Background
The aims of this study were to evaluate the three-dimensional features and geometric determinants of the vena contracta (VC) in functional tricuspid regurgitation (TR) and to identify optimal width cutoff values for assessing functional TR severity.
Methods
Real-time three-dimensional full-volume and color Doppler and two-dimensional Doppler echocardiographic images were obtained in 52 patients with various degrees of functional TR and in sinus rhythm. The tricuspid valve and right ventricle were geometrically analyzed. VC widths parallel to the septal-lateral and anteroposterior directions, VC area, and effective regurgitant orifice area (EROA) using proximal isovelocity surface area methods on real-time three dimensional color Doppler images were measured.
Results
The septal-lateral VC width was 0.39 ± 0.37 cm smaller than the anteroposterior VC width ( P < .001). VC widths and area were strongly correlated with EROA. The optimal cutoff values for the septal-lateral VC width, anteroposterior VC width, and VC area were 0.63 cm, 0.76 cm, and 0.37 cm 2 , respectively, for moderate functional TR (EROA ≥0.2 cm 2 ) and were 0.84 cm, 1.26 cm, and 0.57 cm 2 , respectively, for severe functional TR (EROA ≥0.4 cm 2 ). Multiple linear regression analyses showed that the septal leaflet tenting angle and septal-lateral annular diameter independently determined septal-lateral VC width, while the anterior leaflet tenting angle and anteroposterior annular diameter independently determined the anteroposterior VC width.
Conclusions
Different VC width cutoff values should be applied according to the plane of view in functional TR, because the VC cross-sectional shape is ellipsoidal with a long anteroposterior direction. VC widths are determined by annular dilation and leaflet tenting in the corresponding directions.
Tricuspid regurgitation (TR) is associated with a poor prognosis irrespective of underlying disease. The American Society of Echocardiography recommends that the vena contracta width be used in quantification of TR. Although a vena contracta width >0.7 cm is regarded as a marker for severe TR, there is no established vena contracta width cutoff value for differentiating between moderate and mild TR. The vena contracta cross-sectional shape was reported to be more ellipsoid in TR compared with mitral regurgitation. It appears, however, that no study has examined the vena contracta in functional TR, a major etiology of TR. If the vena contracta cross-sectional shape in functional TR is not circular, then different cutoff values should be applied on the basis of the two-dimensional plane used for viewing. However, this issue has not been resolved yet. The tricuspid valve has three leaflets, which results in coaptation along three radial lines between the leaflets. It is not known whether the vena contracta cross-sectional shape in functional TR is triangular or ellipsoidal. If it is ellipsoidal, the direction of the maximal vena contracta width should be identified. Furthermore, although annular dilation and leaflet tethering have been suggested as causes of functional TR, the effect of remodeling of the right ventricle on functional TR severity remains to be clarified. In addition, the effect of geometric factors in tricuspid valve apparatus and the right ventricle on vena contracta width in functional TR have not been determined. Therefore, we sought to evaluate three-dimensional features of vena contracta in functional TR and geometric determinants for vena contracta and to determine the optimal cutoff values of vena contracta width obtained from different views for assessment of functional TR severity, using real-time three-dimensional color Doppler echocardiography.
Methods
We enrolled 52 adult patients (mean age, 54 ± 17 years; 30 women) with various degrees of functional TR and in sinus rhythm. Excluded from the study were patients with definite organic deformation, restricted tricuspid valve motion, in atrial fibrillation, or with frequent premature beats. A real-time three-dimensional echocardiography system (Sonos 7500 or iE33; Philips Medical Systems, Andover, MA) with a 2-MHz to 4-MHz 3,000-element xMATRIX transthoracic transducer was used to acquire three-dimensional full-volume and color Doppler images. Images were obtained from the low parasternal or apical views with gain, compression controls, and time gain compensation settings optimized for image quality. Care was taken to include the entire right ventricle and tricuspid valve apparatus in the full-volume data set. Full-volume data sets were acquired in wide-angle acquisition mode (93° × 80°), in which four wedge-shaped subvolumes (93° × 20° each) were obtained from four different cardiac cycles during held respiration. Three-dimensional color Doppler image volumes were acquired as seven subvolumes gated to form a full Doppler volume (30° × 30°). Care was taken to include proximal convergence and the entire vena contracta in the color Doppler image set. All images were stored digitally and analyzed offline.
