Fig. 18.1
Mesenchymal stromal cell (MSC) differentiation was confirmed via multi-lineage differentiation. (a, b) WT and BMPR2 mutant iPSC were differentiated to MSC. Subsequent MSC were analyzed for their potential to differentiate into the mesenchymal lineages of bone, fat, and cartilage. (c–f) Representative images of mesenchymal lineage differentiation. (c) Von Kossa stain was used to detect the accumulation of calcium. (d) Oil red O localized the appearance of lipid droplets. (e–f) Alcian blue was used to stain matrix characteristic of chondrocyte microspheres. Scale bar = 500 μM
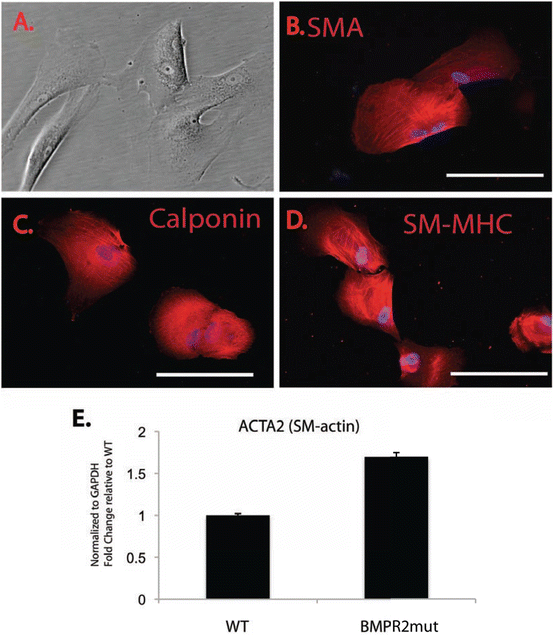
Fig. 18.2
Smooth muscle cell differentiation of PAH iPSC cells. WT and BMPR2 mutant iPSC were differentiated to smooth muscle cells (SMC). (a) Representative phase image of PAH SMC. (b–d) Successful SMC differentiation was demonstrated by immunofluorescent staining to localize and detect smooth muscle alpha actin (SMA), calponin, and smooth muscle myosin heavy chain (SM-MHC). The proteins localized to the cytoskeletal elements. 200× mag. (e) Quantitative PCR was confirmed differential expression of smooth muscle alpha actin message (Acta2) in WT and BMPR2 mutant iPSC-derived SMC
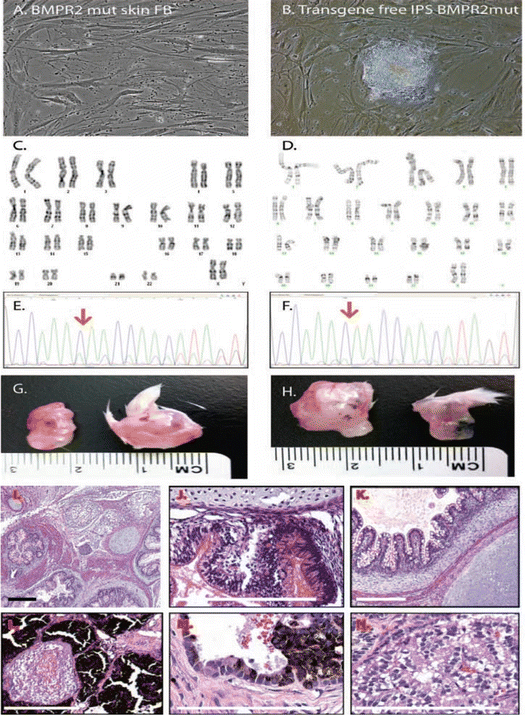
Fig. 18.3
Characterization of PAH iPSC cells from mutant skin fibroblasts. (a, b) iPSC cell lines were engineered using the STEMCCA vector and an HPAH patient primary skin fibroblast cell line containing a mutation in exon 12 of the BMPR2 gene. (c, d) Karyotype was confirmed by banding analysis in both the fibroblast (FB) and iPSC cells. (e, f) The HPAH patient primary skin fibroblast cell lines contained a mutation in exon 12 (nucleotide change 2504delC) of the BMPR2 gene that localized to the cytoplasmic domain of the receptor. Sequencing of BMPR2 mutant iPSC genomic DNA confirmed the retention of this mutation in the BMPR2 mutant iPSC cell clones. In the sequence photo, “C” is denoted by the blue color. In the native cell HPAH skin FB, there is a large blue peak, and a smaller green peak. That smaller green peak is actually a shift of the next nucleotide, since the green would be “next”. Red arrow indicates the mutation site. (g, h) Representative images of teratoma analysis of the wildtype (WT) and BMPR2 mutant iPSC cell lines. (i–n) Representative images of (h, e) stained sections from the BMPR2 mutant iPSC cell-derived teratomas. Visible cell types include: mesodermal proliferation, adipocytes, cyst formation, epithelial cells (gut, respiratory, mucous producing), melanocytes (hypersecreting), and chondrocytes. Scale bar = 100 μM
In first study to report reprogramming of iPSC from PAH patient samples, Geti and colleagues utilized late outgrowth endothelial precursor cells (L-EPCs) isolated from peripheral blood mononuclear cells (Geti et al. 2012). These studies demonstrated the feasibility and high efficiency of reprogramming control and PAH (HPAH and IPAH) L-EPC to iPSC using integrating retroviral technology. The resultant iPSC were capable of clonal proliferation and retained normal karyotype, providing evidence that this method is an ideal tool for high-throughput pharmacological screening.
