Background
The aim of this study was to evaluate the accuracy of three-dimensional (3D) ultrasound compared with the standard magnetic resonance imaging method in determining right ventricular (RV) volumes and function in adult patients with congenital heart disease and chronic, severe pulmonary regurgitation (PR).
Methods
Twenty-five patients with severe PR secondary to either pulmonary valvotomy or tetralogy of Fallot repair were evaluated using 3D ultrasound and MRI.
Results
The mean RV ejection fractions were 42 ± 8% on 3D ultrasound and 44 ± 7% on MRI ( r = 0.89, P < .0001). The mean end-diastolic volumes were 249 ± 66 and 274 ± 82 mL and the mean end-systolic volumes 147 ± 50 and 159 ± 60 mL on 3D ultrasound and MRI, respectively. Similarly, there were strong correlations of both end-diastolic volume and end-systolic volume on 3D ultrasound and MRI ( r = 0.88 and r = 0.89, respectively).
Conclusions
Three-dimensional ultrasound was comparable with MRI in determining RV size and function in most patients with complex congenital heart disease. It will be important to study 3D US in a larger population of patients with TOF, which will be possible only through multi-center collaboration.
The accurate evaluation of right ventricular (RV) volume and function remains challenging using two-dimensional (2D) echocardiography because of the complex crescent shape, large infundibulum, and prominent trabeculations of the right ventricle. Recently described measurements of tricuspid annular motion and myocardial performance index have improved 2D RV functional assessment. Despite these advancements, 2D echocardiography still provides only cross-sectional views of the heart, making direct volume and ejection fraction (EF) measurements unreliable. Although cardiac magnetic resonance imaging (MRI) is considered the best standard method for the evaluation of RV size and function, issues related to cost, claustrophobia, or the presence of pacemakers or defibrillators can make MRI prohibitive. Clinical studies to date have shown good agreement between cardiac three-dimensional (3D) ultrasound and MRI measurements of RV volume and function in the general normal adult population, providing an alternative to MRI. RV assessment is an important consideration but also most challenging in adult patients with congenital heart disease. Adults with chronic severe pulmonary regurgitation (PR) secondary to previous pulmonary valvotomy or previous intracardiac repair of tetralogy of Fallot (TOF) require regular RV assessment because they are at risk for progressive RV dilation and dysfunction, arrhythmias, and sudden cardiac death if the pulmonary valve is not replaced in a timely fashion.
Literature addressing the feasibility and accuracy of 3D echocardiographic RV assessment in adults with complex congenital heart disease is sparse, with a few studies confirming its utility in the pediatric population with congenital heart disease. Our study was designed to evaluate the feasibility and accuracy of a new 3D RV-specific analysis program in relation to the standard MRI method in determining RV volumes, stroke volumes (SVs), and EFs in adult patients with severe PR secondary to either previous pulmonary valvotomy or TOF repair.
Methods
Study Population
The Mayo Clinic Institutional Review Board approved the research protocol, and all patients were enrolled after providing written informed consent. Twenty-nine consecutive patients with chronic severe PR secondary to previous pulmonary valvotomy or intracardiac repair of TOF referred for clinically indicated MRI for the determination of RV size and function were asked to participate in this study. Both 2D and 3D ultrasound images were obtained within 24 hours of MRI. No participant was excluded on the basis of 2D ultrasound image quality, but 4 patients were excluded on the basis of 3D image quality that prohibited RV analysis. The remaining 25 patients were included in this study.
