(1)
Anatomy Department, University of Medicine and Pharmacy Carol Davila, Bucharest, Romania
Abstract
This chapter presents aspects of the epicardium and endocardium, the microscopic anatomy and infrastructure of myocardium, the structure of myocardium regarding the atrial and ventricular territories, the fibrous frame of the heart and the excito-conductor system.
This chapter presents aspects of the epicardium and endocardium, the microscopic anatomy and infrastructure of myocardium, the structure of myocardium regarding the atrial and ventricular territories, the fibrous frame of the heart and the excito-conductor system.
7.1 Epicardium and Endocardium
The epicardium is in fact the visceral layer of the serous pericardium. Histologically, it is a mesothelium (simple squamous epithelium, represented by a layer of flat cells, located on top of a basement membrane).
The subepicardial space contains connective adipose tissue (abundant subepicardic adipose deposits may form, especially along the coronary arteries). Precipitating of subepicardial fat can start during the intrauterine period, when small adipose lobules appear along the vessels. In the subepicardial space, nervous fibers can be found along with parasympathetic nervous ganglia, a vascular arterio-venous plexus, as well as lymphatic ganglia and vessels. The epicardium contains numerous free nervous endings (pain receptors).
The endocardium is the follow-up of endothelium, of the great vessels of the heart. The subendocardial space has a similar content to that of the subepicardial space. In addition, in this space, cells pertaining to the excito-conductor system are found, along with subendocardial muscular fibers and loose connective tissue. The atrio-ventricular valves are in fact, endocardial folds that cover both sides of a blade of connective fibrous tissue – lamina fibrosa. As the epicardium, the endocardium is supplied by numerous sensitive fibers.
! Lesions of the endocardium, but especially the epicardium, are responsible for cardiac pain, due to the profuse sensitive innervation of these structures.
7.2 Myocardium: Microscopic Infrastructure and Microanatomy
The term myocardium generally refers to the muscular part of the heart. The myocardium is in fact a generic term that describes a greater complexity of notions.
In the structure of the myocardium the following components can be found: proper cardiomyocytes (“working cardiomyocytes), excito-conductor myocytes, myoendocrine myocytes, the connective fibrous matrix (extracellular matrix), arteries, veins, capillaries, lymphatic vessels, nerves, interstitial cells (of Cajal). In order to be more accurate, the extracellular liquid and the migrated haematic cells must be taken under consideration. This extracellular contains mucopolysaccharides, which maintain the cellular adhesion but in the same time operate as a lubricant, allowing and favouring the gliding of structures.
7.2.1 Cardiac Myocyte: General Features and Microscopic Infrastructure
The strictly muscular component of the myocardium is represented by the cardiac myocyte. There are three types of cardiac myocytes: proper cardiac myocyte (“working” cardiomyocyte), excito-conductor myocytes and myoendocrine cardiomyocyte.
The proper cardiac myocyte is a striped muscular cardiac cell. The general aspect is relatively cylindrical and branched. Its diameter varies from 20 to 30 μm while the length is around 100–150 μm. It forms junctions with roughly 11 other adjacent myocytes and relates to a capillary (Figs. 7.1 and 7.2).
The cardiac myocyte is formed of a sarcolemma (cellular membrane), a sarcoplasm (cytoplasm) and usually a single nucleus located in the center of the cell (different from the skeletal muscular cells which have the nuclei situated at the periphery). As main differences of the cardiac myocyte from the striated skeletal muscular cell, the following should be noted: the absence of neuromuscular junctions, the increased number of mitochondrias and the presence of numerous glycogen granules in the cytoplasm.
The myocardium is a muscular tissue that does not regenerate. Its tolerance limit in acute ischemia is about 20 min.
The observation regarding its regenerating capacity has to be considered a postulate. Its validity may however become relative in time. For example, the presence of cardiac myocytes with two nuclei might be interpreted as a sign of regeneration (Fig. 7.7).
As it has already been described, one can observe that, from an anatomic point of view, the myocardium is not a cellular syncytium (a syncytium is a cytoplasmatic mass with many nuclei, and no intercellular limits), because the myocyte has a clear individuality. Classically, the myocardium is considered a functional syncytium [1].
This notion is not appropriate due to the fact that the cardiac myocytes are connected by junctions, in which the speed of the electric impulse is modified (even if favorable). Microscopically, the nature of conducting the electric impulse is discontinuing, which proves that in reality the myocardium is not a functional syncytium.
However the notion of functional syncytium is still used to express that when a cell is excited, all the cells in the network will suffer the effect.
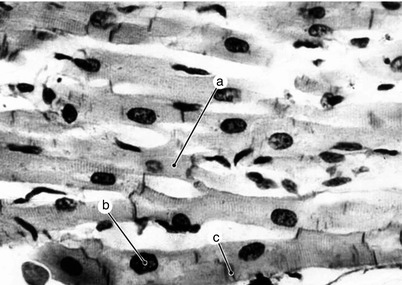
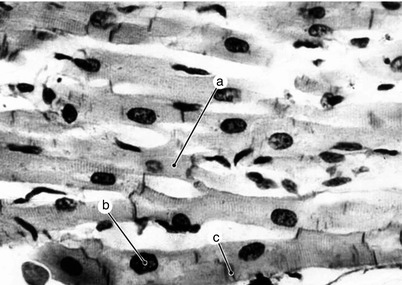
Fig. 7.1
Working myocardium. Electron microscopy (Professor’s Nicolae Ionescu collection) a cardiomyocytes with ramifications, nucleus, c intercalary discs
The structural components of the proper cardiac myocyte (“working” cardiomyocyte) are next to being discussed.
7.2.1.1 Basement Membrane
The basement membrane is a laminar structure which can be observed with the aid of an electron microscope. It covers at the exterior, the cardiac myocyte. It is formed mainly of type IV collagen, proteoglycans and glycoproteins (laminine and fibronectine). It participates in the cellular interchange, but it also interacts with the extracellular matrix, forming an insertion point for the extracellular collagen. In this way, the basement membrane depends on the intercellular cohesion.
The basement membrane is considered a border structure between the components of the extracellular matrix and the components of the myocyte.
7.2.1.2 Sarcolemma
The sarcolemma is the actual cellular membrane, bonded to the internal aspect of the basement membrane. Like any other cellular membrane, it has two lipid layers and contains proteins called integrines. These proteins connect the trans-membrane collagen from the extracellular matrix to the intracellular cytoskeleton. As their name implies, the integrines play a major role in transforming the cellular contraction into an ejection force.
The sarcolemma is involved in the process of forming the intercalated discs and the “T” tubules.
The Intercalary discs (Fig. 7.3) are specialized junctions between myocytes. Their role is to confer endurance, assuring the mechanic cohesion of the myocytes, but they also interfere in conducting the electric impulse. Following electron microscope examination, the junctions are: desmosomes, zonula adherens and gap junctions (communicating junctions).
The desmosomes obtain cellular adhesion with intermediate filaments of desmin.
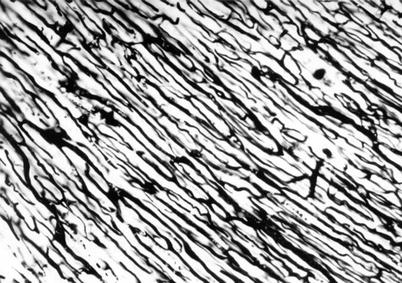
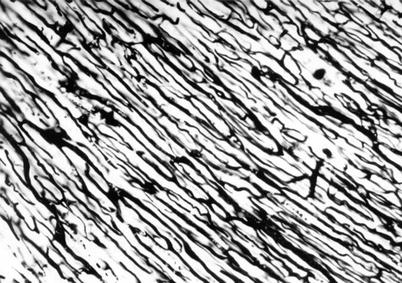
Fig. 7.2
Microscopic aspect of intra-myocardial vascular network after ink injection (Professor’s Nicolae Ionescu collection)
Zonula adherens determines the mechanic adhesion of the cells. The zonules are link zones between the extremities of the actine filaments pertaining to two adjacent myocytes.
The gap jonctions [2] are the equivalent of electric synapses and allow the electric link between myocytes. The gap junctions contain proteins called conexines, formed from units named conexons.