West et al. utilized both excisable and non-integrating reprogramming techniques to engineer control, IPAH, and HPAH patient iPSC lines in a multifaceted approach to evaluate molecular pathways affected by dysregulated BMPR2 signaling (West et al. 2014). While integrating retroviral reprogramming is associated with high efficiency reprogramming, they also have the potential to mask subtle mutations and changes within the genome, hence the choice for excisable or non-integrating approaches to generate iPSC lines. In these experiments, control and PAH iPSCs were differentiated to MSC and endothelium. PAH patient iPSC-derived cells maintained a “PAH” phenotype in vitro, including decreased expression of Tie2, VEGF-A, Flk-1/KDR, and BMP-4, all factors involved in the regulation of vascular stability. Additionally, MSC exhibited decreased rates of apoptosis and increased oxidant stress following exposure to hypoxia (West et al. 2014). Further, gene expression profiles during differentiation across iPSC-derived mesenchymal and endothelial cells were compared to skin fibroblasts from control, HPAH, and IPAH patients, addressing the hypothesis that altered human BMPR2 signaling would result in a genetic signature common across multiple cell types. Common molecular pathways affected by deregulated BMPR2 signaling were identified, the most prominent being Wnt. BMPR2 signaling may regulate both canonical and noncanonical Wnt pathways in endothelium and mesenchymal cells to influence proliferation, survival, and motility during angiogenesis and remodeling of the pulmonary circulation (Laumanns et al. 2009; de Jesus Perez et al. 2011; Alfaro et al. 2010). While the relationship of BMPR2 and Wnt pathways has been defined during development, the regulatory targets of Wnt signaling PAH are largely unknown.
Taken together, these studies exploited iPSC technology and linked deregulated developmental pathways with adult disease, over multiple cell types and differentiation states. Combinations of iPSC and primary patient cell modeling may ultimately enable the identification of cellular defects that initiate the vascular pathology in PAH and provide access to multiple renewable cell types in which to test potential therapies (West et al. 2014; Geti et al. 2012).
18.4.3 Cardiac Myocyte Lineage
Critical differences exist between human and animal cardiomyocytes including mechanical and physiological properties (Sheng and Charles 2013). For example, the mouse heart beats seven times faster and is 1,000-fold smaller than human. While human ESCs may be used to model cardiac diseases, there exist significant obstacles including limited availability of embryonic tissues and ESC lines as well as ethical controversies hindering scientific progress using these experimental techniques. The use of stem cells to model cardiac disease in humans is ongoing especially advancing in those diseases with a pronounced clinical presentation or inherited genetic component (Sheng and Charles 2013; Moretti et al. 2010, 2013). Recent work by our group and others has suggested that BMPR2 may have independent effects on the RV in PAH that cannot be explained by pulmonary vascular disease with evidence of RV lipotoxicity (Hemnes et al. 2013; McGoon et al. 2013). Because of the limitations to animal models and access to human tissue, exploring the mechanisms of this has thus far been challenging. The underlying BMPR2-dependent molecular mechanisms affected in cardiomyocytes during PAH, either heritable or idiopathic, are unknown and ESC as well as iPSC offer an important tool to investigate these mechanisms.
