Background
Venoarterial extracorporeal membrane oxygenation (VA-ECMO) has been proved to effectively rescue patients from refractory cardiogenic shock. The role of the right ventricle in VA-ECMO has been emphasized, but quantitative right ventricular (RV) analysis in this population has been lacking. Three-dimensional echocardiography (3DE) is currently suggested for RV volumetric analysis. The aims of this study were to assess 3DE-derived RV ejection fraction (RVEF) in patients with refractory cardiogenic shock stabilized by VA-ECMO and to explore the association between 3DE-derived RVEF and weaning success as well as the prognosis after the first intent of decannulation.
Methods
Three-dimensional echocardiographic data sets before the first intent of decannulation were retrospectively selected and analyzed in 46 patients who underwent VA-ECMO for refractory acute circulatory collapse.
Results
Twenty-eight of the 46 patients had protocol-defined success in weaning from VA-ECMO. In the success group, both ventricles were smaller and had better pumping function. By stepwise multivariate linear regression, RV free wall strain, left ventricular ejection fraction, RV fractional area change, and central venous pressure were found to be independently associated with RVEF. Receiver operating characteristic curve analysis showed that RVEF had the highest area under the curve (0.90, P < .001) for weaning success with a cutoff value of 24.6%. Worse RVEF (≤24.6%) was also associated with poor prognosis in terms of all-cause mortality within 30 days (hazard ratio, 15.86; 95% CI, 3.56–70.73; P < .001).
Conclusions
Three-dimensional echocardiography–derived RVEF might represent the composite results of RV contractility, left ventricular performance, and fluid status. Under mechanical circulatory support of VA-ECMO, RVEF > 24.6% was associated with higher weaning success and lower 30-day mortality after the first intent of decannulation.
Highlights
- •
Three-dimensional echocardiography-derived RVEF is a feasible and reproducible parameter that can be used to evaluate patients with devastating cardiogenic shock.
- •
The authors offer a cutoff value of RVEF (24.6%) as a useful parameter in decision making for weaning from VA-ECMO.
- •
RVEF before decannulation of VA-ECMO correlated well with 30-day survival after disconnection from VA-ECMO.
- •
The noteworthy application of RVEF might reside in its nature of conveying information as the composite results of RV contractility, fluid status, and LV performance.
Venoarterial extracorporeal membrane oxygenation (VA-ECMO) has been proved to effectively rescue patients with refractory cardiogenic shock as a bridge to recovery, transplantation, or implantation of assist devices. Once patients have been hemodynamically stabilized, a timely decision regarding strategy between bridge to recovery and bridge to transplantation is crucial, because prolonged mechanical circulatory support increases the risk for complications, whereas premature withdrawal may jeopardize outcomes, especially when unanticipated failure of the right ventricle occurs.
The displacement of venous volume into the arterial circulation via VA-ECMO can significantly increase afterload on the left ventricle, thereby potentially reducing left ventricular (LV) stroke volume, increasing myocardial oxygen demand and filling pressure of the left ventricle. In contrast, a VA-ECMO weaning trial that reduces ECMO supporting flow will increase right ventricular (RV) preload but decrease LV afterload, allowing assessment of whether myocardial recovery will tolerate VA-ECMO termination.
Many investigations have been performed to determine echocardiographic parameters for establishing a framework for VA-ECMO weaning. Although LV ejection fraction (LVEF) is an established prerequisite for ceasing VA-ECMO support after assistance for refractory cardiogenic shock, its numeric improvement during the weaning from VA-ECMO is small. As mentioned above, VA-ECMO itself may impede LV unloading and thus the assessment of contractility, whereas the weaning test may merely reduce afterload to unmask LV reserve. By contrast, a significantly lower incidence of RV failure at the time of weaning, although not quantitatively measured, has been found among patients who survived weaning, implicating the important role of the right ventricle.