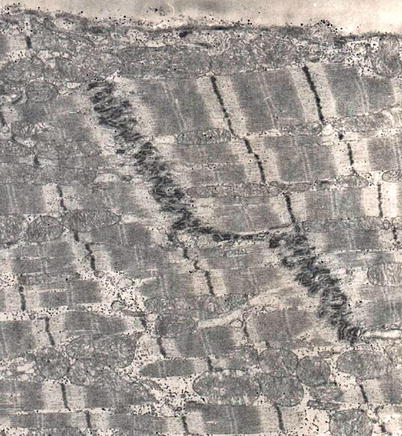
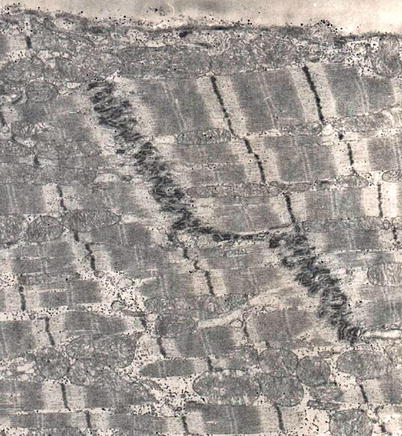
Fig. 7.3
Cardiomyocyte infrastructure. Electron microscopy. Intercalary disc (Professor’s Nicolae Ionescu collection)
The “T” tubules are involved in the excitation – contraction coupling.
The sarcolemma presents on its surface a protein called dystrophin, found in all the types of myocytes in the organism. Its role is to accomplish the adhesion of actine filaments to the laminin of the basement membrane. The synthesis of this protein is affected in a genetic X-linked disease with a recessive transmission, called the Duchenne progressive myopathy. This disease leads to a slow degeneration of the muscular fibers and death around the age of 20.
7.2.1.3 Sarcoplasm
The sarcoplasm has a striped aspect, due to the sequence of segments called sarcomeres. Following electron microscope examination, a sarcomere is bounded by two Z membranes (dark vertical bands). On each side of the Z membrane a clear band is located (the zone where the actine filaments attach on to the Z membrane; it is an isotropic zone in polarised light microscopy). Between two clear bands one can find a dark band (anisotropic zone in polarised light microscopy). At this level, the thin myo-filaments (actine, tropomyosin and the troponin complex: troponin T, I and C) overlap, connect and interact with the thick filaments (myosin).
The M band (which is narrow) is located in the center of the dark band and it is limited on each side by two thin and lighter (in electron microscopic examination) bands, called the H bands.
There are two types of actine: F actine (filamentous) and G actine (globular). The long polymers of F actine result from G actine segments assembling.
Myosin can be decomposed enzymatic. Thus, under the action of trypsin, myosin can be fractionized into light mero-myosin, and heavy mero-myosin. Another enzyme – papain, may fractionize myosin in two sub-fragments, S1 and S2.
The sarcomeres align end to end and form myofibrils. These are grouped in packs just like electric wires in a multifillar cable.
Myofibrils align parallel to the longitudinal axis of the cardiac myocyte and stretch from one end of the cell to the other.
In a transversal section of a sarcomere, the myo-filaments are arranged as a hexagon, following a recurrent pattern, determined by the way the myosin bridges are fixating on actine myo-filaments [3].
From this pattern results the ability of a myocyte to contract only in the direction of the longitudinal axis. Alterations of the short axis are passive or elastic.
The contraction is initiated by the income of calcium in the sarcoplasmic reticulum. Thus, determines the interaction myosin-actin, the hydrolysis of ATP and the energy release with the alteration in the angle of myosin bridges. The modification in this angle leads to the overlapping of the myosin microfilaments with the actine ones and therefore to the shortening of the sarcomere.
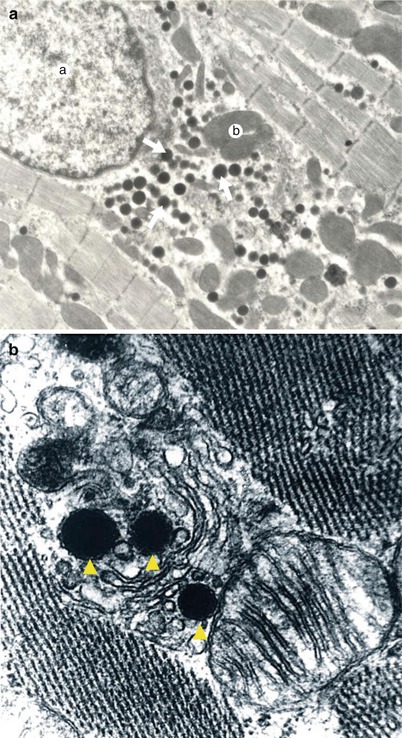
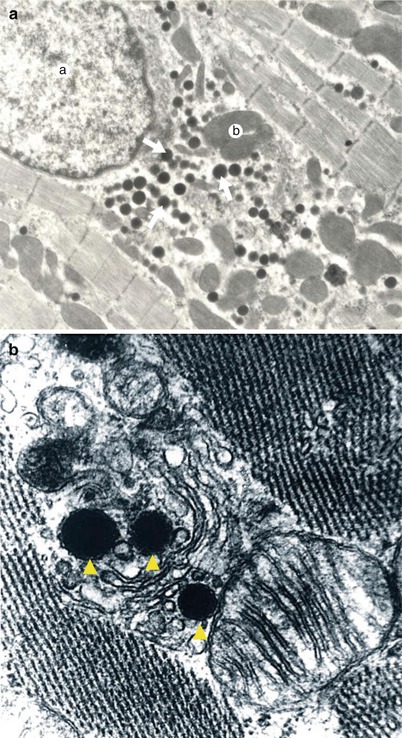
Fig. 7.4
(a) Cardiomyocytic infrastructure. In the cardiomyocytic cytoplasm, near the nucleus, the arrowheads indicate vesicles containing natriuretic peptide. Electron microscopy (Professor’s Nicolae Ionescu collection) a nucleus, b mitochondria. (b) Vesicles containing natriuretic peptide near the rough endoplasmic reticulum. Electron microscopy (Professor’s Nicolae Ionescu collection)
The packs of myofibrils (structured in sarcomeres formed of myo-filaments) constitute a cardiac myocyte. The cardiac myocyte presents a basement membrane and a sarcolemma. Packs of cardiac myocytes, conjoined by collagen bundles of the extracellular matrix, form a muscular fiber covered by endomysium. The endomysium covers each cell and connects the muscular cells with the capillaries. Several muscular fibers form a muscular bundle covered by perimysium. Several bundles form a lamella, covered by epimysium. In areas where the striped structure is not obvious, several bundles form trabeculae, a papillary muscle or a larger bundle, covered by epimysium.
The epimysium (of the lamellae) merges inside the heart (covering the cavities), but it also fuses externally (it covers the subepicardic region of the heart and it connects the lamellae).
7.2.1.4 Cytoskeleton
The cytoskeleton connects the adjacent sarcomeres at the level of the Z membranes. Structurally, it is composed of microtubules and intermediate filaments of desmine, with the thickness of about 10 nm. Titine (conectin) is another protein of the cytoskeleton with role in maintaining the alignment of the sarcomere and preventing an over-stretch and elastic rebound. Titine connects the M bands with the Z membranes [4]. The cytoskeleton also contains alfa actinin, tubulin and talin. The presence of proteins of the cytoskeleton integrates the extracellular matrix and also influences the mechanic performance of the cardiac myocyte.
! Atrial cardiac myocytes contain in the sarcoplasm vesicles that stock atrial natriuretic peptide (Figs. 7.4 and 7.5).
The proteins of the cardiac-myocyte can be identified by imunohistochemical analysis.
7.2.2 Extracellular Matrix: Definition, Components, Structure
7.2.2.1 7.2.2.1 The Definition and General Features of Extracellular Matrix
Inside the myocardium, the determined functional contractile part, named parenchyma, is represented by three types of cardiac myocytes (proper cardiac myocytes, excito conductor myocytes, secretory myocytes). The structure which supports and connects them, named stroma, is represented by the extracellular matrix (it is not a parenchymal cellular component) (Figs. 7.7, 7.8 and 7.9).
