As the population becomes more obese and the prevalence of diabetes and metabolic syndrome increases, low-density lipoprotein cholesterol (LDL-c) is increasingly losing its value as a predictor for cardiovascular (CV) risk. In addition, targeting LDL-c treatment to less than 70 mg/dL has achieved significant cardiovascular disease (CVD) benefits, but a high residual risk continues, especially if other lipid parameters, such as triglyceride (TG) and high-density lipoprotein cholesterol (HDL-c) levels remain abnormal. Identifiable residual risk factors include low HDL-c and elevated TGs, LDL particle number, apolipoprotein (Apo) B, high-sensitivity C-reactive protein (hs-CRP), and lipoprotein-associated phospholipase A2 (Lp-PLA2). Although additional therapeutic intervention beyond statin therapy to modify these residual risk factors has not yet been proven to reduce CV events, ample evidence suggests that patients with these abnormalities remain at increased risk despite having LDL-c and non–HDL-c at goal (<70 mg/dL and <100 mg/dL, respectively), according to the National Cholesterol Education Program Adult Treatment Panel III (NCEP ATP III) 2004 update. Statin combination therapy with niacin, fibrates, and prescription omega-3 fatty acids provides additional improvements in non–HDL-c and HDL-c. Clinical trials are underway to evaluate the effects of niacin in combination with a statin on CV outcomes. Cholesteryl ester transfer protein (CETP) inhibitors, which increase HDL-c, are also in late-phase development, with the potential to address the residual risk for patients with low HDL.
Rationale for Combination Therapy
Lipid treatment options to reduce coronary heart disease (CHD) risk have focused on lifestyle changes and LDL-c–lowering therapy, most commonly with statins, as recommended by the ATP III. However, studies show that a significant proportion of patients who receive statin therapy do not achieve all optimal lipid levels, particularly those patients with mixed dyslipidemia. Substantial residual risk of CV events may exist in patients with abnormal TGs and HDL-c after statin treatment has been initiated. Specifically, the clinical benefit of having a TG level below 150 mg/dL on statin treatment has been demonstrated in an acute coronary syndrome (ACS) population. The Pravastatin or Atorvastatin Evaluation and Infection Therapy–Thrombolysis in Myocardial Infarction 22 (PROVE-IT–TIMI 22) trial observed a significant 1.6% reduction in the incidence of death, myocardial infarction (MI), and recurrent ACS for each 10 mg/dL (0.113 mol/L) decrement in TG levels after adjusting for LDL-c. Statin trials demonstrate that for patients with elevated TGs or low HDL-c, risk for a future CHD event is increased by 40% to 70% ( Figures 25-1 and 25-2 ). Currently available statins and other lipid-altering drugs used as monotherapy often do not reach optimal levels in all lipid parameters; therefore, the combination of statins and other lipid-altering drugs that target TG and HDL-c levels can potentially fill this unmet need in patients with mixed dyslipidemia.


Lack of Achievement of Non–High-Density Lipoprotein Goals
Many patients with CHD have therapeutic needs that exceed the simple reductions in LDL-c obtained by using statins. The ATP III has acknowledged the increasing evidence of an independent association between hypertriglyceridemia and CHD by issuing guidelines that identify non–HDL-c as a secondary target for therapy in patients with elevated TG levels. This association is likely related to the atherogenicity of some species of TG-rich lipoproteins, particularly small very-low-density lipoprotein (VLDL) and intermediate-density lipoprotein particles. Elevation of these “remnant” lipoproteins has been shown to contribute to atherosclerosis in mice. Evidence exists that genetic dyslipidemias characterized by increased concentrations of remnant lipoproteins are associated with the development of premature CHD, and remnant lipoprotein levels strongly predict the progression of atherosclerosis. In addition, in both observational studies and during treatment in clinical trials, levels of ApoB, reflecting the total number of circulating atherogenic particles (LDL plus VLDL), are better associated with CHD risk than with LDL-c concentration.