Because the right ventricle is highly vulnerable to the increased pulmonary load secondary to congestive heart failure, reliable assessments of its size and function are regarded as paramount for effective prognostic evaluations and therapeutic decisions under various conditions. Cardiac magnetic resonance imaging is considered the gold-standard reference for RV volume and ejection fraction (RVEF) but is infeasible for patients under VA-ECMO support. Measurements of RV volumes and RVEF by two-dimensional echocardiography are challenging because of the complicated structure and shape of the right ventricle and are not recommended for clinical use or as a standard reference in research. It is currently suggested that these parameters can be approached with higher accuracy and reproducibility by three-dimensional echocardiography (3DE), which allows direct measurements instead of requiring geometric assumptions.
Accordingly, the purposes of this study were to observe 3DE-derived RVEF in patients with refractory cardiogenic shock stabilized by VA-ECMO and to explore the association between 3DE-derived RVEF and weaning success as well as the prognosis after the first intent of decannulation.
Methods
Study Population, Data Collection, and Definitions
We retrospectively selected and analyzed a population from our ECMO database from August 2014 to September 2015 during which 3DE was performed to evaluate the ventricular function before VA-ECMO decannulation. Eligible patients were ≥18 years of age and were hemodynamically stabilized by VA-ECMO after a circulatory collapse refractory to conventional cardiopulmonary resuscitation and medical treatment. For the satisfactory acquisition of 3DE, we selected only patients undergoing peripheral VA-ECMO and excluded patients who underwent recent cardiotomy or thoracotomy using VA-ECMO as the prolonged cardiopulmonary support. Patients without attempts of decannulation from VA-ECMO were not included in this study. The institutional review board approved the collection protocol and initially waived the requirement to obtain informed consent because of the difficulty obtaining consent from critically ill patients, the need for immediate implementation, and the lack of alternative therapies (201409041RIN).
Baseline characteristics and first-day conditions after VA-ECMO support, such as critical care score and extracorporeal cardiopulmonary resuscitation, were retrieved from the ECMO database. Logistic Organ Dysfunction System score for organ dysfunction in the intensive care unit and Survival After Veno-Arterial ECMO score were calculated. On the day of the first intent of decannulation, the fluid loading status as central venous pressure (CVP), the ECMO supporting flow, the use of intra-aortic balloon pump, ventilator, renal replacement therapy, and catecholamine dose by the inotrope equivalent method were also retrieved from the ECMO database. After the decision of decannulation in the morning visit, routine echocardiography and the additional three-dimensional (3D) echocardiographic data sets were acquired before decannulation.
According to our surgeons’ protocol, successful weaning was defined as free from mortality without reconnection 48 hours after removal of ECMO circuits. To analyze 30-day survival after the first intent of decannulation, we defined all-cause mortality as the event. Because the actual longevity of patients’ own hearts was unknown while accepting ventricular assist device or heart transplantation, these patients along with those who survived to discharge within 30 days after the first intent of decannulation were considered as censored. Hazard ratios of all-cause mortality were analyzed at 30 days after the first intent of decannulation. For repeatedly recorded parameters, including critical care score, CVP, dose of inotropic agents, and ECMO supporting flow, the values on the day of the first intent of decannulation were adopted for logistic or Cox regression analyses.
Procedure of VA-ECMO Implantation
To deal with refractory cardiogenic shock, our cardiovascular surgeons and ECMO technicians could provide emergent VA-ECMO implantation at bedside via peripheral vascular accesses (usually the femoral vessels) with previously reported equipment and management protocol.
Procedure of VA-ECMO Decannulation
Patients who met the criteria listed in the footnote to Table 1 were candidates for decannulation. After the decision for VA-ECMO decannulation, ECMO technicians conducted the evaluation protocol. The VA-ECMO flow rate was decreased to 0.5 L/min and be maintained for a 5-min observation period. If patients tolerated the observation, the cardiovascular surgeon clamped both arterial and venous cannulas. Activated clotting time was corrected to <180 sec; as a result, there was a 3-min observation period off VA-ECMO support. If the patient tolerated this period well, the arterial and venous cannulas were removed sequentially with repair of insertion site under direct vision. Any hemodynamic instability would stop the decannulation procedure.
