fraction and estimation of ventricular dyssynchrony. The aim of this review is to describe 3D echocardiographic techniques that are currently available and how these are being applied to congenital heart disease.
3D echocardiographic techniques
In the past, 3D echocardiographic images were produced by reconstruction of multiple consecutive cross-sectional images into a 3D echocardiographic dataset. The 3D images that resulted thus represented a ‘virtual’ cardiac cycle and images were not truly real-time. A variety of acquisition techniques were described including the use of rotational transthoracic or transoesophageal echocardiography probes . Although composite 3D images of congenital heart defects were produced, these often had artefacts related to patient movement or due to the reconstruction technique itself ( Fig. 1 ). Importantly, the 3D images produced represented a cardiac cycle that had never truly existed but was a virtual reconstruction from many sequential cardiac cycles acquired by two-dimensional (2D) echocardiography. The acquisition of such data was time consuming, due to the need to wait for the ultrasound probe to rotate through a large angle (typically 180 degrees) in 2–3 degree increments. Post-processing was also relatively slow and was performed offline, which limited the clinical applicability, and certainly made live guidance of procedures impossible. This approach has been superseded by the development of ‘matrix’ ultrasound probes . Matrix probes have a number of arrays of ultrasound crystals arranged in parallel on top of each other, which transmit and receive the ultrasound signal. The amount of echocardiographic information generated is vastly greater than conventional 2D systems. Some of the post-processing takes place in the probe itself, to reduce the thickness of cables required to attach the probe to the ultrasound system. This has meant that 3D probes have had to be larger than 2D probes, but the very latest generations of 3D ultrasound probes have become much smaller, with a size and footprint similar to 2D probes. Conventional modalities such as 2D, M-mode, colour flow and Doppler functionality have been incorporated into most 3D probes.

In an analogous manner to 2D echocardiography, 3D images of the heart can be visualized ‘live’ within a user-defined area. Alternatively, a ‘full volume’ dataset, incorporating a larger breadth and depth of view may be acquired, usually over several consecutive cardiac cycles. The larger volume dataset requires post-processing either on the ultrasound system or offline. Some 3D ultrasound systems will now allow acquisition of a ‘full volume’ of data within a single cardiac cycle but with lower image resolution. 3D colour flow Doppler is also feasible on the current generation of 3D echocardiographic equipment but usually with a limited field of view and at a lower frame rate. The range of 3D ultrasound probes has increased to include a paediatric matrix probe (with high frequency and small footprint) to permit optimal imaging of smaller infants and children. 3D transoesophageal echocardiography (TOE) has been made possible by the incorporation of a matrix transducer into the TOE probe. The currently available 3D TOE probe, due to its size, is only suitable for patients with a body weight greater than 25–30 kg, which precludes its use in smaller patients. 3D TOE can facilitate the guidance of catheter procedures in real-time as well as the evaluation of surgical repair. For the intraoperative assessment of patients too small for the 3D TOE probe, the alternative of 3D epicardial echocardiographic imaging remains an option .
Potential advantages of the 3D echocardiographic approach
Using 2D echocardiography, in either standard or user-defined planes, the image and its subsequent projection are defined at the time of acquisition. In contrast, the 3D dataset can be cut in an infinite number of planes at any time after acquisition. Furthermore the images can be displayed either as series of multiplanar reformatted images (MPR) or as rendered 3D views. The MPR technique permits the operator to view any cross-sectional cut plane and to move sequentially through the dataset. Rendered 3D views can be produced from planes selected by the operator and have the advantage of showing a depth of field. This allows visualization of the spatial relationship of near-field structures to far-field structures within a single projection. The ability to alter the plane of interrogation without limitation, means that some projections, for example en face views of atrioventricular valves or septal structures, can be produced. Such views cannot be achieved using conventional 2D techniques. Once such 3D projections have been produced, our own preference is to rotate the final images into a consistent anatomical orientation so that structures that are superior anatomically are projected uppermost on the image.