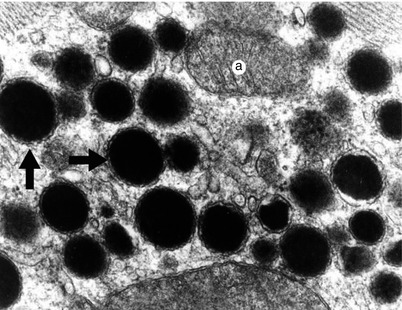
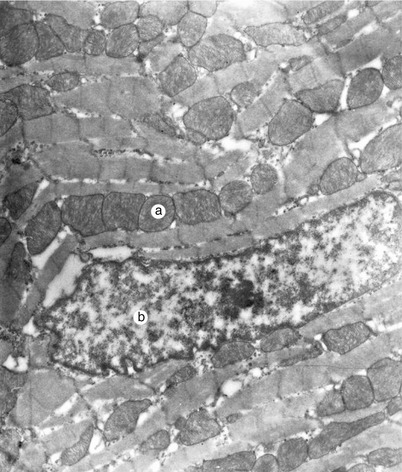
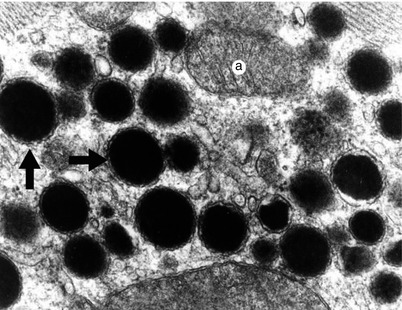
Fig. 7.5
Detail of vesicles containing natriuretic peptide. Electron microscopy (Professor’s Nicolae Ionescu collection) a mitochondria
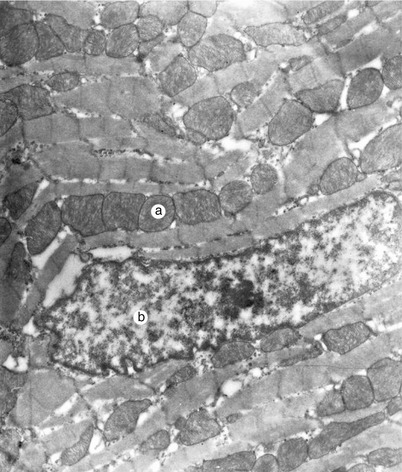
Fig. 7.6
Cardiomyocyte. Mithochondial chains between the myofiber bundles near the nucleus, proof of intense energy generating activity. Electron microscopy (Professor’s Nicolae Ionescu collection) a mitochondria, b nucleus
The extracellular matrix, also called the fibrous matrix, is composed of fibrous connective tissue, vessels, nerves, lymph vessels, migrated haematic cells and Cajal’s interstitial cells.
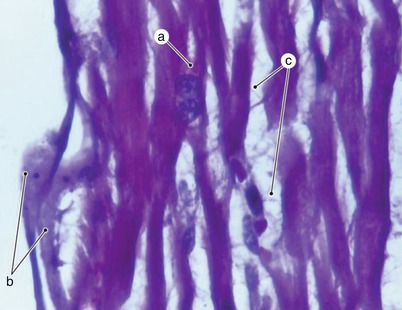
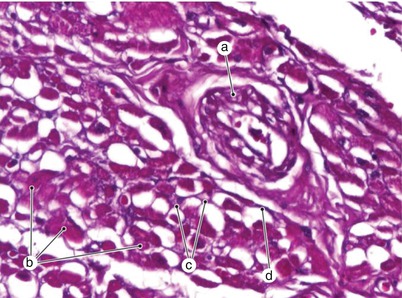
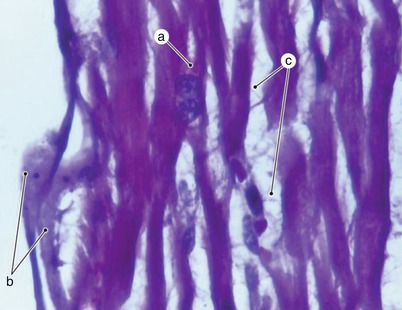
Fig. 7.7
Working myocardium. Optical microscopy. The image captures two extremely rare to objectify facts: a binuclear muscular fiber and, especially, the presence of some intramyocardial neurons in direct junction with muscular fibers. a binuclear muscular fiber, b neurons in direct junction with muscular fibers, c extracellular matrix
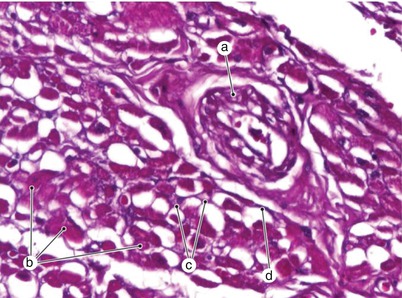
Fig. 7.8
Atrial myocardium. Highlighting some components of the extracellular matrix. a Arteriole, b myocytes, c fibroblasts, d epimisium
The cells of the fibrous matrix constitute 60 % of the entire number of cells of the heart.
Adjacently to its mechanic role to confer the shape of the organ, the resistance to contortion, the resistance to elongation, support, alignment and connection of myocytes, an important role of the extracellular matrix is to intercede the transfer of forces resulted during the contraction and to convert them into ejection systolic force.
The re-elongation (drawback to initial length) of myocytes during the diastole is also partially determined by the extracellular fibrous matrix [5].
The extracellular matrix can be partially defined as the reunion of the three fascial connective structures of the myocardium – endomisium, perimisium, epimisium.
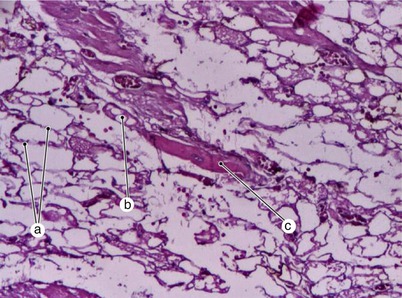
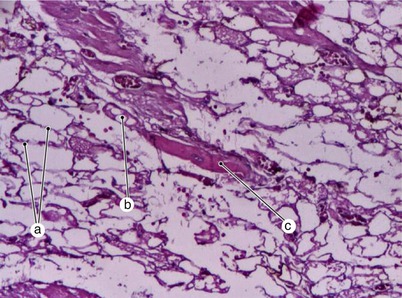
Fig. 7.9
Subepicardial necrosis in the atrial teritory allows us to observe an unaffected cardiomyocyte and episium sheets remaining after the myocyte dies. a epimisium, b myocyte necrosis allows episium visibility, c cardiomyocyte with ramifications
Endomisium binds the myocytytes and connects them with the nutritive vessels. Some authors consider that the cuspids and the tendinous cords are exterior structures of the extracellular matrix [6].
Fibroblasts (which can develop into fibrocysts), miofibroblasts (a phenotypic variety of fibroblasts), connective fibers (collagen, reticular and elastic fibers) and intercellular substance form the fibrous connective tissue of the extracellular matrix.
7.2.2.2 7.2.2.2 The Types of Connective Cells of the Extracellular Matrix
The following section reviews the types of connective cells of the extracellular matrix.
Fibroblasts [7] can develop into fibrocytes. These cells produce vinmentine – a protean intermediate filament, which is also found in other cells, such as the smooth muscle, endothelial cells or lymphocytes. These cells have the capacity of tissue movement because they can extend cytoplasmatic prolongations called lamelipodes.
Miofibroblasts are a phenotypic variety of fibroblasts. They produce actinic filaments which have contractile properties. It is a paradox that although these cells are genetically fibroblasts, the phenotype appears to be of a myocyte.
Tissular macrophages (histiocytes) have the capacity of tissue movement and phagocytosis. They are involved in the local immune mechanisms.
7.2.2.3 7.2.2.3 The Group of Migrated Haematic Cells of the Extracellular Matrix
The passage of the blood cells is accomplished by diapedesis. These cells and the endothelial cells display on their membrane specific proteins – selectins, which determine the adhesion of leukocytes to the wall of vessels.
The cells of this group are:
mast cells contain numerous deposits of heparin and histamin
plasmocytes are antibody-secreting cells
monocytes can develop into macrophages
lymphocytes are involved in the specific immune mechanisms
eosinophil cells have the capacity of phagocytosis
7.2.2.4 7.2.2.4 The Types of Fibers of the Connective Tissue of the Extracellular Matrix
The collagen fibers – they have a protean structure, resulting from the junction of smaller segments, called tropo-collagen. The protean structure is abundant in glycine (30 %), prolyne, hidroxyprolyne, lysine and hidroxy-lysine.
The reticular fibers have a protean structure, which is mainly made of type III collagen that anchors on basement membranes.
The elastic fibers are compound of glyco-proteins, with a high concentration of glycine and prolyne.
7.2.2.5 7.2.2.5 Basement Membrane
It is a structure of the extracellular matrix, compounded of type IV collagen fibers displayed in a network-like architecture, located on the external aspect of the sarcolemma. It is involved in maintaining the cellular cohesion, while in the same time it is an anti-diffusion barrier for glucose and oxygen.
The elements which build-up the basement membrane are: type IV collagen, fibrillin [8], laminine and fibronectine. Among these components, the non-collagen glyco-proteins, laminine and fibronectine play an important role in the cellular adhesion and interaction.