Geometric analyses of the right ventricle and tricuspid valve were performed using TomTec software (TomTec GmbH, Munich, Germany). On the multiplanar reconstruction images, the tenting angles of the three tricuspid valve leaflets, septal-lateral, and anteroposterior tricuspid annular diameters and right ventricular (RV) inlet dimensions, septal-lateral mid-RV dimension, and the distance between the annulus and apex were measured in a midsystolic frame, when tricuspid valve tenting was smallest. By the guidance of a moving cross-sectional image of tricuspid valve, three longitudinal planes that crossed the midportions of three leaflets were obtained ( Figure 1 A). The tenting angle of each leaflet was measured on each longitudinal plane. By adjusting the orientation of the cross-sectional short-axis plane at the annular level, we generated septal-lateral and anteroposterior longitudinal planes that were mutually perpendicular ( Figure 1 B). On these two longitudinal planes, annular diameters and RV inlet dimensions were measured. RV inlet dimensions were measured 1 cm apart from, and parallel to, annular lines. Septal-lateral mid-RV dimension was measured, but mid-RV dimension on the anteroposterior plane could not be consistently measured because the RV outflow tract was located on the anterior side. The distances from the annular plane to RV apex on the two longitudinal planes were measured. If those two values were not equal, the larger number was chosen. All measurement points marked on real-time three-dimensional echocardiographic still frames were verified by overlap with moving images. We also measured end-diastolic and end-systolic RV volumes and RV ejection fraction using a four-dimensional RV analysis program.

QLAB (Philips Medical Systems) software was used for analysis of real-time three-dimensional color Doppler images. From those images, we generated cross-sectional images of the vena contracta using multiplanar reconstruction images ( Figure 2 A). In detail, septal-lateral and anteroposterior longitudinal planes were generated by adjusting the longitudinal plane axes on the cross-sectional short-axis image (green and red lines in the bottom left panel) by the guidance of moving cross-sectional color and color-suppressed images of tricuspid annulus. Then, the location and direction of the longitudinal plane axes (red and green lines in the top panels) were adjusted along the longitudinal mid-portion of the TR jets in a midsystolic frame, and the cross-sectional planes (blue lines in the upper panels) were moved to become orthogonal to the jet direction and consequently parallel to the vena contracta. The location of the cross-sectional plane was determined by the guidance of longitudinal and cross-sectional color images and set to the point at which the jet widths and cross-sectional area were smallest. Generally, the location of the vena contracta was more easily noticed in the septal-lateral than in the anteroposterior longitudinal plane in most cases. We confirmed using color-suppressed images that the locations of the cross-sectional planes (blue lines in the upper panels) were on the right atrial side of the tricuspid valve tips on the longitudinal planes ( Figure 2 B). The cross-sectional images were used to measure the septal-lateral vena contracta width, the anteroposterior vena contracta width, and the vena contracta area ( Figure 3 ). Care was taken not to include blurred color signal artifacts into the vena contracta area by double-checking with the color and color-suppressed longitudinal and cross-sectional images. The vena contracta circular index was defined as the septal-lateral vena contracta width divided by the anteroposterior vena contracta width. For quantification of functional TR severity, the effective regurgitant orifice area (EROA) was calculated using a proximal isovelocity surface area (PISA) method with the aliasing isovelocity set to about 28 cm/s. EROA was calculated as follows: EROA = (2π r 2 × V a )/ P k V TR , where r is the radius from the orifice to the PISA outer shell obtained from real-time three-dimensional color Doppler images, V a is the aliasing velocity, and P k V TR is the maximal velocity of the regurgitation TR jet obtained from two-dimensional continuous-wave Doppler echocardiography. Moderate TR was defined as EROA ≥0.2 cm 2 , while severe TR was defined as EROA ≥0.4 cm 2 . For evaluation of interobserver variability, septal-lateral and anteroposterior vena contracta widths, vena contracta area, and EROA were measured by two independent investigators using real-time three-dimensional color Doppler images of 15 randomly selected patients.