Patient number | Gender (age in years) | Implantation of VA-ECMO | Total duration (h) | Intent of decannulation | ||
---|---|---|---|---|---|---|
Clinical scenario | Pump failure ∗ | Indications † | Result | |||
1 | Male (55) | AMI (OHCA, ECPR, no revascularization) | LV, RV | 51.4 | 1 | Success |
2 | Male (64) | AMI (OHCA, ECPR, successful primary PCI, patent LM) | LV, RV | 42.7 | 1, 2 | Success |
3 | Female (51) | AMI (IHCA, successful rescue PCI, patent LM) | LV | 95.7 | 2 | Success |
4 | Male (66) | AMI (OHCA, ECPR, successful primary PCI, stenotic LM) | LV | 114.3 | 2 | Success |
5 | Female (65) | AMI (IHCA, ECPR, failed primary PCI, stenotic LM) | LV | 238.2 | 1 | Success |
6 | Male (66) | AMI (IHCA, successful primary PCI, stenotic LM) | LV | 237.3 | 1, 2 | Success |
7 | Female (73) | AMI (IHCA, ECPR, failed primary PCI, patent LM) | LV | 73.8 | 1 | Success |
8 | Male (65) | AMI (IHCA, successful primary PCI, patent LM) | LV | 81.2 | 1, 2 | Success |
9 | Male (63) | AMI (IHCA, ECPR, failed primary PCI, patent LM) | LV, RV | 108.8 | 1 | Success |
10 | Male (60) | AMI (IHCA, ECPR, successful primary PCI, stenotic LM) | LV | 258 | 2 | Success |
11 | Male (61) | AMI (successful primary PCI, patent LM) | LV | 118.8 | 1 | Success |
12 | Male (77) | AMI (IHCA, ECPR, successful primary PCI, stenotic LM) | LV | 86.4 | 1, 2 | Success |
13 | Male (68) | AMI (successful primary PCI, patent LM) | LV | 191.7 | 1, 2 | Success |
14 | Male (79) | AMI (successful primary PCI, patent LM, with RV infarction) | RV | 62.8 | 1 | Success |
15 | Male (71) | AMI (successful primary PCI, stenotic LM) | LV | 96.5 | 1, 2 | Success |
16 | Male (63) | AMI (OHCA, ECPR, successful primary PCI, patent LM) | LV | 124.1 | 1 | Success |
17 | Female (81) | AMI (complicated PCI, occluded LM with rescue, IHCA, ECPR) | LV | 98.1 | 1 | Success |
18 | Male (68) | AMI (successful rescue PCI, stenotic LM) | LV, RV | 209 | 3 | Failure |
19 | Male (50) | AMI (IHCA, successful primary PCI, patent LM) | LV, RV | 330.3 | 2 | Failure |
20 | Male (43) | AMI (OHCA, ECPR, successful primary PCI, stenotic LM) | LV, RV | 113.5 | 1, 2 | Failure |
21 | Male (36) | AMI (IHCA, successful rescue PCI, patent LM) | LV | 28.4 | 1 | Failure |
22 | Male (63) | AMI (complicated PCI, occluded LM, IHCA, ECPR) | LV | 699.2 | 2 | Failure |
23 | Male (48) | AMI (IHCA, ECPR, failed primary PCI, stenotic LM) | LV, RV | 38.8 | 1, 2 | Failure |
24 | Male (29) | Dilated cardiomyopathy | LV, RV | 91.1 | 2 | Success |
25 | Female (65) | Dilated cardiomyopathy (IHCA) | LV, RV | 70.1 | 1, 2 | Success |
26 | Male (31) | Dilated cardiomyopathy | LV, RV | 315 | 3 | Failure |
27 | Male (39) | Dilated cardiomyopathy | LV, RV | 91.3 | 1, 2 | Failure |
28 | Male (55) | Dilated cardiomyopathy | LV, RV | 1494.3 | 1 | Failure |
29 | Male (19) | Dilated cardiomyopathy | LV, RV | 736.9 | 3 | Failure |
30 | Male (65) | Dilated cardiomyopathy | LV, RV | 136 | 3 | Failure |
31 | Male (59) | Ischemic cardiomyopathy | LV, RV | 122.1 | 1, 2 | Failure |
32 | Female (63) | VT/VF (IHCA, ECPR, digoxin overdose) | LV, RV | 121 | 1, 2 | Success |
33 | Male (22) | VT/VF (OHCA, Brugada syndrome) | LV, RV | 110 | 1, 2 | Success |