7.2.2.6 7.2.2.6 The Intercellular Substance and Its Components
The intercellular substance (amorphous) is assembled from the following:
Proteoglycans. They are heavy weight substances, characterized by the presence of a protean axis on which lateral chains of sulfated glycosaminoglycans, anchor. Proteoglycans are linked to hyaluronic acids.
The proteoglycans associated to the basement membrane are: heparan sulfate proteoglycans and syndecan.
The proteoglycans associated to the intercellular substance are: chondroitin sulfate, heparan sulfate, dermatan sulfate.
Glycosaminoglycans are polymers of disaccharide acids, which contain amine sucrose.
Glyco-proteins for cellular adhesion
Fibronectine is a dimeric glyco-protein which can be fibrillar (in the extracellular matrix) or soluble (in the plasma). It may form connections with the proteoglycans, integrines and collagen.
Laminine is a glycoprotein, of the basement membrane. It may link with proteoglycans, integrines and entactines.
7.2.2.7 7.2.2.7 The Cellular Receptors of the Extracellular Matrix and the Metalloproteinases
The cellular receptors of the matrix are:
Integrine is in fact, a group of trans-membrane proteins, formed of alfa and beta units. They link to the cellular adhesion molecules of the matrix, as well as to the ones of the cytoskeleton.
Syndecans are a subtype of trans-membrane proteoglycans. The protean core of these proteins perforates the sarcolemma. The external extremity connects with heparan sulfate. The internal extremity (cytoplasmatic) connects with the cytoskeleton.
The matrix metalloproteinases [9]
This is a family of enzymes which degrades (reshapes) the proteins of the extracellular matrix. They have a specific bonding situs for zinc. They are at balance with inhibitors of metalloproteinases.
Examples of matrix metalloproteinases: stromelysin which degrades laminine, fibronectine and proteoglycans. The collagenase degrades the type I and type III collagen. The gelatinase degrades elastine and type IV collagen.
7.2.2.8 7.2.2.8 The Types of Collagen of the Extracellular Matrix
The type I and type III collagen are the main types of collagen in the structure of the extracellular matrix. The resistance to elongation of the type I collagen is approximate equal to that of steel. As a repercussion, having remarkable mechanic properties, the matrix is considered to be a determinant factor in maintaining the shape and diastolic rigidity [10,11].
Eighty-five percent of the total collagen of the extracellular matrix is represented by fibrillar type I collagen [12]. This is synthesized by fibroblasts and myo-fibroblasts. The rest of the percentage is filled by fibrillar type III and type V collagen and type IV and type VI collagen, of the basement membrane.
Collagen is a protein with a slow turnover, of about 80–120 days. This slow turnover in a healthy heart is replaced with a very fast turnover in certain pathological situations, when aggregation of collagen leads to fibrosis. These pathologic conditions lead to a new type of phenotypic fibroblast called, myo-fibroblast, which is fibrogenic.
! In morphologic terms, aggregations of collagen may be reactive interstitial fibrosis (the perimisium component enlarges) or a repair fibrosis – replacement fibrosis (appearance of scars which replaces the dead cells).
A fact to be taken into consideration is that because apoptosis (“cellular suicide”) is not being accompanied by inflammation, it is not followed by fibrosis.
It is now well known that not only lesions of the contractile system may lead to heart failure, but also lesions of the extracellular matrix!
7.2.2.9 7.2.2.9 Examples of Pathological Situations in Which the Extracellular Matrix Is Incriminated
The role of the extracellular matrix and its importance can very well be underlined, presenting a few pathological conditions in which lesions of the matrix have important reperccusions [13].
Fibrosis with massive growth in type I and type II collagen
At a certain expansion level of fibrosis, the rigidity of myocardium increases and may lead to diastolic heart failure – heart failure with preserved ejection rate. This condition is frequent in patients with concentric cardiac hypertrophy which accompanies arterial hypertension.
Alteration of fibrillar collagen
If the collagen is highly and massively degraded, as for example in idiopathic dilated cardiomyopathy, the destruction of fibrillar collagen leads to excessive gliding of muscular fibers which determines rarefaction of myocardium and dilated ventricles. The heart becomes globular and systolic heart failure appears, with decreased ejection rate.
Monitoring of the serologic markers linked to synthesis and degradation of collagen may be an important part of a follow up strategy in the evolution of these diseases.
We would like to underline the fact that, in the myocardic mass, besides the possibility of variation in parameters of the extracellular matrix, the structure and the aspect of the cardiomyocyte are themselves dynamic.
Like the extracellular matrix, the dimensions of the muscular cells may also vary under the influence of physiologic or pathologic stimuli (increase defines myocyte hypertrophy).
7.2.3 The Structure of the Myocardium of the Atrium. The Continuous Atrium Theory
The logic of the embryologic evolution from the two atria – structures which develop from a unique cavity – must be found in the distribution of the main muscular bundles pertaining to these cavities.
In other words, on one hand the atria must be reunited through muscular bundles which form a continuous atrium (do not forget that the muscular fiber is the sub-layer of conduction of the nervous impulse). On the other hand, given the complex embryogenesis, atrial muscles have a distribution that may vary. Due to the ontogenetic complexity, a breakage of the continuous atrium appears.
Normal connections between muscles of the right and left atrium are highly important, due to the fact that they allow the transfer of the physiologic electric impulse on a normal pathway from the right atrium towards the left one.
Abnormal connections represent the sub-layer of pathologic electro-physiologic manifestations.
The wall of the primitive atrium is composed of an endocardic layer, with a membranous aspect, which will develop into myocardium.
The continuous atrium initially is altered and becomes non homogenous due to a few elements responsible for generating heterogeneity of the adult atrial wall:
embedment of segments of the atrio-ventricular junctions into the wall of atrium
embedment of extensions of the endocardial cushions into the wall of atrium
embedment of extracardial cellular populations into the wall of atrium
embedment of the venous sinus into the wall of right atrium
embedment of the primitive pulmonary vein into the wall of left atrium
the development of septa and different venous ostia.
The physiologic heterogeneity of the atrial architecture has as a main effect – abruption of the continuous atrium and therefore avoids a circular pathway of an electric impulse and a possible re-entry mechanism!
The wall of left atrium is generally thicker than the wall of right atrium, which reaches laminar dimension and aspect in the interpectinate spaces.
In fact, the disposal of the atrial muscular bundles follows a few patterns:
Circular, internal and external muscular bundles are present at the base of the atria, in the vestibular territory. The external bundles connect with the ones on the opposite side, while the internal bundles connect with the septal muscles.
Around all venous ostia detour bundles are present, on each side of the ostium, which emerge from a main bundle with a route towards the ostia. These bundles fuse with circular bundles at the base of the atria.
The base of the auricles is surrounded following the same pattern by two detour bundles, which afterwards unite.
Muscular interatrial bundles are also present.
In each atrium one can find intraatrial bundles.
7.2.3.1 7.2.3.1 The Muscular Bundles of The Right Atrium
In the structure of the right atrium, several bundles have been described:
The vestibular muscles of the right atrium are represented by two circular vestibular bundles, one internal and one external. These bundles surround a large area of the base of the atrium.
The internal circular vestibular bundle is better structured anteriorly, while posteriorly, it fades in the atrial wall of the atrio-ventricular crease, at the level of the terminal crest.
The external circular vestibular bundle of the right atrium is the right continuity, below the right auricle, of Bachmann’s interatrial bundle (look forward) (Figs. 7.10 and 7.11). It is less structured than it, and after it reaches the acute margin, due to the crimped atrio-ventricular junction, it becomes profound and fades posteriorly in the vestibule of the atrium, at the limit with the terminal crest. This bundle is continued by a “delicate” superficial branch called the external bundle of right atrium (Figs. 4.36 and 6.10).
The external bundle is not always well structured, but when it exists, it consists of thin muscular fibers which connect the epicardium with the pectinate muscles. A part of these fibers form subepicardic loops (in the subepicardic space, they connect the deep bundles). The external bundle of the right atrium ends at the level of the terminal groove. At times, it may continue towards the posterior with the intercavum bundle (non-constant) which lays between the terminal groove and the interatrial groove of Waterstone. The intercavum bundle may be an accessory route of interatrial transmission (Figs. 7.12 and 7.13).