Residual Risk for Statin Therapy
With the recognition that additional event reduction can be obtained by more intensive LDL-c reductions in some patients who are at very high risk for CHD events, the ATP III recommended the therapeutic optional goal for LDL-c less than 70 mg/dL and non–HDL-c less than 100 mg/dL. Cholesterol-lowering therapy with statins has been established as an effective method for reducing death and MI among patients with CHD. However, a significant number of individuals who receive statin therapy continue to have high residual risk. An important clinical challenge exists to reduce residual CHD risk with optimal therapies without increasing adverse effects. Combination therapy appears most appropriate for patients with a high rate of events on optimal statin therapy. In addition to lifestyle modification, the use of combination therapy in CHD is an acknowledged strategy in optimal management to prevent or delay the morbidity and mortality associated with CHD and its risk factors. Current recommendations for CHD prevention and treatment advise the use of combination drug therapy for high-risk patients, including those with combined hyperlipidemia and diabetic dyslipidemia.
In light of the residual risk of CHD events in statin trials within certain subgroups, combination therapy appears most appropriate in patients with a high rate of events despite optimal statin therapy. The updated ATP III guidelines recommend an optional LDL-c goal of less than 70 mg/dL in patients at very high risk, including those with established CVD in conjunction with multiple major risk factors, severe or poorly controlled risk factors, multiple metabolic syndrome components, or ACS. In a national survey of compliance with the ATP III guidelines, 75% of patients with CHD met the definition of “very high risk,” yet only 18% had an LDL-c level below 70 mg/dL, and only 4% had an LDL-c level below 70 mg/dL and a non–HDL-c level below 100 mg/dL when TGs were above 200 mg/dL. These data substantiate the use of more aggressive statin therapy and implementation of combination therapy to reduce residual risks with statins.
Combined Dyslipidemia
Non–High-Density Lipoprotein Cholesterol Reduction
The ATP III identified non–HDL-c as a secondary therapeutic target for individuals with elevated TGs of 200 mg/dL or more. Non–HDL-c goals are set 30 mg/dL above the LDL-c goal for a patient’s risk category ( Table 25-1 ), a level based on the Friedewald formula, in which VLDL cholesterol (VLDL-c) levels are estimated to be one fifth of the TG concentration. Because TG concentrations below 150 mg/dL are considered normal, a VLDL-c concentration below 30 mg/dL is within the normal range. As TG levels increase, the fraction of non–HDL-c accounted for by LDL-c declines.
OMEGA-3 FATTY ACIDS | FENOFIBRATE/FENOFIBRIC ACID | NIACIN | |
---|---|---|---|
LDL-c | ↑ | — | ↓ |
Non-HDL | ↓ | ↓ | ↓ |
Triglycerides | ↓↓ | ↓↓ | ↓ |
ApoB | ↓ | ↓ | ↓↓ |
ApoA1 | — | ↑ | ↑↑ |
LDL-P | ↓ | — | ↓ |
LDL size | ↑ | ↑ | ↑ |
VLDL-P | ↓ | ↓ | ↓ |
VLDL size | ↓ | ↑↓ | NA |
HDL-P | — | ↑ | ↑ |
HDL size | — | ↑ | |
Lp-PLA2 | ↓ | NA | ↑A |
hs-CRP | — | ↓ | NA |
Homocysteine | — | ↑↑ | ↑ |
RLP-C | ↓ | ↓ | ↓ |
ApoCIII | ↓ | ↓ | ↓ |
Non–HDL-c correlates well with ApoB concentration and is therefore a good proxy for the total number of circulating atherogenic particles. Consistent with this relationship, population studies have shown non–HDL-c to be a somewhat stronger predictor of CVD and mortality risk than LDL-c.
Therapy to Modify High-Density Lipoprotein
The success of LDL-c reduction to improve cardiovascular outcomes is now well recognized. Heart failure hospitalizations have dropped 29.5% nationally over the past decade, largely because fewer MIs resulted in a decrease in the development of heart failure. Although the reasons for this decline are many, better risk factor modification, especially LDL-c control with statins, is believed to be a major contributor.
Yet, despite optimal LDL-c management, patients with low HDL-c remain at increased risk for cardiovascular events. Low HDL-c is a powerful risk factor, equivalent to elevated LDL-c for premature CHD, and high HDL-c provides protection from cardiovascular mortality. Therefore, logic presumes that therapeutic approaches to raise HDL-c should reduce events.