34 | Female (54) | VT/VF (IHCA, valvular heart disease, prostheses dysfunction) | LV, RV | 129.4 | 2 | Failure |
35 | Male (60) | VT/VF (IHCA, ECPR, old myocardial infarction) | LV, RV | 354.3 | 2 | Failure |
36 | Female (63) | VT/VF (IHCA, old myocardial infarction) | LV, RV | 205.3 | 1, 2 | Failure |
37 | Female (40) | Acute myocarditis (IHCA, ECPR) | LV, RV | 91.5 | 1, 2 | Success |
38 | Female (39) | Acute myocarditis | LV, RV | 119.2 | 2 | Failure |
39 | Male (20) | Acute myocarditis | LV, RV | 68.5 | 1 | Failure |
40 | Female (78) | Pulmonary embolism | RV | 52.3 | 1, 2 | Success |
41 | Male (48) | Pulmonary embolism | RV | 404 | 1, 2 | Failure |
42 | Male (30) | Thyrotoxicosis (IHCA, ECPR) | LV, RV | 669.7 | 1 | Success |
43 | Female (38) | Thyrotoxicosis (IHCA) | LV, RV | 79.4 | 1, 2 | Success |
44 | Female (63) | Idiopathic (unknown etiology after extensive workup, IHCA) ‡ | LV | 105.2 | 2 | Success |
45 | Male (64) | Idiopathic (possible sarcoidosis, IHCA, ECPR) § | LV, RV | 792.8 | 1 | Success |
46 | Male (38) | Acute rejection of previously transplanted heart | LV, RV | 136.9 | 2 | Success |
∗ Pump failure was assessed after initial return of spontaneous circulation.
† Candidates for decannulation had (1) CVP < 12 mm Hg, (2) inotropic equivalent < 10 μg/kg/min, or (3) the discretion of the principal cardiovascular surgeon, in addition to stable hemodynamic status (systolic blood pressure > 90 mm Hg, pulse pressure > 40 mm Hg) and adequate tissue perfusion (serum lactic acid < 4 mmol/L).
‡ A patient had been stabilized after 6-day VA-ECMO support without explainable etiology at later workup such as diagnostic angiography, laboratory tests, and even bone marrow biopsy.
§ A patient was a victim of sarcoidosis but with negative myocardial biopsy.
Transthoracic Echocardiography
The 3D echocardiographic data set was acquired using an iE33 system equipped with an X5-1 transducer (Philips Medical Systems, Andover, MA) and stored digitally for offline analysis. Because all patients were supported by peripheral VA-ECMO, their acquisition windows were relatively satisfactory for the transthoracic approach. When the left decubitus position was mandatory for better acquisition, ECMO technicians stood by for safe examination. When the left or right ventricle was too dilated to be included together in a single 3D pyramidal data set, we performed RV 3DE by the medial or lateral approach as previously suggested ( Figure 1 A and 1B). The orthogonal biplane display before acquiring a full-volume data set was adopted to prevent truncation of the apex. Angles and depths of scanning were optimized to achieve a high frame rate with four-beat acquisition mode in patients with regular rhythm. Sometimes the respiratory motion of the heart led to stitching artifacts, and the ventilator settings of respiratory rate, tidal volume, and VA-ECMO gas flow were adjusted to diminish such artifacts while maintaining adequate gas exchange. For patients with frequent premature ventricular or atrial complexes, the 3D echocardiographic acquisition mode at high volume rate was adopted at the slight expense of spatial resolution. Valvular regurgitation was qualitatively assessed by color flow jet area (Nyquist limit 50–70 cm/sec) and flow convergence zones.