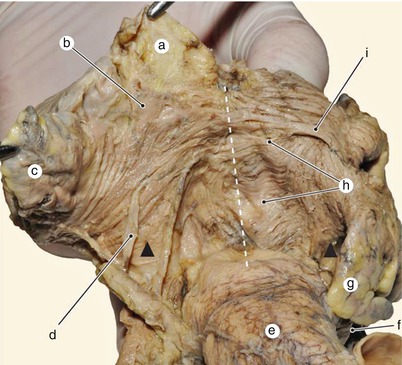
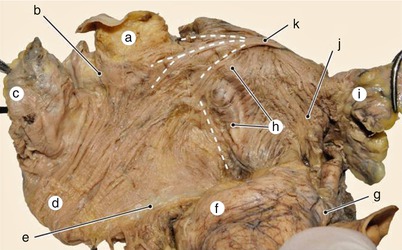
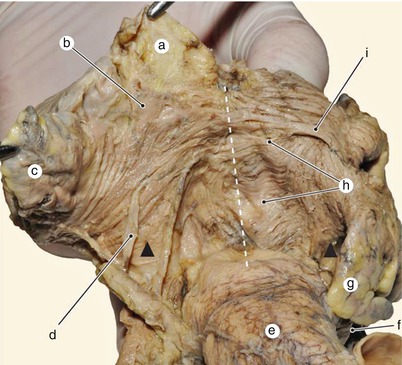
Fig. 7.10
Distribution of muscle fascicles from the anterior-superior face of atria. Dotted line indicates the interatrial boundary. Arrows show vestibular bundles. a superior vena cava, b area of sinoatrial node, c right auricle, d sinoatrial node artery, e aorta, f left coronary artery, g left auricle, h interatrial notch fascicle, i Bachmann fascicle
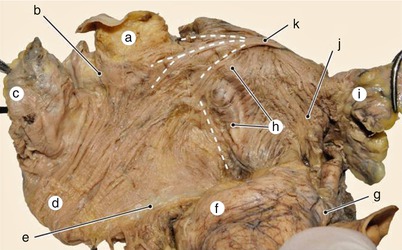
Fig. 7.11
Detail of previous image, with highlighting of the roots of the Bachmann fascicle. a superior vena cava, b area of sinoatrial node, c right auricle, d Anterior vestibular fascicle, e atrial-ventricular junction, f aorta, g left coronary artery, h interatrial notch fascicle, i left auricle, j Anterior vestibular fascicle, k Bachmann fascicle with its three originary roots: atrial, one anterior and one posterior to the vena cava
Both the intercavum and the external bundles are frequently under-developed and cannot be individualized.
The pectinate muscles emerge from the terminal crest and fade into the vestibule, almost right-angled on the internal circular bundle. There is a group of pectinate muscles with an opposite direction (however see the cavity of right atrium).
The muscular bundle of the terminal crest divides towards inferior, at the level of the cavo-tricuspide isthmus into two bundles:
The inferior bundle fades into the floor of atrium; its fibers reach the triangle of Koch.
The superior bundle fades into the structure of the Eustachian margin (it is a variable structure). Its fibers may reach the triangle of Koch.
The (semi)circular bundle of fossa ovalis is also known as the greater limbic bundle. It connects with other septal bundles (Fig. 6.25).
As far as the interatrial septum is concerned, it must be noted that the superior and posterior part of septum secundum is in fact a crease of the atrial wall. As consequence, it is but logic that the superficial bundles continue their pathway in the thickness of the interatrial septum (to follow the configuration of the crease and connect with the opposite side).
The bundles of the dorsosinusal space have been discussed in the dedicated chapter.
7.2.3.2 7.2.3.2 The Muscular Bundles of Left Atrium
In the structure of the left atrium, one can find: the septal-atrial bundle, which emerges from the anterior and inferior part of the septum and fades into the anterior wall of the atrium, corresponding to the aorto-mitral continuity (on which it also partially inserts). It is possible that this muscular bundle develops from the left extension of the superior endocardial cushion (Fig. 2.9).
The opposite of the previous is a muscular bundle that consolidates the base of the interatrial septum on the left side (developed from the extensions of the inferior endocardial cushion) (Fig. 2.16).
The external circular vestibular bundle of the left atrium surrounds the base of the left atrium and it fades posterior, near the interatrial groove. In fact, all the other bundles of the left atrium fuse in with this one (look forward) (Figs. 7.14 and 7.15).
The inter-pulmonary bundle descends in the space between the right and left ostia of the pulmonary veins and it ends in the angle between the coronary sinus and the interatrial groove, where they may o may not fuse with the external circular vestibular bundle (Figs. 4.39 and 7.15).
Fig. 7.12
Heart with a very well represented intervenous fascicle. Posterior view. a superior vena cava, b interatrial sulcus, c inferior vena cava, d vestibular fascicle, e intervenous fascicle, f terminal sulcus
Fig. 7.13
Detail of previous image. Notice the existence of a thin vertical fascicle, which seems to bypass the intervenous fascicle fibers. a superior vena cava, b interatrial sulcus, c inferior vena cava, d external circular vestibular fascicle, e intervenous fascicle, f terminal sulcus
Fig. 7.14
Postero-lateral segment of the vestibular fascicle seen from the pulmonary surface of the left atrium. a left pulmonary veins, b vestibular fascicle, c left auricle, d Circumflex artery, e limit of coronary sinus, f inter-pulmonary fascicle
Fig. 7.15
Posterior aspect of the left atrium. Inter-pulmonary and vestibular fascicles end near the inter-atrial sulcus. Thin muscular periinusal fibers continue with the vestibular ones (arrow). a inferior left pulmonary vein, b vestibular fascicle, c venous sinus, d inter-atrial sulcus, e inter-pulmonary fascicle
It may very well be observed anterior and to the left of the orifice of superior vena cava (Figs. 7.10, 7.17 and 7.18).
It has a transversal disposal, crossing over like a bridge to the other side of the atria. The right extremity originates in two roots (superior and inferior), on each side of the orifice of superior vena cava. Sometimes, three roots may fuse in order to form this extremity.
At the limit between the atria, fibers from this bundle have a deep trajectory into the septum. These fibers have this direction because the atrial wall tends to crease itself at this level in order to form the limb of the fossa ovalis.
In its trajectory towards left, the bundle of Bachmann crosses the interatrial border and fades either at the base of the left auricule or at the level of the orifice of left superior pulmonary vein. In each situation, it divides into a superior and inferior bundle. These bundles circle the left auricle or the pulmonary vein and connect with the external circular vestibular bundle of the left atrium, at the base of the atrium. This external circular bundle fades posterior, at the base of the heart, in the inferior part of the interatrial groove or near it.
As previously discussed, in its trajectory towards right, the bundle of Bachmann originates in two roots.
The inferior root continues not only the pathway of the external circular vestibular bundle of the right atrium but also a group of muscular fibers that originate in a region near the sinoatrial node. It is possible that along this pathway the electric impulse generated in the sinoatrial node reaches the left atrium.
The superior root continues the pathway of the inter-cave muscular bundle (Fig. 7.11).
As previously demonstrated, around fossa ovalis, in the thickness of the limb, one can find a very well structured muscular bundle, called the limbic (semicircular) muscular bundle. This bundle has a relative interruption in the posterior and inferior region fossa ovalis.
At the base of the atria, anterior, on the trajectory of the former interatrial notch of the primitive atrium, there is a fine layer of muscular fibers that connects the two atria. The muscular layer continues towards left the external vestibular bundle of the right atrium and it could be named the bundle of the interatrial notch (Figs. 7.11 and 7.17). This bundle unites towards inferior with the circular vestibular bundle of the left atrium, while its superior part descends as the inter-pulmonary bundle. Having this conformation, we consider that this bundle must also be remarked as an interatrial transmission pathway!
7.2.3.3 7.2.3.3 The Conveyance of Electric Impulse Through the Muscular Continuous Atrium
For an assembly preview, we will shortly discuss the possible trajectories of the electric impulse generated in the sinoatrial node towards the two atria, following the muscular continuous atrium.
There are two areas from which the nervous impulse can depart towards the walls of atria, due to the fact that the excito-conductor system extends into the terminal groove:
1.
The superior area of the terminal groove – the inferior root of the bundle of Bachmann and then the main bundle, with two possibilities:
(a)
profound, towards the interatrial septum (and possibly towards the atrioventricular node)
(b)
towards the left atrium, through the peri-venous bundles and the peri-auricular bundles, which fuse with the external circular bundle of the left atrium. This bundle fades near the interatrial groove.
In the space between the ostia of the pulmonary veins, the muscular bundles fuse and form a thin layer (which is sometimes incomplete) of muscular fibers, which might be named pulmonary inter-venous bundle (Fig. 7.15). As already discussed, this bundle continues the bundle of the interatrial notch.
The eventual muscular inbreaking of the terminal part of the pulmonary veins, forms a peri-venous circular muscular route that might alter the local electrophysiology.
2.
The median and inferior area of the terminal groove may represent another zone from which the electric impulse generated in the sinoatrial node is launched on the following trajectory: pectinate muscles – vestibular bundles of the right atrium – bundle of the interatrial notch – external circular bundle of the left atrium (or sometimes inter-pulmonary bundle) – posterior interatrial groove.