Limitations of 3D techniques
There are several technical limitations to 3D echocardiographic techniques. Currently, the spatial and temporal resolution of 3D echocardiographic images is inferior to cross-sectional techniques. Although there is often a facility to optimize frame rate, the resulting 3D frame rates are typically 25–40 frames per second. This is a particular problem in the early newborn period where heart rates are much higher, at 120–160 beats per minute. Thus, high heart rates coupled with the small size and fast movement of cardiac structures are a particular challenge in early infancy. The limitation of frame rate is important not only with respect to cardiac morphology but also with regard to cardiac function, where precise estimation of end-diastole and end-systole is important to derive indices such as ejection fraction and dyssynchrony index. 3D echocardiography should therefore be regarded as complementary to, not substituting for, 2D echocardiography. If a 3D volume of echocardiographic data is acquired over several cardiac cycles, the timing of acquisition is linked to the QRS complex on the electrocardiogram. Significant arrhythmia or patient movement can then create ‘stitch’ artefacts between different segments of the full 3D echocardiographic volume.
Patient selection for 3D echocardiography
Despite the technical advances in 3D echocardiography, the basic principles of ultrasound, relating to probe frequency, ultrasound penetration and axial versus lateral resolution, still apply. Thus, for optimal imaging, good acoustic windows are important. Intracardiac structures such as atrioventricular valves will be relatively easy to visualize whereas acoustically inaccessible structures such as intrapulmonary vessels will not be imaged well regardless of the ultrasound technique used. The introduction of a transoesophageal ultrasound probe has overcome some of the problems of acoustic windows, particularly in older and larger patients. The use of a paediatric matrix probe with a small footprint has also helped to improve image quality in younger children. Patient movement can be a significant problem, particularly in children, necessitating the use of sedation for full volume acquisition. For patients who are mechanically ventilated, a brief suspension of ventilation can optimize image quality by removing respiratory motion.
Suitable cardiac lesions for 3D echocardiography
Patients with congenital heart disease are ideal candidates for imaging using 3D echocardiography. Many surgical and catheter interventions are undertaken in younger children in whom sonographic windows are excellent. Given the range of different congenital heart lesions, and the necessity to understand spatial relationships to plan therapy, congenital heart disease has become a major application of 3D echocardiography. Prominent among the cardiac lesions studied are abnormalities of the atrioventricular valves, atrial septal defects, ventricular septal defects and more complex abnormalities of the cardiac connections. These will be reviewed to demonstrate some of the novel advantages of 3D echocardiography. Guidance of catheter intervention for structural lesions will also be addressed.
The atrioventricular valves
In children, repair of atrioventricular valves is far preferable to replacement because this avoids the need for anticoagulation and obviates the need for replacement of mechanical valves simply to accommodate patient growth. For successful repair to be achieved, an understanding of valve morphology and mechanism of valvular closure is essential to plan repair. The 3D echocardiographic technique provides a depth of field that cannot be achieved using cross-sectional techniques and novel projections of anatomy of the valve can be produced, including en fac e views of the valves. When atrioventricular valves are shown en face , they can either be projected as if being viewed from the ventricle or alternatively as if being viewed from the atrium, which has been termed the ‘surgical’ view. As an example, atrioventricular septal defects can be assessed comprehensively including valve leaflets and the size of the atrial and ventricular components of the defect ( Fig. 2 ). Such comprehensive evaluation involves not only en face visualization of the atrioventricular valves themselves but also en face projections of the atrial and ventricular septums . Use of multiplanar reformatted images, rendered 3D views and colour flow Doppler can provide comprehensive imaging of the lesion, including valve morphology, chordal apparatus, closure mechanism and identification of areas of valvular regurgitation . More subtle aspects of the anatomy of the atrioventricular septal defect such as the angle of the valvular leaflets to the crux of the heart have also been investigated with respect to longer-term valve function .