To date, however, efforts to increase HDL-c by pharmacologic means have not shown a clinical benefit. Estrogen, torcetrapib, and most recently niacin raised HDL-c in trials; not only did these agents fail to provide a clinical benefit, they may have caused harm. Although off-target effects may have been responsible, profound confusion remains as to why raising HDL-c has not achieved the expected benefits.
The HDL hypothesis , as yet unproven, suggests that the benefits of modifying HDL are mediated through several atheroprotective mechanisms. Most importantly, HDL removes excess cholesterol from peripheral tissues and delivers it to the liver for elimination via the bile into the gut, a process known as reverse cholesterol transport (RCT). Because free cholesterol cannot be metabolized in humans on its own, this RCT process represents an important mechanism for regulating cholesterol pools and maintaining cholesterol homeostasis.
The key role that HDL plays in mediating the RCT process is regarded as the primary mechanism by which HDL affects atherosclerosis. HDL functionality may be more important than absolute HDL-c levels, and recent data indicate that HDL has both proinflammatory and antiinflammatory properties. However, no large trials have yet confirmed this hypothesis. The view that raising HDL-c will result in a CV benefit is likely overly simplistic. On the horizon are new CETP-modulating drugs that do not share the off-target toxicity of torcetrapib. The dal-VESSEL and dal-PLAQUE trials of dalcetrapib recently demonstrated its vascular safety. In the Determining the Efficacy and Tolerability of CETP Inhibition With Anacetrapib (DEFINE) trial, the cardiovascular toxicity associated with torcetrapib was ruled out with anacetrapib. Large outcomes trials are now underway to evaluate the clinical benefits of these drugs in populations with high CV risk. Despite disappointment and confusion, the quest to establish the value of modifying HDL continues, and it is hoped this promising target of therapy will yield the results that address a very important unmet medical need.
Statin-Fibrate Combination
Fibrates are an important class of drugs for the management of dyslipidemia. This class of drugs is generally well tolerated but is infrequently associated with several safety issues. By activating peroxisome proliferator activated receptor α (PPARα), fibrates result in the regulation of several genes important in lipid metabolism. These include increasing the expression of lipoprotein lipase and reducing the production of ApoC-III, an inhibitor of lipoprotein lipase activity, both of which enhance the lipolysis and elimination of TG-rich particles. In addition, increases in the production of apolipoprotein AI (ApoA-I) and ApoA-II contribute to an increase in HDL-c. Fibrates may reversibly increase creatinine and homocysteine but are not associated with an increased risk for renal failure in clinical trials. In patients with combined dyslipidemia, fibrate-statin combination therapy can be used to promote reductions in LDL and TGs and simultaneous increases in HDL-c.
Fibrates are associated with a slightly increased risk (<1.0%) for myopathy, cholelithiasis, and venous thrombosis. In clinical trials with patients who did not have elevated TGs and/or low HDL levels, fibrates are associated with an increase in non-CV mortality. In combination with statins, gemfibrozil generally should be avoided. The preferred option is fenofibrate, which is not associated with an inhibition of statin metabolism. Clinicians are advised to measure serum creatinine before fibrate use and to adjust the dose accordingly for renal impairment. Routine monitoring of creatinine is not required, but if a patient has a clinically important increase in creatinine, and other potential causes of creatinine increase have been excluded, consideration should be given to discontinuing fibrate therapy or reducing the dose. The active metabolite of fenofibrate, fenofibric acid, has been approved for clinical use in combination with a statin.
A total of 2715 patients were randomly assigned to 12-week treatment with either fenofibric acid 135 mg monotherapy; low-, moderate-, or high-dose statin (rosuvastatin, simvastatin, or atorvastatin) monotherapy; or fenofibric acid plus a low- or moderate-dose statin. Fenofibric acid plus low-dose statin combination therapy resulted in significantly greater improvements in HDL-c, TG, non–HDL-c, VLDL-c, total cholesterol, ApoB, and hs-CRP compared with low-dose statin monotherapy. The safety of combination fenofibric acid 135 mg with a statin was similar to monotherapy for each drug, and no cases of rhabdomyolysis were reported. Outcome studies are underway to evaluate whether the beneficial effects of a combination of fenofibrate and statins on lipoproteins translates into a reduction in the residual risk for patients on statin monotherapy with elevated TGs and low HDL-c.