Vena contracta widths were also measured from the low parasternal four-chamber and parasternal RV inflow views using two-dimensional color Doppler echocardiography in 10 patients ( Figure 4 ).

Statistical analyses were performed using SPSS (SPSS, Inc., Chicago, IL). Data are expressed as mean ± SD. Interobserver variability was evaluated by means of the intraclass correlation coefficient. Comparisons between two paired variables were performed using paired t tests. Receiver operating characteristic curve analysis was performed to determine the best cutoff values for the identification of moderate and severe TR. Univariate linear regression analysis was used to evaluate the correlation between two continuous variables. Multivariate analysis was used to identify independent geometric determinants of vena contracta widths and EROA. That based on stepwise multiple linear regression analysis was implemented, and the final model, which included variables with P values < .10, was derived. P values < .05 were considered to indicate significance.
Results
Table 1 shows the mean absolute measurement values of echocardiographic parameters. As indicators of interobserver variability in three-dimensional measurements of TR severity, intraclass correlation coefficients were 0.863 ( P < .001) for septal-lateral vena contracta width, 0.894 ( P < .001) for anteroposterior vena contracta width, 0.780 ( P = .004) for vena contracta area, and 0.939 ( P < .001) for EROA.
Variable | Value |
---|---|
Septal-lateral VC width (cm) | 0.54 ± 0.35 |
Anterolateral VC width (cm) | 0.93 ± 0.63 |
VC area (cm 2 ) | 0.61 ± 0.67 |
EROA (cm 2 ) | 0.22 ± 0.22 |
Maximal velocity of TR (cm/sec) | 295 ± 71 |
Tenting angles (°) | |
Septal | 24 ± 17 |
Anterior | 21 ± 13 |
Posterior | 26 ± 12 |
Annular diameters (cm) | |
Septal-lateral | 3.2 ± 0.7 |
Anteroposterior | 3.5 ± 0.7 |
RV dimensions (cm) | |
Septal-lateral RV inlet | 3.7 ± 1.0 |
Anteroposterior RV inlet | 4.8 ± 1.0 |
Septal-lateral mid-RV | 3.1 ± 1.0 |
Annulus to apical distance (cm) | 6.1 ± 1.2 |
RV volumes (mL) | |
RV end-diastolic | 158 ± 76 |
RV end-systolic | 100 ± 60 |
RV ejection fraction (%) | 40 ± 10 |
Vena contracta widths measured on the septal-lateral ( r = 0.782, P < .001) and anteroposterior ( r = 0.761, P < .001) planes were found to strongly correlate with EROA ( Figure 5 ). Vena contracta area also strongly correlated with EROA ( r = 0.808, P < .001), but the former was significantly larger than the latter (0.61 ± 0.67 vs 0.22 ± 0.22 cm 2 , P < .001). The anteroposterior vena contracta width was found to be 0.39 ± 0.37 cm larger than the septal-lateral vena contracta width ( P < .001), and the difference between the two width values increased as EROA increased. The mean vena contracta circular index was 0.68 ± 0.30 (range, 0.21–2.00) for the whole study population and was 0.57 ± 0.18 (range, 0.21–0.95) for patients with moderate and severe functional TR.