! As discussed, the area represented by the sinus venosus and located between the terminal and interatrial grooves, interrupts the muscular continuous atrium. Sometimes at this level, one can find the inter-cave bundle which may vary or alter the atrial electrophysiology.
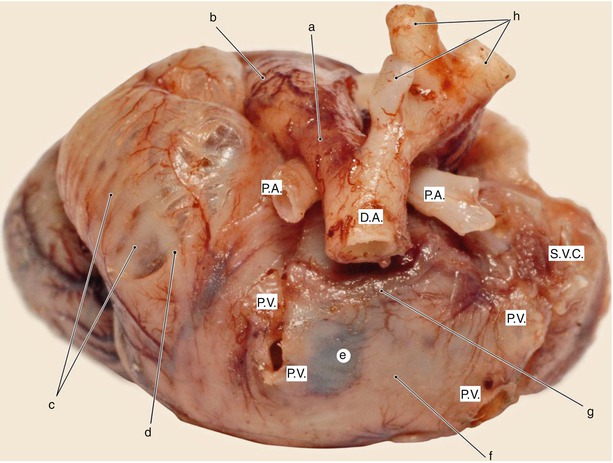
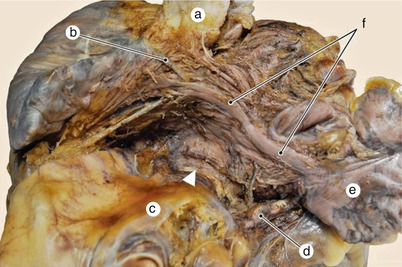
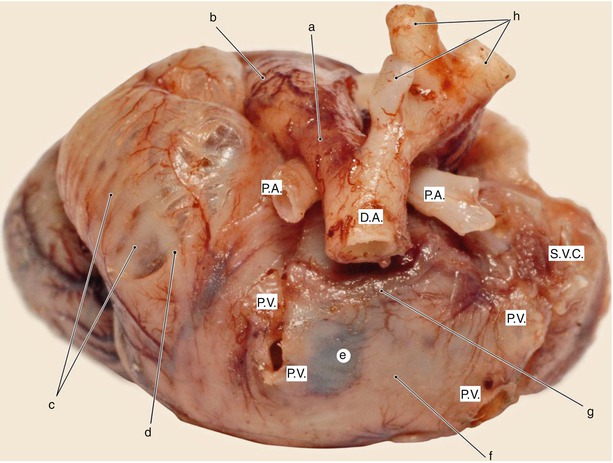
Fig. 7.16
Fetal heart – 5 months old. Posterior view. Myocardic formation process of the atrial walls is captured in evolution. Notice the left auricle muscularisation pattern in circular strips. The image captures remarkably the anatomical relation between the arterial canal, descending aorta (DA) and the pulmonary arteries (PA). a arterial canal, b pulmonary trunk, c left auricle muscularisation pattern in circular strips, d sagittal muscularisation strip, e low muscularisation area, f inter-pulmonary fascicle, g forming area of the Bachmann fascicle, h branches from aortic arch, PV pulmonary veins
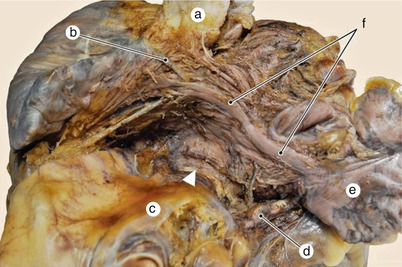
Fig. 7.17
Bachmann fascicle ends divergent at the base of the left auricle. Its root forms a myocardic bridge over the sinoatrial node artery. Inter-atrial notch fascicle (arrow). a superior vena cava, b sinoatrial node artery, c aorta, d left coronary artery, e left auricle, f Bachmann fascicle
7.2.3.4 7.2.3.4 The Variability of Embryologic Myocardic Inbreaking of Atrium Walls – Anisotropic Substratum of Electrophysiology
Due to the complexity of the development of the atrial wall, we consider that the best way to present the atrial myocardium is to relate to its embryologic evolution and the process of muscular inbreaking.
The muscular inbreaking of atria follows a membranous (endocardic) pattern (Fig. 7.19), on multiple variable ways, regarding the territory involved:
The mesenchymal extensions of the endocardial cushions (Figs. 2.4 and 2.9) will form cardio-myocytes. This group is represented by territories derived from the fusion of endocardial cushions, as well as those derived from their extensions: base of the interatrial septum, the territory derived from the former intermediate septum, the anterior region of septum secundum and the anterior and medial parts of the left and right atrial vestibule.
The extra-cardiac mesoderm which invades the membranous part of the septum primum, through the vestibular spine, determines myocardic inbreaking, rendering to the formation of the muscular part of the interatrial septum, mainly the floor of the fossa ovalis (Fig. 2.20).
Regarding the formation and presence of the vessels (conditioned mostly by the evolution of the cells of the proepycardic organ) -the myocardic inbreaking begins either in the territories around the venous ostia, or in the auricles, in mostly circular patterns (Fig. 2.52, 2.54 and 7.16). The bundle of Bachmann develops on the reflection line of pericardium on the atrial wall.
Fig. 7.18
Variant of Bachmann fascicle that ends near the ostium of left superior pulmonary vein. a superior vena cava, b artery of sinoatrial node, c right auricle, d aorta, e left auricle, f Bachmann fascicle, g LPV left pulmonary vein
The myocardic inbreak of segments derived from atrio-ventricular junctions, which are encircled in the atrial vestibule (Fig. 2.80). The remnant bundles derived from the mesenchyme of these regions may suffer a process of muscular inbreak and may represent abnormal pathways of transmission between atria and ventricles (Fig. 6.82).
The myocardic inbreak of the coronary sinus is obviously part of the same process which takes place near by, at the level of the left atrial wall. One can observe that the same vessel supplies simultaneously the adjacent regions of right and left atria, as well as the wall of the sinus venosus (Fig. 7.20).
This process underlines the fact that the coronary sinus is an extension of the right atrium and explains the possibility that remnant muscular bundles may represent abnormal transmission pathways between the two atria, around or through the wall of the sinus venosus.
Normally, due to the involution of the left cornus of the primitive sinus venosus, the muscles of the coronary sinus should have devolved and lost their connection with the right atrium.
The embedment of the right cornus of the sinus venosus in the atrial wall is followed by a heterogeneity increase of the atrial architecture, due to the development of the eustachian margin, the terminal crest, dorsosinusal space and eustachian and thebessian valve.
Electro-physiologically, the embedment of sinus venosus into the wall of atrium and the variability of the myocardic inbreak of structures represent major events which lead to an increased anisotropism of the wall of atrium.
After the myocardic inbreak process has begun in these specific areas, the inbreak zone increases due to local proliferation and these zones tend to fuse, in order to form the atrial muscular layer. Incomplete myocardic inbreak areas may still be sometimes visible in adults (Fig. 7.20).
7.2.4 Myoendocrine Cardiomyocyte – The Heart as an Endocrine Organ
In mammals, the atrial myocyte contains in its cytoplasma a great number of electron-opaque granules [15], with a diameter of 0.3–0.4 μm, associated with structures of the Golgi Complex (Figs. 7.4 and 7.5). Ever since 1956 it has been known that the majority of human atrial myocytes contained the so-called atrial specific granules. Soon a conclusion was reached that these granules result from a secretory function. This is how morphological studies led to a radical change of the image of the heart, which is currently seen not only as a simple central pump but also as an endocrine organ with crucial regulatory functions in the vascular and even the renal system. Cardiomyocytes have four fundamental properties: excitability, conductibility, contraction and secretion. It seems reasonable to conclude that all these functions are inter-linked [16].
As far as secretion is concerned, we can say that there is a group of substances found to be secreted by cardiomyocytes, both the atrial and the ventricular. These substances are peptidic hormones which reveal a structural equivalence but have a distinct genetic determinism. These substances are:
Atrial Natriuretic Peptide (ANP)
Brain Natriuretic Peptide (BNP or GC-B). It is called this way because it was initially discovered in pig brains
Natriuretic Peptide Type C (CNP).
These substances are secreted both by the atrial and the ventricular myocytes. ANP is secreted particularly by the atrial and BNP by the ventricular myocytes. CNP is present in a variety of tissues (paracrine hormone).
The main actions of these substances are: to decrease peripheral vascular resistance, to decrease central venous pressure, to increase natriuresis and diuresis.