In vitro studies show that the differentiation of iPSCs into cardiovascular progenitors, and ultimately to mature ECs and cardiomyocytes, is enhanced by proper exposure to BMP morphogens at specific time points of the differentiation process (Kattman et al. 2006; Yang et al. 2008). When directing the differentiation of normal ESCs or iPSCs, antagonists of BMP signaling, such as Noggin and PRDC or chemical compounds such as dorsomorphin, influence both the yield and physiologic properties of stem cell-derived cardiomyocytes (Hao et al. 2008). Thus, iPSCs derived from HPAH patients carrying BMPR2 mutations as well as IPAH patients, with decreased BMP signaling, may manifest differences in cardiac myocyte differentiation compared to iPSCs from healthy individuals without dysfunctional BMP signaling. Thus, iPSCs provide a useful model for comparative studies.
18.5 Murine Embryonic Stem Cell and Induced Pluripotent Stem Cell Models
While significant biological differences exist between rodent and human models of disease, there remains an advantage to using rodent models to study disease processes in an integrative fashion. Starting material is readily available and the ethical concerns associated with human somatic cell nuclear transfer (SCNT) and derivation of ESCs from fertilized embryos are not concerns when studying genetic disease modeled with rodents.
18.5.1 Benefits of Pluripotent Rodent Model Systems
As human iPSC are an invaluable and innovative tool to repeatedly generate multiple cell types from a single clone, murine ESC and iPSC provide the ability to study genetically identical derived cell types indefinitely, from existing, well-characterized rodent models of disease. This ability to re-derive identical cell types from pluripotent lines is in striking contrast to typical primary cell lines isolated from rodent models, which are very heterogeneous in phenotype and proliferate for a limited number of passages. The derivation of ESC or iPSC lines from a rodent model has a few innate differences including the efficiency of generation and degree of manipulation of the starting cell population, which should ideally be minimized to preserve the genomic stability of clones.
Expanding de novo isolated ESC lines is straightforward due to the absence of transfection with vector or infection with virus. If background strain is not a consideration, crossing mutants of interest to an inbred mouse strain (129) can also increase the efficiency of ES cells derivation. Isolation and expansion of ESC requires specific expertise for isolation and cloning of cells from the inner cell mass of a fertilized embryo. In contrast, while iPSC cells require exogenous factors to confer pluripotency, these factors can be administered to any somatic cell type in culture. The specific somatic cell type may influence reprogramming efficiency. While challenging, each technique requires specific expertise.
Both of these model systems in combination with an animal model provide the opportunity to perform gene correction in stem cells and subsequent evaluation of the therapeutic potential of the “autologous” manipulated product in an animal model of disease. Hanna and colleagues performed such a landmark study in 2007 (Hanna et al. 2007). The investigators employed a humanized mouse model of sickle cell anemia to engineer iPSC. Following gene correction of the human sickle hemoglobin allele in iPSC, the cells were differentiated into hematopoietic progenitors and transplanted back into the sickle cell mouse model. Following engraftment of the iPSC-derived hematopoietic cells, the sickle cell defect in iPSC-derived cells was corrected (Hanna et al. 2007).
Another important use of rodent pluripotent stem cells is to further understand the pathogenesis of genetic diseases, which cause embryonic lethality or deformity. One example of such a disease class is lysosomal storage disorders. Using a mouse model of mucopolysaccharidosis (MPSVII), Meng et al. characterized an enzyme deficiency specific to this disease (Meng et al. 2010). Following partial correction of the enzyme deficiency, cell functions and developmental specification of cells improved (Meng et al. 2010).
For the purposes of modeling PAH, there is a paucity of humanized rodent models that would enable study of either adult disease or embryonic lethality, thus demonstrating the benefit of using patient-derived iPSC. To date, such models include the human R899x, delEx4, and delEx2 BMPR2 mutations (Johnson et al. 2012; West et al. 2005, 2008; Majka et al. 2011; Frump et al. 2013). However, ESC may also be stably transfected with variations of mutant human BMPR2 and mechanisms of diseases evaluated in lineage-specific cell derivatives.