Echocardiography and MRI
All ultrasound images were acquired using a Philips iE33 ultrasound system (Philips Medical Systems, Andover, MA). Complete 2D echocardiography was performed, with the emphasis on the degrees of RV dilation and dysfunction. Global RV function was also assessed using Doppler parameters used to derive the Tei index, as previously described. Tissue Doppler measurements were also performed, guided by the 4-chamber view; a region of interest was placed at the basal/annular portion of the RV free wall. In this way, the peak RV systolic tissue velocity (S′) was obtained. Continuous-wave Doppler from all standard views recorded the maximal tricuspid regurgitation velocity; the view that yielded the highest peak velocity during end-expiration was used to estimate pulmonary artery pressure. A full matrix-array 3D transducer was used to acquire the 3D RV volume data set. The pyramidal volume data set was obtained from the apical window over 4 cardiac cycles during held end-expiration. Care was taken to include the entire RV cavity within the pyramidal scan volume. The full-volume 3D RV ultrasound data sets were digitally stored on CD-ROMs and transferred to a TomTec workstation with 4-dimensional (4D) analysis software (TomTec Imaging Systems, Munich, Germany) for offline analysis. Each volume data set was imported into the software program, and all images were viewed in 3 planes: 4-chamber (to visualize the apex), sagittal (to visualize the tricuspid valve), and coronal (to visualize the RV outflow tract) views. The images were then manipulated by slicing, rotating, and angulating in any of these 3 displayed orthogonal planes to optimize images for RV volume analysis. Postprocessing tools allowed for changes in contrast and shading to better delineate the endocardial borders. The end-diastolic and end-systolic frames were chosen by inspection of the cine loops in all 3 views. The end-diastolic frame marked near the onset of the QRS complex corresponding to the largest volume, and the end-systolic frame marked at the middle or end of T wave corresponding to the smallest volume. Tracings were performed in the 4-chamber, sagittal, and coronal planes. On the basis of the tracing in each view, the software reconstructed traced points in the other two views ( Figure 1 ). In the 4-chamber view, the tracing was performed from the lateral aspect of the tricuspid annulus to the apex and then up to the medial aspect of the annulus. In the coronal view, the tracing was performed from the free edge of the tricuspid annulus, down the free wall and up the RV outflow tract to the level of the pulmonary valve. In the same coronal view, a separate tracing was performed to delineate the infundibulum from the tricuspid annulus to the RV outflow tract. The endocardial border of the RV was traced at end-diastole and end-systole in all 3 orthogonal planes, with trabeculations included in the endocardial trace. Ultimately, endocardial traces in each slice extended from the annulus to the ventricular outflow tract. The software program also used a semiautomated border detection algorithm based on in vivo normal and pathologic RV models. The 3D images were then constructed with the opportunity for manual correction of borders in all frames of the cycle. Manual correction was required for all study patient images. We included RV trabeculations and papillary muscles in the tracing to incorporate them in the blood volume. The RV volumes were calculated by the sum of the area for each slice of the complete volume data set. In this way, the RV end-diastolic volume (EDV) and RV end-systolic volume (ESV), SV, and EF could be assessed. The software for 3D RV analysis created a surface geometry of the right ventricle by combining information embedded in the 3D volume set and the traces in the orthogonal planes ( Video 1 ; view video clip online). Image quality by 3D ultrasound was graded by the reader on the basis of the ability (1) to clearly visualize all 3 areas (tricuspid valve, apex, and ventricular outflow tract) and (2) to delineate the endocardial border.

Electrocardiographically gated cardiac MRI was performed with a 1.5-T system (Twin Speed EXCITE, GE Healthcare, Waukesha, WI). Following the identification of the long axis of the heart, contiguous short-axis cine steady-state free precession images were obtained during repeated end-inspiratory breath holds from the RV apex to above the atrioventricular ring using an 8-mm slice thickness with a 1-mm slice gap. Sequence parameters were as follows: echo time, 1.7 ms; repetition time, 3.4 ms; flip angle, 45°; matrix size, 256 × 192 mm; and phase field of view, 0.75 to 1.0. Several long-axis views of the right ventricle were also obtained to aid in the functional separation of the right atrium, right ventricle, and pulmonary artery. The endocardial contours of the right ventricle were traced manually on all short-axis slices and phases with commercially available software (MASS Analysis 6+; Medis Medical Imaging Systems, Leiden, Netherlands). Care was taken to include the tricuspid inlet and the RV outflow tract up to the pulmonary valve, and on the most basal slice, the right atrium and pulmonary artery were avoided. For the axial orientation, the same pulse sequence was used. Coronal localizing images were used to obtain orthogonal images in an axial orientation to cover the heart from the diaphragm to the pulmonary artery bifurcation using an 8-mm slice thickness with a 1-mm slice gap. The endocardial contours of the right ventricle were traced manually on all axial slices and phases in a similar fashion to the short-axis images. The uppermost slice was defined as the first slice at which a pulmonary valve cusp could be identified, and the area of the pulmonary cusp was excluded from the volumes. The most apical slice was defined as the last slice to contain blood volume. For both short-axis and axial images, end-diastole and end-systole were defined as the phases with highest and lowest volumes, respectively. RV EDV and ESV and RV EF were then automatically calculated by adding the volume of each individual slice. Separate readers analyzed all 3D ultrasound and MRI data independently.