BNP is co-secreted together with a terminal fragment of amino acid, biologically inactive, called NT-pro BNP. The period for the division of BNP is longer than that of ANP, and NT-pro BNP has the longest period of division. This fact makes NT-pro BNP to be preferable for blood diagnostic tests. Blood levels of BNP and NT-pro BNP are used to diagnose acute cardiac insufficiency.
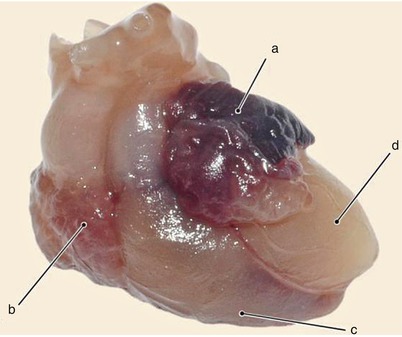
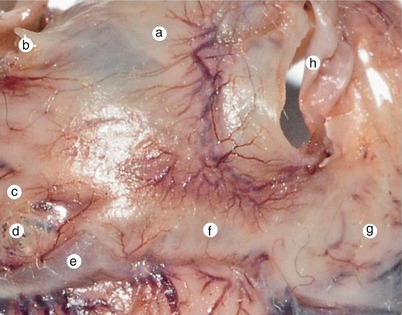
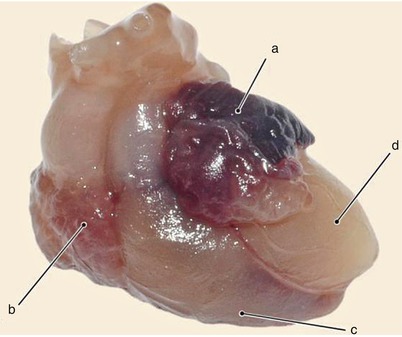
Fig. 7.19
9 weeks old heart. The atrial walls still have a membranous aspect. a left atrium, b right auricle, c left ventricle
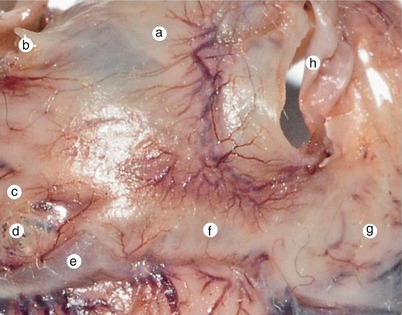
Fig. 7.20
5 months old fetal heart. Posterior view of the cross region of the heart. Detail. The local muscle formation process of the two atria and the sinusal wall is unitary and continuous. The process depends on vascular presence. a intrapulmonary fascicle, b left inferior pulmonary vein, c vestibular fascicle, d loss of compacting of the atrial wall, e great vein, f muscle formation of the sinusal wall, g right atrium, h inferior vena cava
7.2.5 Architecture of the Myocardium-General Considerations. The Theory of “Shutters”
Every concept of microstructure that has been presented represents the basis for the next stage, that of describing the macroscopic structure of the ventricular myocardium. Although it may seem surprising, at the moment, there is no description of the architecture of the myocardium which is sufficiently well-reasoned, thorough and general in order to be unanimously accepted. This is due to the difficulty of the subject as well as to the fact that until now only minimal concepts have been necessary for clinical needs. These minimal concepts can be summed up as follows:
The atrial myocardium is thinner than the ventricular one. It consists of superficial muscle fibers and deep muscle fibers. Superficial fibers make up a system of ansae which bind the two atria. Deep muscle fibers are specific to each atrium.
As far as the organization of the ventricular myocardium is concerned it is generally accepted that there is a superficial layer that passes from one ventricle to the other and which at the top of the heart twists and goes deeper, forming the vortex cordis. There is also a middle muscular layer in each ventricle, well represented particularly in the left ventricle.
There is as well a deep muscular layer specific for each ventricle. This classification cannot by far exhaust the topic of the myocardium architecture.
Once the technical opportunities to explore the heart increased, it was discovered that the current anatomical data do not provide a theoretical framework that should be sufficient for the technical capacities of morphofunctional exploration. This fact re-launched the race to solve “the problem of the myocardium structure”.
As it often happens in research, numerous opinions were put forward, which, surprisingly, were quite often divergent. These opinions vary from the theory of the myocardium structured as a helical band to mathematical models where the fiber orientation is governed by strict formulas.
Beyond some exaggeration, we consider that each study has brought up a specific point of view, so a data basis has been built which helps us come closer to forming a universally valid conclusion. In what follows we will present, with arguments, the results of our observations regarding the myocardium structure.
In the anatomical description we will proceed from the premise that a structural theory is correct if there is a correspondence between organizational and functional details.
7.2.6 The Architecture of the Ventricular Myocardium
First we will go over the summary of the microstructural organization: Packs of myofibrils (structured as sarcomeres consisting of myofilaments) make up a cardiomyocyte. The aspect of the cardiomyocyte is essentially longitudinal, branched and anastomosed. This aspect can be found as a pattern at different organizational levels. The cardiomyocyte has a basal and sarcolemmic membrane. The cardiomyocyte packs, bound by collagen fascicles and varied proteins of the extracellular matrix make up a muscular fiber, surrounded by a connective tissue sheath called endomysium. The endomysium covers every cell as well as the fiber itself and binds muscle cells at the capillary wall. More muscle fibers make up a bundle, surrounded by the perimysium. More bundles make up a muscle lamella [17], surrounded by the epimysium. In the areas where the strand structure is not obvious, more bundles make up a trabeculation, a papillary muscle or a larger bundle, surrounded by the epimysium.
Practically, the epimysium (of the lamellae) fuses both at the interior of the heart (lining cavities subendocardially) and at the exterior (lining the heart subepicardially and binding lamellae).Fibers, bundles and lamellae are essentially branched and anastomosed. The acceptance of the lamellar arrangement is important and (for some) obvious in the myoarchitecture of the left ventricle. In comparison with the long axis of the left ventricle (originating in the primitive ventricle) the muscle lamellae are flat, superposed and circular, the most suggestive comparison being that of a horizontal blind (Fig. 7.21). Consequently, we will call this type of organization “the theory of shutters”.
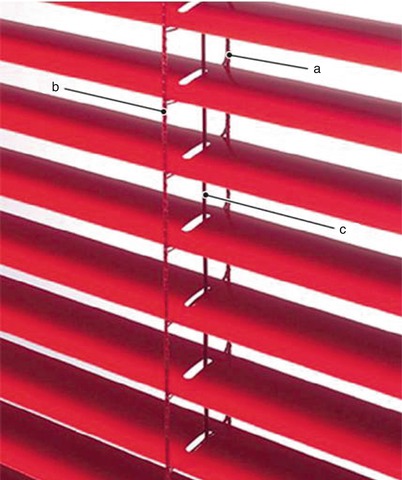
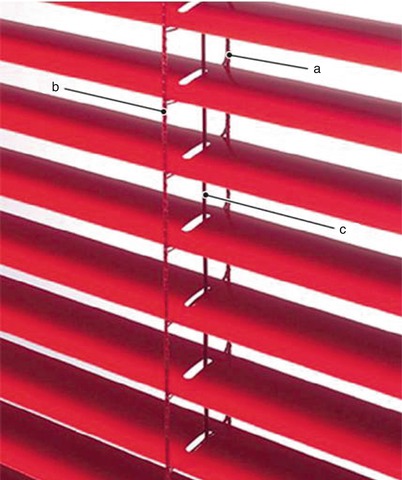
Fig. 7.21
Blind-like structure formation. See the position of the cohesion lines that allow the system to work like a unit. a external cohesion line, b internal cohesion line, c central cohesion line
Towards the top and the basis of the ventricle, the lamellae slant, and towards the ventricular center they are almost horizontal. They are not perfectly horizontal because the outer edges have a divergent tendency. We say that the lamellae are oriented relatively radially towards the surface of the heart. The inter-lamellar anastomoses play the role of the cohesion threads from the structure of a shutter. These threads are arranged in three columns: the central (near the middle of the lamella), the interior and the exterior column- a position similar to that of the cohesion threads from the structure of a shutter (Figs. 7.22, 7.23, 7.24, 7.25, 7.26, 7.28, 7.29 and 7.30). Similarly to the functional principle of the shutter, the central column ensures, first of all, co-axiality, while the inner and outer columns modify the inclination angle of the lamellae. This is possible due to the progressive excitation of the myocardium starting from the apex toward the basis and from the interior towards the exterior. During the systole the lamellae become horizontal, thus explaining the thickening of the wall in systole. The horizontalization of the lamellae concentrates myocyte forces, offering the maximum of expulsion power. During the diastole the lamellae become slanted, explaining the thinning of the ventricular wall. This type of behaviour of the lamellar structure should not be understood as an isolated behaviour, but, on the contrary, as integrated in the generality of a coherent parietal contraction, which is multisectorial and multiaxial! The lamellae have a luminal region, a central and a superficial one (towards the epicardium). The fibers and muscular bundles from the luminal region, forced by the blood flow to re-orientate during ontogeny, do not keep the lamellar arrangement, they are cut off from the lamellae and arranged in the direction of the blood flow. These fibers still keep the anastomoses with the fibers and the bundles from the neighbouring lamellae. They change into trabeculations oriented in the direction of the flow forces. In the classic description they correspond to the deep myocardial layer. The trabeculations contain bundles from more neighbouring lamellae (Fig. 7.34). The fibers and the bundles from the superficial region, under the influence of intrapericardial pressure (in phylogeny and ontogeny) gradually lose the lamellar arrangement, preserving however the anastomoses with the neighbouring fibers and bundles as well as with the original lamellae. These fibers will make up the superficial layer. Their orientation (as well as the orientation of the other layers) is mostly the result of the folding and reorganization of the heart tube (Figs. 7.35 and 7.36).