18.5.2 KOMP ES Cell Bank
The knockout mouse project-embryonic stem cell repository (KOMP-ES) is an additional resource which may be utilized to study underlying mechanisms of PAH. The KOMP is a sub-portion of an National Institute of health (NIH) initiative in the United States, established with the purpose of creating a comprehensive and public resource comprised of mouse embryonic stem (ES) cells containing a null mutation in every gene in the mouse genome and related materials (mice, sperm, etc.). Programs including but not limited to the NIH-sponsored Knockout Mouse Project (KOMP) (Skarnes et al. 2011), and the European Conditional Mouse Mutagenesis Program (EUCOMM) (Friedel et al. 2007), have combined resources to reach the target goal of murine ES knockout lines representative of each of approximately 20,000 protein-coding genes. Currently, NIH and other agencies have funded the creation and phenotyping of mice from these ESC lines (Ryder et al. 2013). ESC are injected into the blastocoel cavity of 3.5 day old mouse blastocysts. These injected embryos are transferred to the uterine horns of pseudopregnant recipient females. Embryos gestate for approximately 18 days and resulting pups are chimeras, consisting of the ESC and the blastocyst cells. These resources provide valuable tools for the study of heritable mechanisms of human pulmonary and vascular disease in the mouse lines generated as well as in vitro differentiation of primary ES lines to cell types of interest.
18.6 Summary
Both rodent and human pluripotent stem cell technologies, available in ESC and iPSC, offer the potential to investigate a complement of PAH disease spectrum and to characterize genetic and environmental influences on vascular cell phenotype. Moreover, in the years ahead iPSC and their vascular derivatives should promote advancement of PAH research by: (1) providing access to a patient-derived expandable cell population; (2) recapitulating