In receiver operating characteristic curve analysis, the areas under the curves for the septal-lateral vena contracta width, anteroposterior vena contracta width, and vena contracta area as determined by EROA were 0.878, 0.910, and 0.903, respectively, for moderate functional TR ( Figure 6 ), and 0.891, 0.896, and 0.889, respectively, for severe TR. The optimal cutoff values for septal-lateral vena contracta width, anteroposterior vena contracta width, and vena contracta area were found to be 0.63 cm (sensitivity, 82%; specificity, 83%), 0.76 cm (sensitivity, 100%; specificity, 66%), and 0.37 cm 2 (sensitivity, 100%; specificity, 69%), respectively, for moderate functional TR, and 0.84 cm (sensitivity, 75%; specificity, 91%), 1.26 cm (sensitivity, 88%; specificity, 80%), and 0.57 cm 2 (sensitivity, 100%; specificity, 64%), respectively, for severe functional TR.
Vena contracta widths measured from the low parasternal four-chamber view on two-dimensional color Doppler images tended to be smaller than those measured from the RV inflow view, but there was no statistical significance (0.97 ± 0.34 vs 1.02 ± 0.44 cm, P = .651) in 10 patients. Septal-lateral and anteroposterior vena contracta widths measured from three-dimensional color Doppler images correlated significantly with vena contracta widths measured from the low parasternal four-chamber view and the RV inflow view on two-dimensional color Doppler images ( r = 0.641, P = .046, and r = 0.810, P = .004), respectively ( Figure 7 ). Anteroposterior vena contracta width was significantly larger than vena contracta width measured from the RV inflow view (1.33 ± 0.41 vs 1.02 ± 0.44 cm, P = .005), whereas septal-lateral vena contracta width tended to be smaller than vena contracta width measured from the low parasternal four-chamber view (0.78 ± 0.29 vs 0.97 ± 0.34 cm, P = .051).