Analysis of 3DE
These 3D echocardiographic data sets were imported to a software platform (Image-Arena version 4.6; TomTec Imaging Systems, Unterschleissheim, Germany). The investigators, blinded to the results of weaning from VA-ECMO, performed the volumetric analyses with the module for the right ventricle (4D RV-Function 2.0) and the left ventricle (4D LV-Analysis 3.1) according to the operating instructions. Briefly, after deciding anatomic axes and landmarks on software-defined sections at end-diastole ( Figure 1 C), the endocardial border was automatically detected and improved by interactive manual adjustment ( Figure 1 D). The coronal section of the right ventricle was optimized to delineate the RV outflow tract by adjusting the degrees of the cutting plane with the center of the mitral valve. The surface model of the contemplated ventricle was then dynamically tracked throughout the cardiac cycle to compute end-diastolic volume, end-systolic volume, and ejection fraction. We also calculated global shortening in longitude as global longitudinal strain (GLS) and global shortening in latitudes as global circumferential strain from the LV surface model. On the basis of the 3DE-derived apical four-chamber view with maximum RV dimension, the longitudinal strain of the RV septum and free wall were also measured.
Analysis of Intra- and Interobserver Variability
The semiautomatic quantifications of ventricular volume and deformation were repeated in a randomly selected subgroup of 20 patients for analysis of reproducibility. This included repeated measurements by the same observer >1 month apart, as well as measurements by a second observer, both blinded to all prior measurements. Interobserver and intraobserver variability was calculated as an absolute difference between the corresponding pair of repeated measurements, shown as the mean ± SD. The coefficient of variation and intraclass correlation coefficient were also calculated to appraise reproducibility. According to a prior investigation of the right ventricle using 3DE, a coefficient of variation < 10% was considered to indicate good consistency.
Statistical Methods
Categorical variables were compared using the χ 2 test or Fisher exact test. Continuous variables are expressed as mean ± SD. On the basis of their distributions, continuous variables were compared using Student’s t test or the Mann-Whitney U test. Receiver operating characteristic (ROC) curves were constructed to determine the optimal cutoff of chosen variables and the area under the curve (AUC). The differences in AUC between ROC curves were analyzed using the DeLong test. Net reclassification improvement (NRI) was calculated to define an incremental value of one parameter over another. Spearman’s rank correlation method was used as a nonparametric measure of association between RVEF and clinical and echocardiographic parameters. Those parameters that reached statistical significance ( P < .05) were entered in a stepwise model to identify independent determinants of RVEF. Cox proportional hazard models were used to evaluate the relationship between RVEF and survival. Kaplan-Meier curves and the log-rank tests were used to test differences in survival probability between patients stratified by RVEF. The statistical analyses were performed using SPSS Statistics for Windows version 17.0 (IBM SPSS, Chicago, IL). All reported P values were two tailed, and P values < .05 were considered to indicate statistical significance.
Results
Study Population
In this study period, 147 adult patients underwent VA-ECMO support for acute cardiogenic shock. Forty-one patients had VA-ECMO after cardiotomy or thoracotomy. Twenty-seven patients had ECMO duration <24 hours (abrupt recovery or mortality), and thus 3DE was not performed. Twenty-three patients had no attempt at decannulation. Ten patients had poor acoustic windows. As a result, only 46 patients ( Table 1 ) and their 3D echocardiographic data sets before the first intent of decannulation were selected. Twenty-eight patients (60.9%) were successfully weaned. Of the remaining 18 patients who failed the weaning process, 10 died on VA-ECMO, two died within 48 hours off VA-ECMO, one died on a biventricular assist device, one was bridged to an LV assist device, and four underwent heart transplantation. Among the 28 patients who were successfully weaned, 22 survived at discharge, two died of relapse of cardiogenic shock, and four died of fatal complications of the central nervous system. Total duration of VA-ECMO supporting was 200 ± 208 hours in the success group and 242 ± 348 hours for those failed to wean ( P = .61).