disease pathophysiology in a cell-specific manner; and (3) providing multiple cell types affected in PAH, endothelium, smooth muscle, and cardiac myocytes, with the same genetic makeup. As a caveat to this model system, a single cell type is not likely to recapitulate a complex disease phenotype, thus interaction between multiple cell types may be necessary to recapitulate the disease phenotype. In using pluripotent systems, multiple cell types from a single cell clone can now address complex disease pathogenesis in PAH.
Acknowledgments
This work was funded by grants to S.M. Majka from the NIH NHLBI R01HL091105 and R01HL11659701. We would like to extend our gratitude to Dr. Darrell Kotton for the creation of the first BMPR2 mutant iPSC lines submitted to the Vanderbilt University repository (BMP3a and BMP1a).
References
Adams WJ, Zhang Y, Cloutier J, Kuchimanchi P, Newton G, Sehrawat S, Aird WC, Mayadas TN, Luscinskas FW, García-Cardeña G (2013) Functional vascular endothelium derived from human induced pluripotent stem cells. Stem Cell Rep 1(2):105–113, http://dx.doi.org/10.1016/j.stemcr.2013.06.007CrossRef
Alfaro MP, Vincent A, Saraswati S, Thorne CA, Hong CC, Lee E, Young PP (2010) sFRP2 suppression of bone morphogenic protein (BMP) and Wnt signaling mediates mesenchymal stem cell (MSC) self-renewal promoting engraftment and myocardial repair. J Biol Chem 285(46):35645–35653. doi:10.1074/jbc.M110.135335 CrossRefPubMedCentralPubMed
Atkinson C, Stewart S, Upton PD, Machado R, Thomson JR, Trembath RC, Morrell NW (2002) Primary pulmonary hypertension is associated with reduced pulmonary vascular expression of type II bone morphogenetic protein receptor. Circulation 105(14):1672–1678CrossRefPubMed
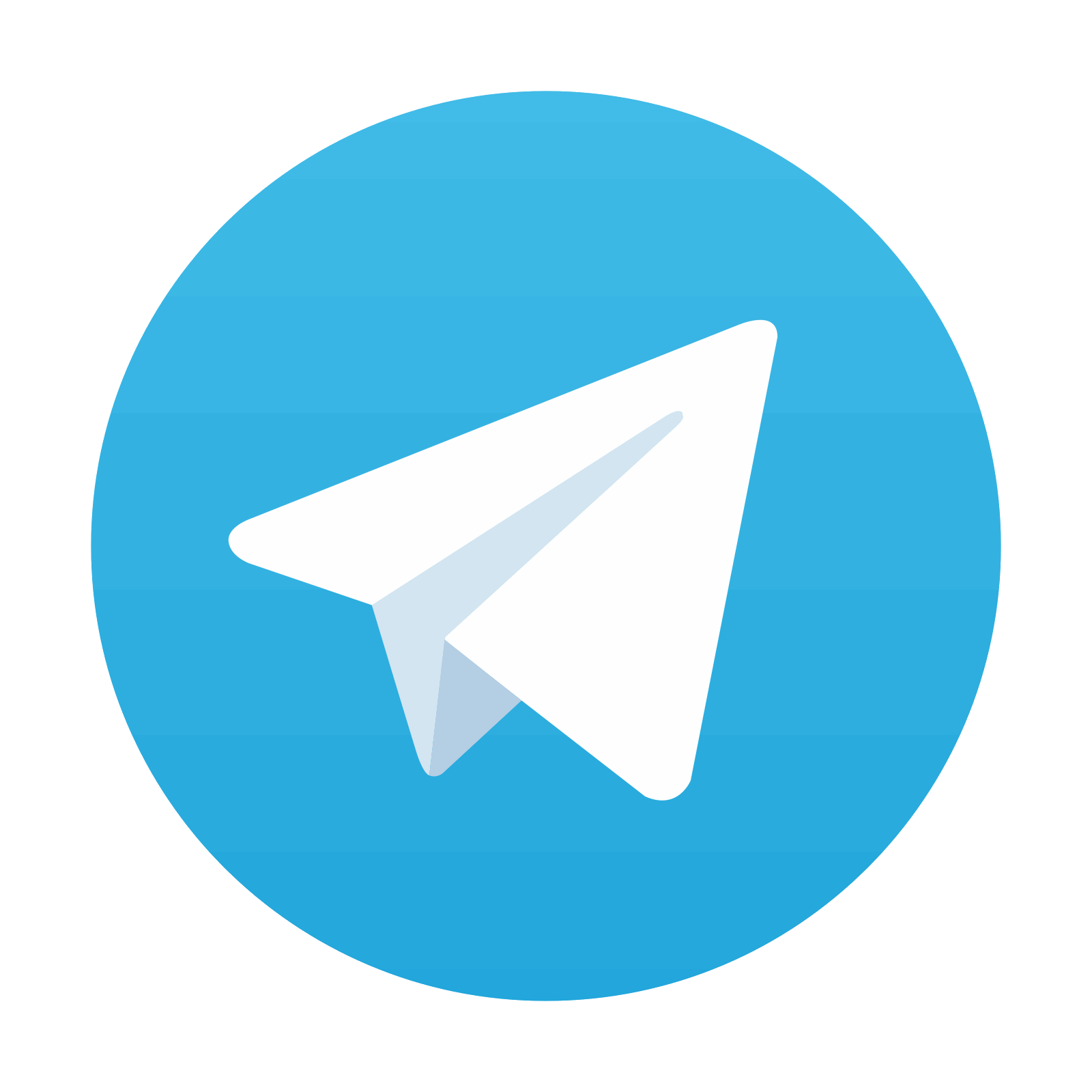
Stay updated, free articles. Join our Telegram channel

Full access? Get Clinical Tree
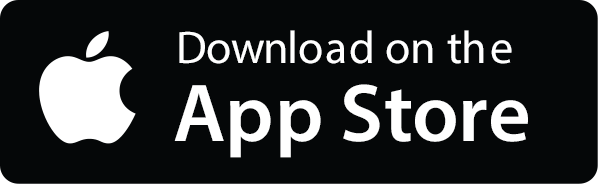
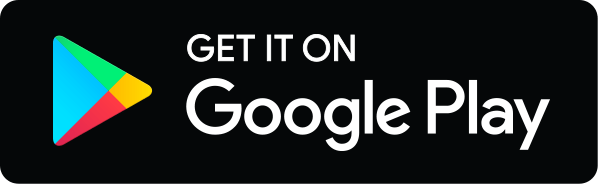