The Action to Control Cardiovascular Risk in Diabetes (ACCORD) trial is the most recent study to compare the combination of a statin (simvastatin) and fenofibrate with a statin alone in an all-comer diabetes population. Although designed primarily to compare the effects of more intensive and less intensive glucose control, the study also compared intensive and standard blood pressure control in 4733 of the patients (n = 10,251), and it investigated the impact of adding fenofibrate to a statin on the lipid profile and CV outcomes in the remaining 5518 patients.
In the lipid arm of ACCORD, 2765 patients were randomized to receive simvastatin plus fenofibrate, and 2753 patients were to receive simvastatin plus placebo. The impact on the lipid profile was as predicted, with a slight decrease in mean total cholesterol, no change in mean LDL-c, a modest increase in HDL-c (modest increases in HDL-c are seen in studies in which patients have high levels of TGs), and a significant decrease in TGs from about 160 mg/dL to 120 mg/dL in the fenofibrate group.
In terms of the primary macrovascular outcome—CV death, nonfatal MI, and nonfatal stroke—no difference was reported between the groups in the proportion of patients with events, the time to event, or the total events between the two arms of the trial. In addition, no difference was noted between the two groups in terms of major prespecified secondary macrovascular outcomes, including major CV events, nonfatal MI, stroke, and death from any cause.
As expected from previous trials with fibrates, a potential but not statistically positive benefit was observed in patients with high levels of TGs and low levels of HDL (which represented just 20% of the study population), with a 31% risk reduction in this patient population ( P = .064). It is important to bear in mind that even on a statin, the population with a combination of high TGs and low HDL-c had a 70% higher event rate than those who did not meet this criteria (456 events [17.3%] vs. 2284 events [10.1%]), which represents a very high residual risk. The fibrate worked well in this population based on the absolute risk reduction (485 [12.4%] events with the combination vs. 456 [17.3%] with the statin alone). The addition of a fibrate thus seems to provide a substantial benefit in patients with high TGs and low HDL-c. In terms of number needed to treat, 20 patients with type 2 diabetes and atherogenic dyslipidemia would need to be treated for 5 years to prevent a single CV event. This compares favorably with results from other trials with fibrates, including the Helsinki Heart Study (HHS), Bezafibrate Infarction Prevention (BIP) study, Veterans Affairs High-Density Lipoprotein Cholesterol Intervention Trial (VAHIT), and Fenofibrate Intervention and Event Lowering in Diabetes (FIELD), which all show substantial risk reductions in this population.
Statin-Niacin Combination
Niacin, or nicotinic acid, is a soluble B vitamin that has favorable effects on all major lipid subfractions but has limited use because of its side-effect profile. The specific mechanism of action of niacin is not well understood, but it appears to reduce ApoB secretion, thereby lowering both VLDL and LDL, increasing ApoA-I, and lowering lipoprotein A [Lp(a)]. However, in contrast to previous dogma, data now suggest that niacin also increases the secretion of HDL ApoA-I by upregulating both adenosine triphosphate–binding cassette transporter (ABCA1) and ApoA-I gene expression in the liver. This results in the expression of very large HDL particles that are more slowly catabolized because of their increased size. Niacin was one of the first lipid-altering drugs to demonstrate a reduction in CHD events in the Coronary Drug Project. At present, niacin is the most effective HDL-raising therapy. Although the mechanism by which niacin raises HDL is not well understood, the predominant hypothesis was that niacin inhibits the holoparticle uptake of HDL, resulting in delayed catabolism. More recent data, however, suggest that niacin may increase ApoA-1 production. Niacin has been demonstrated to lower LDL by 10% to 20%, TGs by 20% to 40%, and Lp(a) by 10% to 30%, and it raises HDL by 15% to 30%.
Statin-niacin combination therapy has been used to reduce residual CV risk. Niacin is often added to a statin in patients with combined hyperlipidemia, especially if the HDL is low or Lp(a) is high. Although statins have demonstrated an approximate reduction in CHD events by 30%, combination therapy with statins and niacin has resulted in reductions of 75%. This significant reduction in CHD events suggests that other effects of niacin—such as TG lowering, Lp(a) lowering, and HDL raising—may also contribute to the benefits.