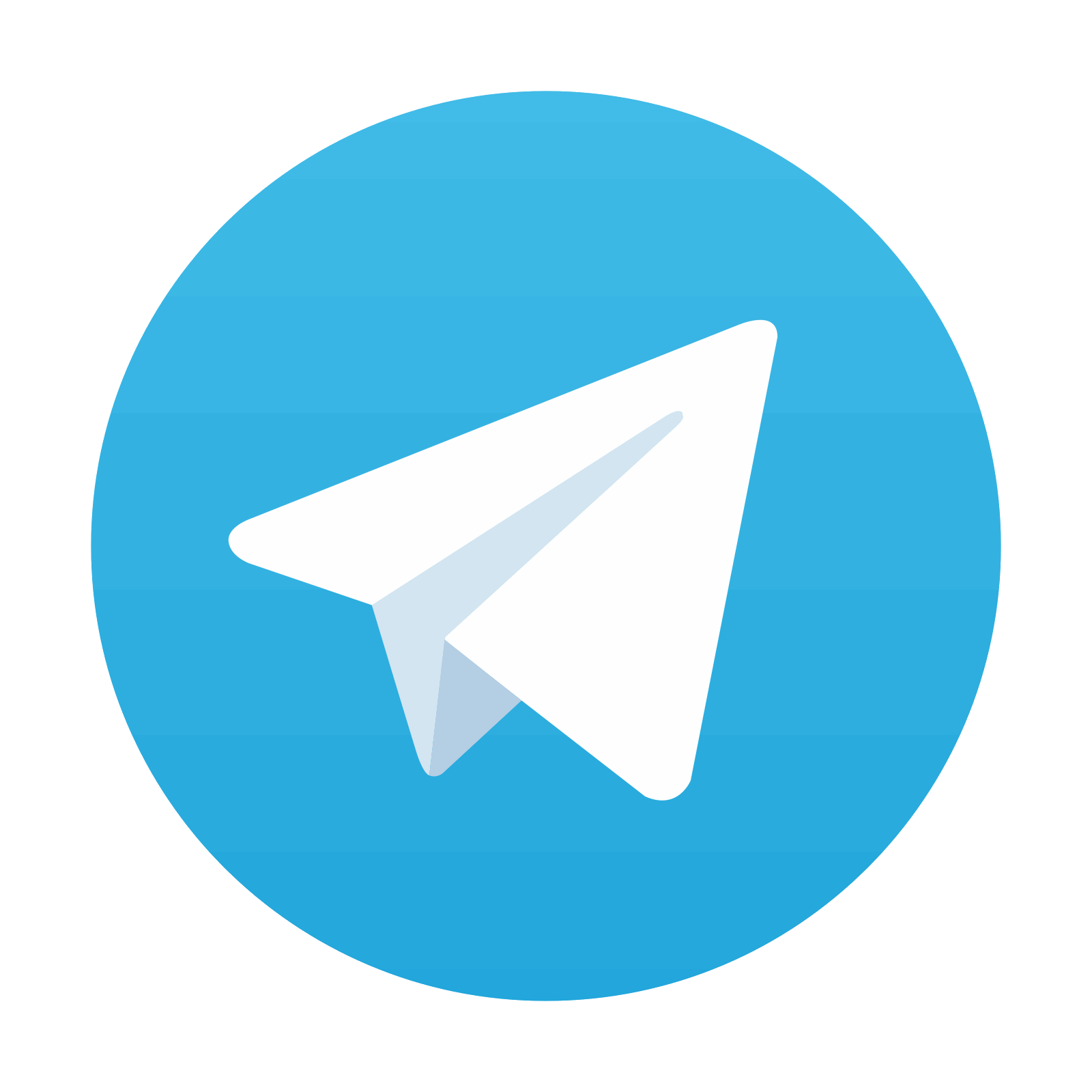
Stay updated, free articles. Join our Telegram channel

Full access? Get Clinical Tree
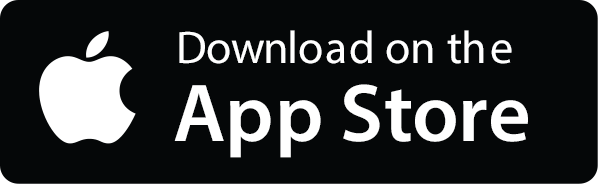
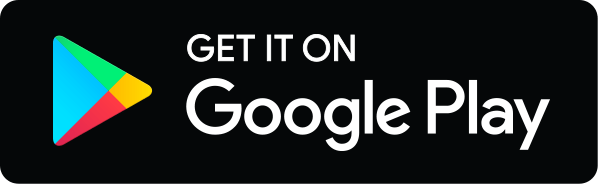