Univariate analysis identified a number of variables that correlated significantly with septal-lateral vena contracta width ( Table 2 ) and anteroposterior vena contracta width ( Table 3 ). Multiple stepwise regression analysis showed that the septal leaflet tenting angle and the septal-lateral annular diameter were independent determinants of the septal-lateral vena contracta width ( Table 2 ), while the anterior leaflet tenting angle and the anteroposterior annular diameter were independent determinants of the anteroposterior vena contracta width ( Table 3 ). In addition, the septal leaflet tenting angle, septal-lateral annular diameter, and septal-lateral RV inlet dimension were found to be major determinants of EROA ( Table 4 ).
Variable | r | Univariate P | Multivariate P |
---|---|---|---|
Age | 0.098 | .488 | |
Maximal velocity of TR | 0.358 | .009 | |
Tenting angles | |||
Septal | 0.553 | <.001 | .003 |
Anterior | 0.558 | <.001 | |
Posterior | 0.539 | <.001 | |
Annulus diameters | |||
Septal-lateral | 0.585 | <.001 | .001 |
Anteroposterior | 0.387 | .005 | |
RV dimensions | |||
Septal-lateral RV inlet | 0.459 | .001 | |
Anteroposterior RV inlet | 0.363 | .008 | |
Septal-lateral mid-RV | 0.390 | .004 | |
Annulus to apical distance | 0.341 | .013 | |
RV volumes | |||
RV end-diastolic | 0.406 | .003 | |
RV end-systolic | 0.448 | .001 | |
RV ejection fraction | −0.480 | <.001 |
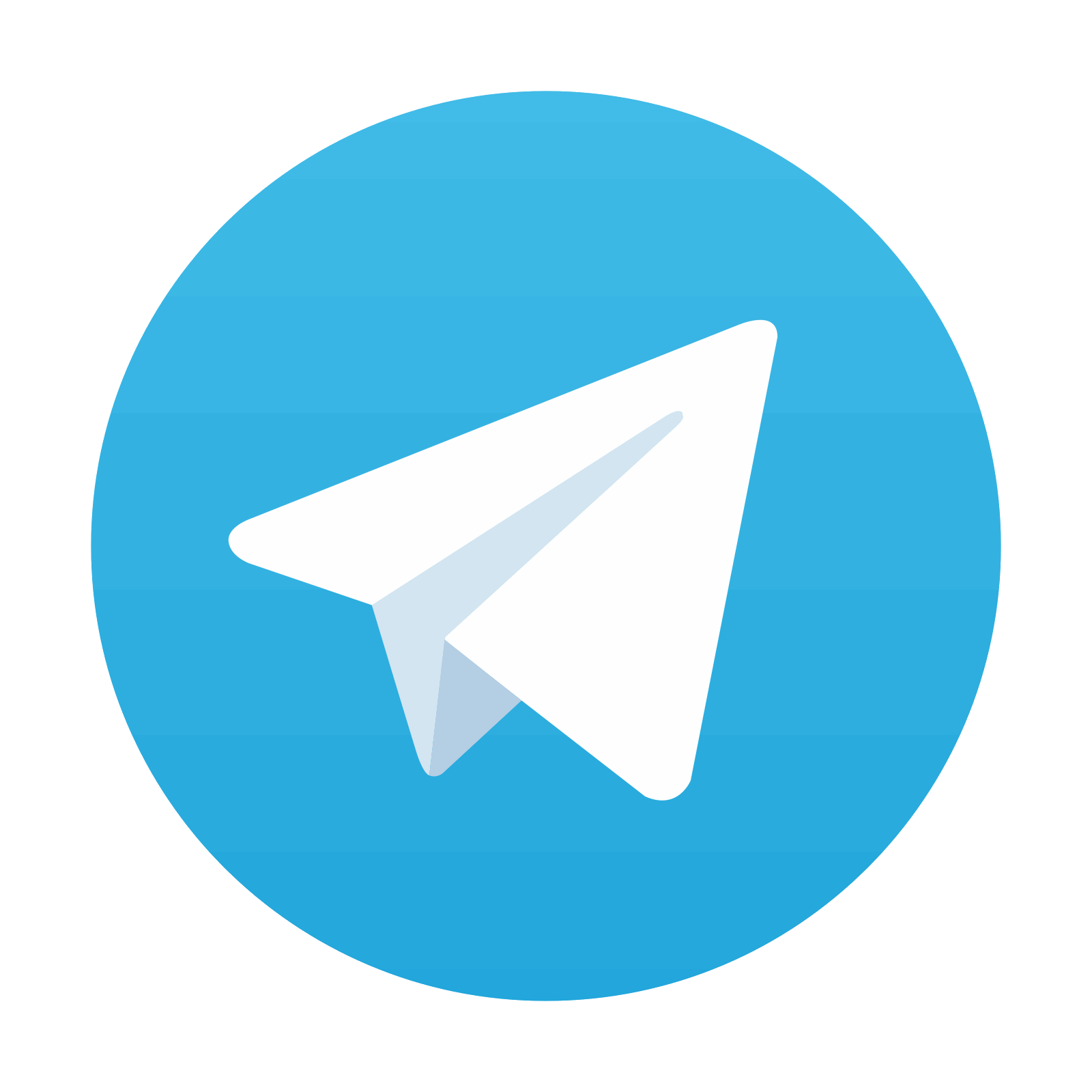
Stay updated, free articles. Join our Telegram channel

Full access? Get Clinical Tree
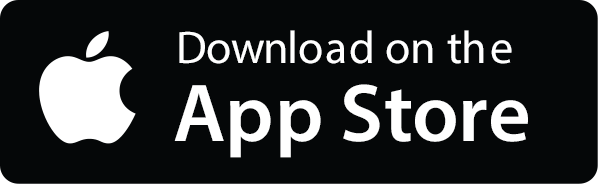
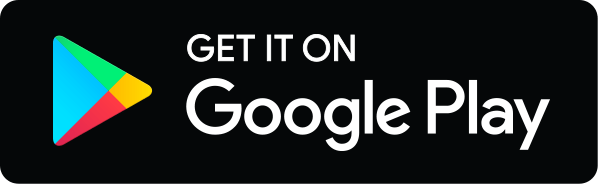