Adding niacin to a statin is a likely combination because niacin has the most significant effects on raising HDL. A number of trials have demonstrated the safety of this combination and its efficacy in inhibiting the progression of atherosclerosis. Most trials have used either immediate-release niacin or extended-release niacin. Because of the safety data of extended-release niacin in combination with a statin, there has been increased interest in the use of combination therapy to maximize risk reduction in patients with dyslipidemia.
In the HDL Atherosclerosis Treatment Study (HATS), the group treated with simvastatin (10 to 20 mg/day) plus niacin (2 to 4 g/day) showed a significant risk reduction of 90% for composite CV endpoints—death from coronary causes, confirmed MI or stroke, or revascularization—compared with those taking placebo ( P = .03). In addition, a 0.4% regression in coronary stenosis was reported with simvastatin-niacin combination therapy, but progression was seen in other treatment groups receiving antioxidants alone, simvastatin plus niacin plus antioxidants, or placebo ( P < .001). Studies to evaluate statin-niacin combination therapy have consistently demonstrated the efficacy in increasing HDL-c and reducing TG and LDL-c. Additional studies to compare statin monotherapy with statin-niacin combination therapy indicate that statins and niacin have additive effects on HDL-c. Angiographic studies further support the effect of statin-niacin combination therapy in impeding the progression of CHD.
As demonstrated by the Arterial Biology for the Investigation of the Treatment Effects of Reducing Cholesterol (ARBITER) 2 trial, adding extended-release niacin to statin therapy increased HDL-c by 21% and slowed the progression of atherosclerosis, as measured by change in carotid intima media thickness (CIMT), compared with statin therapy alone in patients with known CHD and low HDL-c levels. The ARBITER 6-HALTS (HDL and LDL Treatment Strategies) trial was designed to compare the effects of LDL-c lowering with ezetimibe or HDL-c raising with niacin in combination with a statin on CIMT. Patients with CHD or a CHD equivalent, receiving stable statin treatment, with LDL-c below 100 mg/dL and HDL-c below 50 mg/dL for men or 55 mg/dL for women were randomly assigned to ezetimibe (10 mg/day) or extended-release niacin (target dose, 2000 mg/day). The primary endpoint was change in mean CIMT, analyzed according to a last observation carried forward method. The original study was terminated early on the basis of a prespecified interim analysis that showed superiority of niacin over ezetimibe on change in CIMT. Niacin and ezetimibe both produced the expected, and approximately equal, reductions in non–HDL-c, but niacin raised HDL-c by 18.4%. Niacin (n = 154) resulted in significant reduction (regression) in mean CIMT (−0.0102 ± 0.0026 mm; P < .001) and maximal CIMT (−0.0124 ± 0.0036 mm; P = .001), whereas ezetimibe (n = 161) did not reduce mean CIMT (−0.0016 ± 0.0024 mm; P = .88) or maximal CIMT (−0.0005 ± 0.0029 mm; P = .88) compared with baseline. A significant difference was observed that favored niacin for both mean CIMT ( P = .016) and maximal CIMT ( P = .01). By lowering non–HDL-c and raising HDL-c, niacin induces regression of CIMT in high-risk patients with low HDL-c, whereas an LDL-c–lowering strategy with ezetimibe in combination with a statin stops progression.
The Atherothrombosis Intervention in Metabolic Syndrome with Low HDL Cholesterol/High Triglyceride and Impact on Global Health Outcomes (AIM-HIGH) trial was a multicenter clinical trial carried out at approximately 90 sites in the United States and Canada. AIM-HIGH was meant to test whether adding high-dose, extended-release niacin to a statin (simvastatin) is better than a statin alone in reducing long-term CV events in participants whose LDL-c was controlled and who had a history of CVD, low levels of HDL cholesterol, and high levels of TGs. The National Heart, Lung, and Blood Institute (NHLBI) of the National Institutes of Health (NIH) stopped the AIM-HIGH clinical trial 18 months earlier than planned. The trial found that adding high-dose, extended-release niacin to statin treatment in people with heart and vascular disease did not reduce the risk of CV events, including heart attacks and stroke.
It is unclear why the HDL-c–raising effects of niacin did not reduce CV events compared with aggressive treatment to LDL-c targets. Niacin treatment has different effects on the cholesterol and particle measures of LDL and HDL, and it significantly raises HDL-c but without increasing HDL particle number (HDL-p). In addition, it modestly reduces LDL-c, but it usually lowers LDL particle number (LDL-p) much more. The AIM-HIGH trial assessed the efficacy of increasing HDL-c and correcting other non-LDL lipid abnormalities with niacin in CHD patients with optimally treated LDL-c, but the trial design precluded assessment of the potential benefit of LDL-p lowering.