Statistical Analysis
The means and standard deviations and the numbers and percentages were calculated for continuous and categorical variables, respectively. Correlations of RV EDV, ESV, SV, and EF obtained by 3D ultrasound and MRI were calculated by means of linear regression analysis. Agreement between the MRI and ultrasound measurements was further assessed using the Bland-Altman method. Intraobserver and interobserver agreement of a single 3D ultrasound data set derived RV EDV and EF was assessed by calculating the test-retest variability according to the following formula:
∑ 1 n ( x 1 − x 2 ) 2 / n 1 2 ( ∑ 1 n x 1 / n + ∑ 1 n x 2 / n ) ,
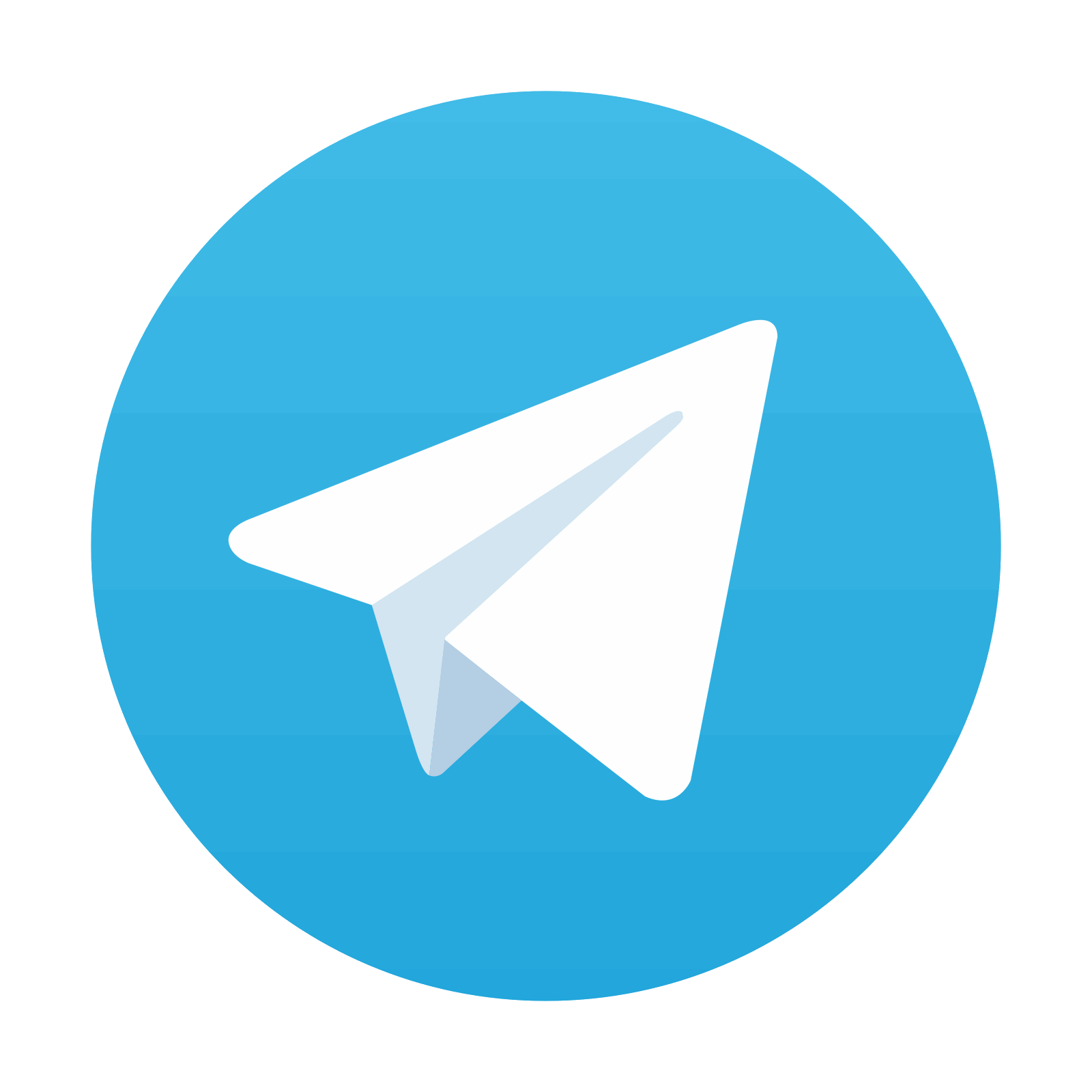
Stay updated, free articles. Join our Telegram channel

Full access? Get Clinical Tree
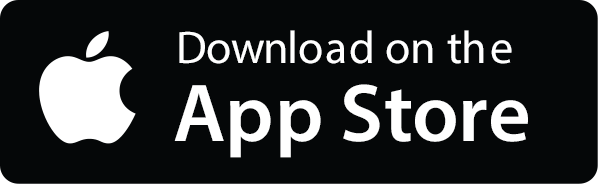
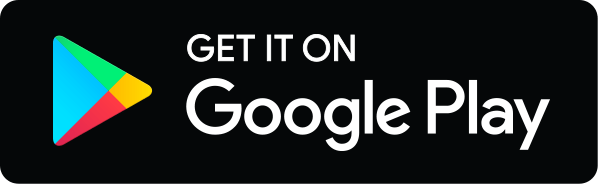