Clinical Characteristics and Echocardiographic Parameters
Clinical characteristics on the day of VA-ECMO implantation (day 1) and the day of 3DE are summarized in Table 2 and Table 3 , respectively. On day 1, there was no significant difference in critical care scores and hemodynamic conditions between the weaning successes and failures. On the day of first intent of decannulation, the success group had lower Logistic Organ Dysfunction System scores and ECMO supporting flow. All RV 3D echocardiographic data sets were of adequate frame rates (20 ± 1.5 Hz). Regarding echocardiographic parameters, the success group had smaller right and left ventricles, better RVEF ( P < .001), and higher RV fractional area change (FAC; P = .011), higher magnitudes of RV strain (free wall, P = .001; septal, P = .004), better LVEF ( P = .045), and higher magnitudes of LV global circumferential strain ( P = .038). The differences in LV GLS, tricuspid annular plane systolic excursion, and the severity of atrioventricular valvular regurgitation did not reach significance.
Population ( N = 46) | Success ( n = 28) | Failure ( n = 18) | P | |
---|---|---|---|---|
Population characteristics | ||||
Male | 33 (72) | 18 (64) | 15 (83) | .148 |
Age (y) | 54.7 ± 16.3 | 59.2 ± 15.8 | 47.7 ± 14.8 | .018 |
Diabetes mellitus | 18 (39) | 14 (50) | 4 (22) | .053 |
Hypertension | 18 (39) | 13 (46) | 5 (28) | .206 |