Statin–Omega-3 Fatty Acids Combination
Omega-3 fatty acids, or fish oils, are essential fatty acids thought to inhibit VLDL and TG synthesis in the liver, although the exact molecular mechanisms are not well understood. Omega-3 fatty acids significantly reduce TG levels and increase LDL-c levels in patients with high TG levels. Dietary supplementation with the n-3 polyunsaturated fatty acids (PUFAs) eicosapentaenoic acid (EPA) and docosahexaenoic acid (DHA) lowers the risk of death, nonfatal coronary events, and stroke after MI. PUFAs reduce TG levels by 20% to 30% in most patients, but they reduce TGs by up to 50% in patients with severe hypertriglyceridemia (TG >750 mg/dL [>8.47 mmol/L]). Studies of combination therapy with n-3 PUFAs and pravastatin 40 mg/day or simvastatin 20 mg/day demonstrated LDL-c reductions of 13% to 24% and TG reductions of 27% to 30%, respectively. Similarly, combination therapy with atorvastatin 10 mg resulted in significant reductions of the concentration of small, dense LDL particles and increases in HDL-c compared with monotherapy.
A highly purified pharmaceutical grade omega-3 fatty acid marine fish oil formulation contains high concentrations of EPA (440 mg) and DHA (260 mg), along with 4 mg (6 IU) vitamin E in each 1-g capsule. Prescription omega-3 fatty acid marine oils are indicated for the treatment of hypertriglyceridemia, and they significantly reduce serum TGs by 19% to 55% at doses of 4 capsules per day when administered over periods from 6 weeks to several years. In the Combination of Prescription Omega-3 With Simvastatin (COMBOS) trial, median percentage decreases in non–HDL-c were significantly greater with combination therapy, using simvastatin 40 mg and omega-3-acid ethyl esters 4 g/day, compared with placebo plus simvastatin (9.0% vs. 2.2% respectively; P < .001). In addition, combination therapy significantly lowered TG (29.5%) and VLDL-c (27.5%) levels, raised HDL-c (3.4%), and lowered total cholesterol/HDL-c ratio (9.6%; P ≤ .001 vs. placebo for all); however, LDL-c increased by 3.5%.
In a post hoc analysis of data from the COMBOS study, the predictors of the LDL-c response to prescription omega-3-acid ethyl esters (P-OM3) therapy was the baseline LDL-c. The median LDL-c response in the P-OM3 group was +9.5% (first tertile, <80.4 mg/dL), −0.9% (second tertile), and −6.4% (third tertile, ≥99.0 mg/dL). Non-HDL-c, VLDL-c, HDL-c, and TG responses did not vary significantly by baseline LDL-c tertile. The reductions in VLDL-c concentrations were greater than the increases in LDL-c, where present, resulting in a net decrease in the concentration of cholesterol carried by atherogenic particles (non–HDL-c) in all baseline LDL-c tertiles. In conclusion, these results suggest that the increase in LDL-c that occurred with the addition of P-OM3 to simvastatin therapy in patients with mixed dyslipidemia was confined predominantly to those with low LDL-c levels while receiving simvastatin monotherapy. In addition, LDL-p and ApoB are reduced with P-OM3 in combination with a statin. These data suggest that the increase in LDL-c with omega-3 therapy is an increase in LDL-p size rather than particle number. Compared with placebo, P-OM3 reduced mean VLDL particle (VLDL-p) size and increased LDL-p size ( P = .006 for both) without altering HDL-p size.
The doses of EPA and DHA obtainable from typical consumption of fatty fish and unprocessed marine oil products (~30% omega-3 fatty acids) are insufficient for the treatment of severe hypertriglyceridemia. This has prompted the development of concentrated forms of omega-3 fatty acids. Prescription omega-3-acid ethyl esters (Lovaza), produced by ethanol extraction and distillation of omega-3 fatty acids, contain 84% EPA plus DHA ethyl esters and are approved by the U.S. Food and Drug Administration (FDA) as an adjunct to diet for the treatment of severe hypertriglyceridemia in adults. Subsequently, a product has been developed that undergoes an additional manufacturing step to hydrolyze and distill the ethyl esters into omega-3 free fatty acids (FFAs).
The Epanova Compared to Lovaza in a Pharmacokinetic Single-Dose Evaluation (ECLIPSE) study assessed the relative bioavailability of a single 4-g oral dose of the FFA omega-3 product compared with ethyl ester omega-3 in 54 healthy volunteers, and it furthermore examined the effect of low-fat and high-fat diet on relative bioavailability. Significantly higher plasma EPA and DHA levels (peak concentrations and areas under the curve) were observed with omega-3 FFA compared with omega-3 ethyl ester in both the low-fat and high-fat diet conditions. Most notably, in the low-fat diet condition, the single dose of the omega-3 FFA resulted in an area under the curve (AUC) for EPA plus DHA that was four times higher than that in the omega-3 ethyl ester condition. Unlike the triglyceride and ethyl ester forms of omega-3 fatty acids, the FFA form does not require pancreatic lipase hydrolysis; thus, as shown in ECLIPSE, it has greater bioavailability, particularly when consumed in conjunction with a low-fat diet after a single dose.
A concern with some triglyceride-lowering therapies is their potential LDL-c–elevating effect. Fibrates and fish oil increase LDL-c by as much as 35% to 45% when administered to patients with severe hypertriglyceridemia; this is due in part to an increased rate of conversion of VLDL to LDL and results in an increase in LDL-p and a rise in LDL-c concentration. Reducing the substrate for CETP may also play a role, because this enzyme catalyzes the exchange of triglyceride from VLDL for cholesteryl ester from LDL and HDL particles. Thus, reducing the triglyceride concentration tends to result in larger, more cholesterol-rich LDL and HDL particles. A highly purified form of EPA—ethyl eicosapentate, or AMR101—contains at least 96% EPA ethyl ester and no DHA. The Multi-Center, Placebo-Controlled, Randomized, Double-Blind, 12-Week Study with an Open-Label Extension (MARINE) trial examined doses of 2 and 4 g/day of AMR101 among 229 diet-stable patients with very high TGs (≥500 and ≤2000 mg/dL, with or without background statin therapy). The study resulted in TG reductions of 19.7% ( P < .005) and 33.1% ( P < .0001), respectively, but AMR101 did not significantly increase placebo-corrected LDL-c levels (+5.2% and −2.3%, respectively).
When TG and LDL-c responses to AMR101 are examined against results from trials of omega-3 EPA plus DHA ethyl ester, which have been shown to increase LDL-c by 0.7% to 46%, the increase in LDL-c with 2 g/day AMR101 is in line with the expected change in LDL-c with a 20% decrease in TG, but the LDL-c response to 4 g/day AMR101 (a 2.3% reduction) is lower than predicted and should be explored further. Median baseline LDL-c concentration, which has been reported to be inversely associated with the change in LDL-c during treatment, was 86.0 mg/dL in MARINE. A systematic review of the individual effects of EPA and DHA on serum LDL-c and other lipoprotein lipid concentrations reported that whereas both EPA and DHA reduced TG, DHA was associated with increases in LDL-c that were directly and significantly associated with the baseline TG concentration. In four of the six studies, these elevations were not observed after EPA treatment. DHA, and not EPA, also appears to increase HDL and LDL particle size.
These results are consistent with previous head-to-head comparisons of pure EPA to DHA, which demonstrated that EPA supplementation resulted in less TG lowering and HDL-c raising than DHA, and DHA increased LDL-c more than EPA, although this increase in LDL-c was found to be associated with a greater increase in LDL-p, albeit in a limited dataset. Further research is needed to investigate the effects of EPA versus DHA on LDL-p and its clinical significance.