Coronary artery disease | 14 (30) | 9 (32) | 5 (28) | .760 |
Chronic lung disease | 2 (4) | 2 (7) | 0 (0) | .161 |
Chronic kidney disease | 6 (13) | 1 (4) | 5 (18) | .047 |
Prior stroke | 2 (4) | 1 (4) | 1 (5) | ≥.999 |
Critical care score | ||||
LODS score (day 1) | 7.4 ± 3.3 | 6.9 ± 3.9 | 8.5 ± 3.6 | .162 |
SAVE score | −6.2 ± 6.1 | −7.2 ± 1.0 | −4.7 ± 1.7 | .173 |
Hemodynamic condition | ||||
ECPR | 18 (39) | 14 (50) | 4 (22) | .053 |
CVP (cm H 2 O) (day 1) | 10.4 ± 3.9 | 10.5 ± 4.0 | 10.4 ± 3.8 | .932 |
IE (μg/kg/min) (day 1) | 11.3 ± 14.5 | 8.0 ± 6.7 | 16.3 ± 21.0 | .122 |
ECMO flow (L/min) (day 1) | 2.6 ± 0.9 | 2.7 ± 0.8 | 2.6 ± 0.9 | .865 |
Population ( N = 46) | Success ( n = 28) | Failure ( n = 18) | P | |
---|---|---|---|---|
Critical care score | ||||
LODS | 7.7 ± 3.0 | 6.8 ± 2.4 | 9.2 ± 3.2 | .006 |
Hemodynamic condition | ||||
CVP (cm H 2 O) | 11.8 ± 5.6 | 10.7 ± 4.5 | 13.5 ± 6.7 | .131 |
HR (beats/min) | 97.9 ± 20.5 | 93.3 ± 17.3 | 105.1 ± 23.2 | .056 |
IE (μg/kg/min) | 10.3 ± 10.9 | 7.9 ± 10.3 | 14.0 ± 11.2 | .068 |
ECMO flow (L/min) | 2.1 ± 0.6 | 1.9 ± 0.7 | 2.4 ± 0.6 | .018 |
IABP support | 17 (36) | 12 (43) | 5 (28) | .373 |
Mechanical support | ||||
Ventilator | 41 (89) | 26 (93) | 15 (83) | .342 |
Renal replacement therapy | 24 (46) | 13 (46) | 11 (61) | .364 |
Echocardiography, right ventricle | ||||
RV EDV (mL) | 69.0 ± 36.6 | 59.7 ± 34.3 | 83.6 ± 36.2 | .033 |
RV EDVi (mL/m 2 ) | 40.1 ± 23.1 | 34.6 ± 20.5 | 48.5 ± 24.9 | .044 |