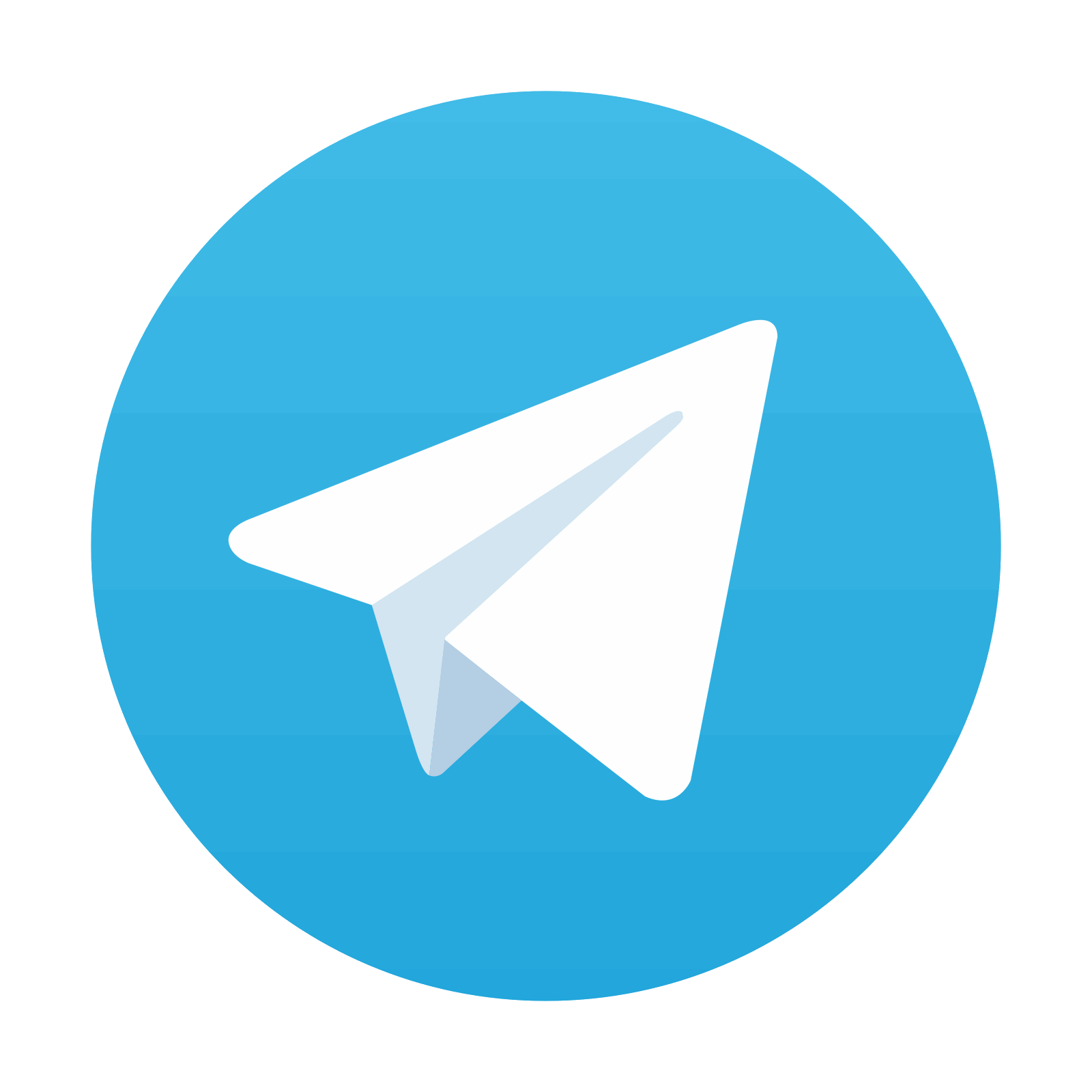
Stay updated, free articles. Join our Telegram channel

Full access? Get Clinical Tree
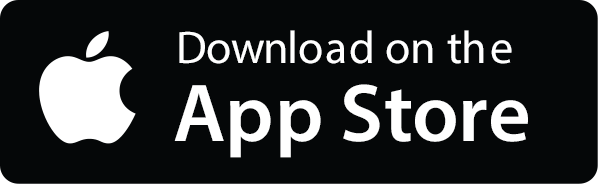
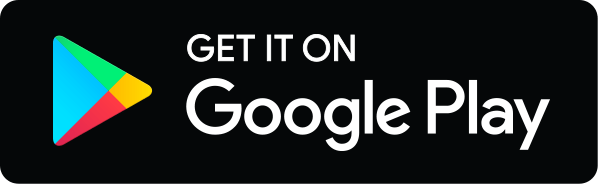