RV ESV (mL) | 49.9 ± 30.0 | 38.9 ± 24.7 | 67.0 ± 30.3 | .002 |
RV ESVi (mL/m 2 ) | 29.0 ± 19.2 | 22.6 ± 14.7 | 39.0 ± 21.3 | .003 |
RVEF (%) | 29.8 ± 11.9 | 36.2 ± 9.7 | 19.8 ± 7.1 | <.001 |
RV FAC (%) | 26.2 ± 11.7 | 29.6 ± 11.9 | 20.8 ± 9.6 | .011 |
TAPSE | 8.0 ± 3.4 | 8.7 ± 4.0 | 6.9 ± 2.1 | .052 |
RV septal strain (%) | −9.5 ± 4.7 | −10.9 ± 4.7 | −7.2 ± 3.7 | .004 |
RV free wall strain (%) | −11.33 ± 6.5 | −13.7 ± 6.9 | −7.6 ± 3.8 | .001 |
TR moderate or greater | 10 (22) | 4 (14) | 6 (33) | .162 |
Echocardiography, left ventricle | ||||
LV EDV (mL) | 101.2 ± 59.6 | 81.4 ± 34.1 | 133.3 ± 77.6 | .004 |
LV EDVi (mL/m 2 ) | 60.1 ± 40.2 | 47.5 ± 20.9 | 80.0 ± 54.3 | .008 |
LV ESV (mL) | 73.2 ± 54.0 | 54.7 ± 27.1 | 106.9 ± 70.6 | .001 |
LV ESVi (mL/m 2 ) | 44.7 ± 36.8 | 32.0 ± 16.5 | 64.7 ± 50.4 | .003 |
LV SV (mL) | 26.6 ± 12.0 | 26.7 ± 10.8 | 26.4 ± 14.1 | .947 |
LV SVi (mL/m 2 ) | 15.4 ± 7.2 | 15.5 ± 6.5 | 15.3 ± 8.4 | .931 |
LVEF (%) | 31.2 ± 14.5 | 35.0 ± 12.3 | 25.6 ± 16.3 | .045 |
LV GLS (%) | −6.5 ± 4.5 | −7.6 ± 4.2 | −5.1 ± 4.3 | .098 |
LV GCS (%) | −12.6 ± 6.2 | −14.0 ± 5.5 | −9.8 ± 6.8 | .038 |
MR moderate or greater | 13 (28) | 6 (21) | 7 (39) | .229 |
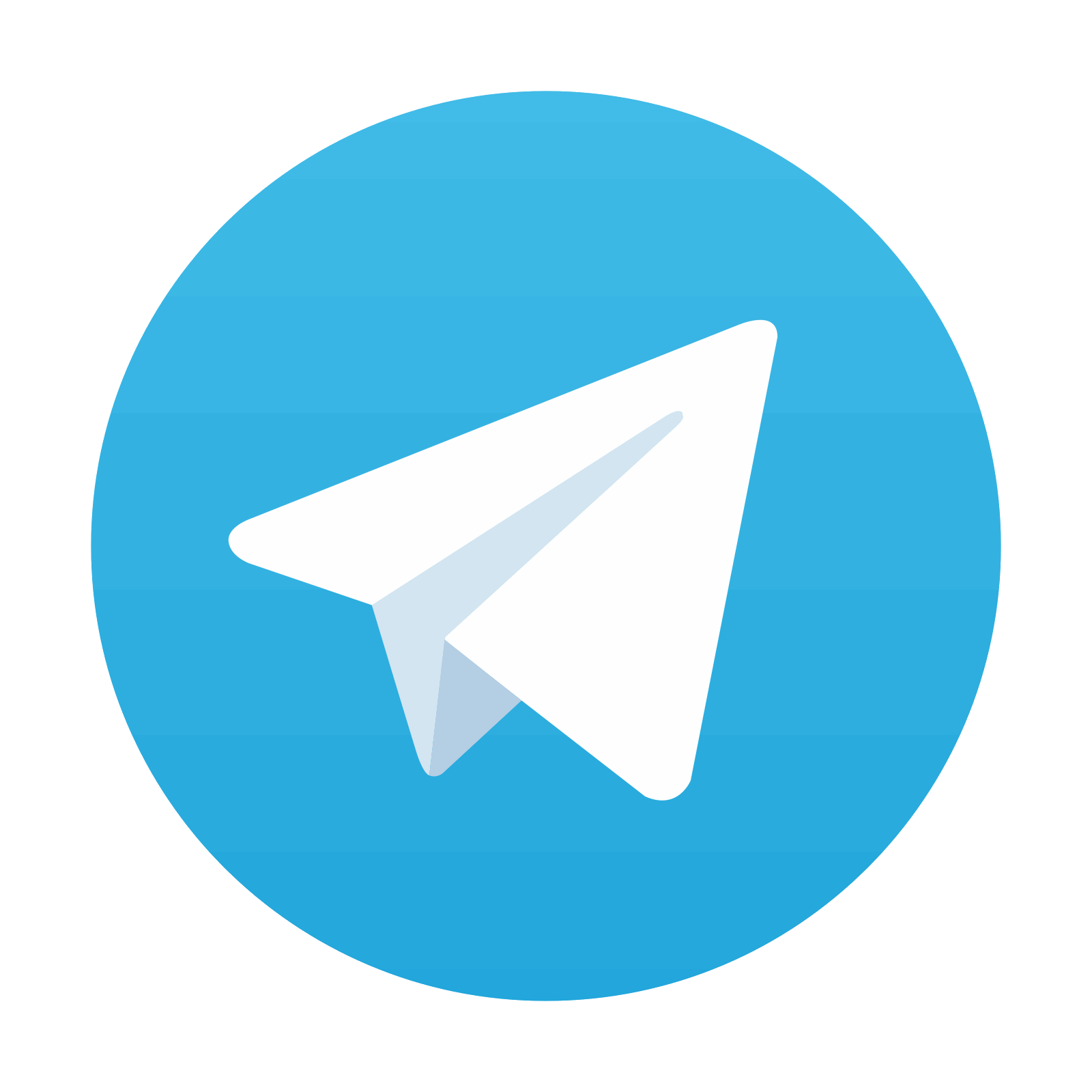
Stay updated, free articles. Join our Telegram channel

Full access? Get Clinical Tree
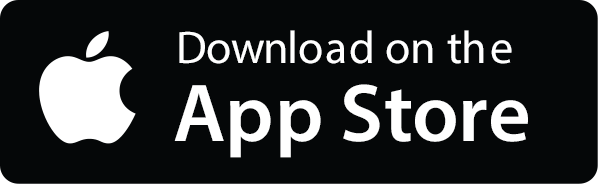
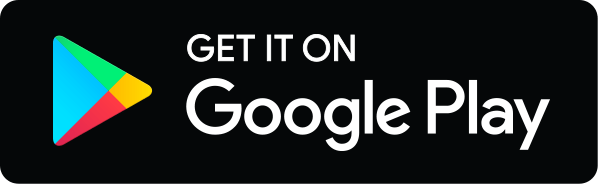