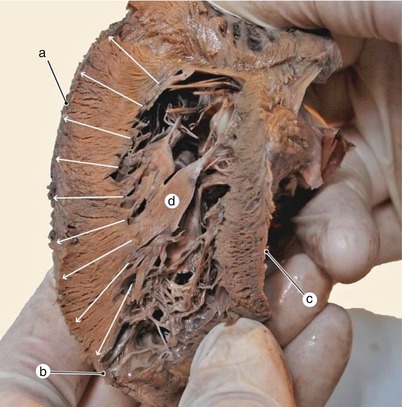
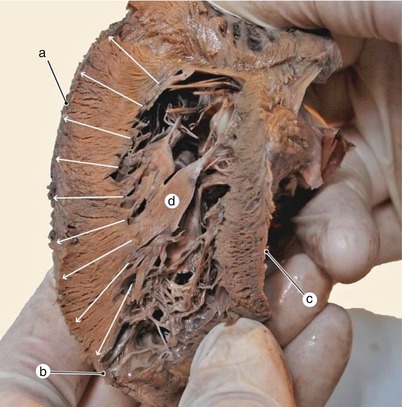
Fig. 7.22
Paramedian sagittal section through the left ventricle. The arrows show radial arrangement of the lamellae in the ventricular wall structure. The subepi-subendocardic superposition from the apex allows ventricular wall extension. a pulmonary side of the left ventricle, b epi-endocardic superposition at the ventricular apex, c diaphragmatic side of the left ventricle, d posterior papillary group
The fibers from the central region of the ventricular wall make up characteristic lamellae, anastomosed by the three types of interlamellar columns. They form the middle layer in the classic description. There is no uniformity in the size and orientation of these lamellae. Clearly, the lamellae do not reach (and there is no need to do so) the subendocardium or subepicardium. Their arrangement is not perfectly regulated and uniform in the whole ventricular wall, but they certainly exist. The columns are not perfectly sketched, rigorous, yet the interlamellar anastomoses between the envisaged areas certainly exist and the described structures perform their function, in all probability, according to the metaphor and the principle of the shutter. Thus structured, the myocardium really forms three layers, without a strict delimitation between them, the orientation of the fibers progressively changing, from the superficial towards the deep layer. Due to the progressive change in the direction of the fibers, the wall of the heart is compact and resistant. There are areas, however, near the apex of the heart and in the vicinity of interventricular grooves, where this superposition of differently oriented layers is not perfect. Thus the danger of the decompaction of myocardial structure appears (Fig. 7.37), leading to the superposition of the epicardium and the endocardium. The possibility of decompaction is raised in dilated hearts with probably an altered extracellular matrix. In conclusion, the structure of the ventricular myocardium is simultaneously lamellar and stratified. The structural unities (fibers, bundles, lamellae) are branched and anastomosed, which creates structural interdependency and functional unity. There are certainly three layers: the superficial layer with predominantly slanted fibers towards the long axis. The middle layer has predominantly circular fibers, perpendicular on the long axis. The deep, trabecular layer has a reversed obliqueness towards the superficial layer. The transitions between the three layers are progressive. There are no cleavage planes or strict delimitations at the borders between layers.
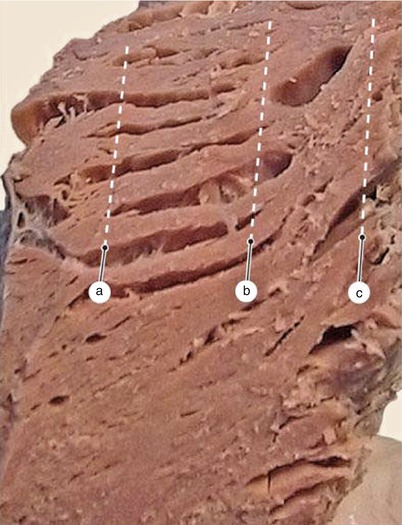
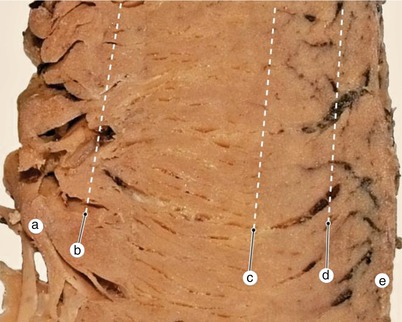
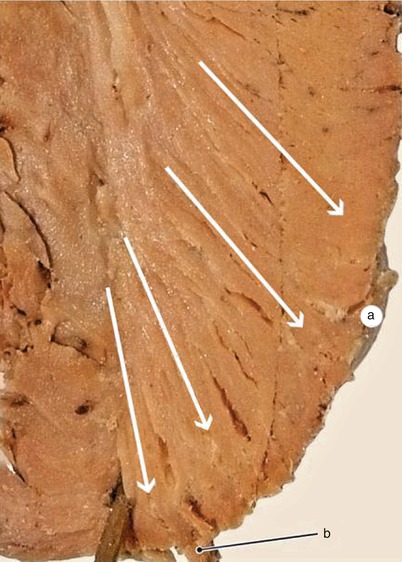
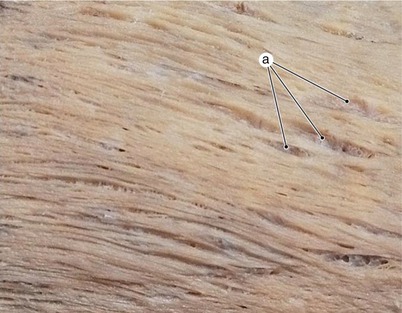
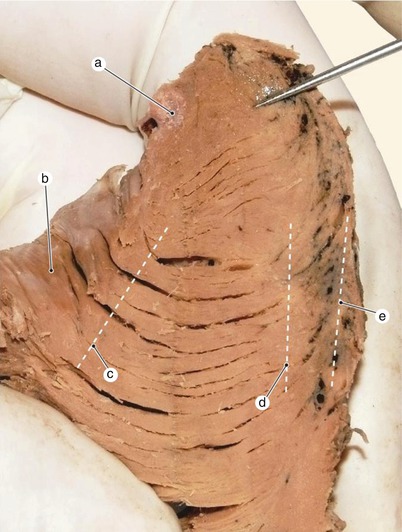
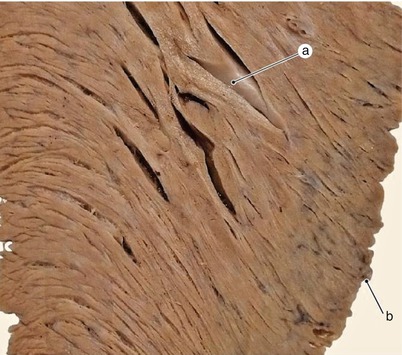
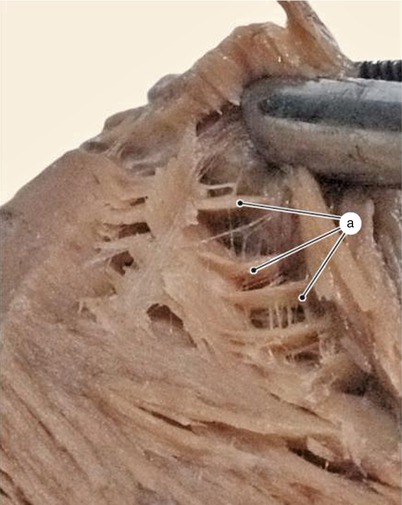
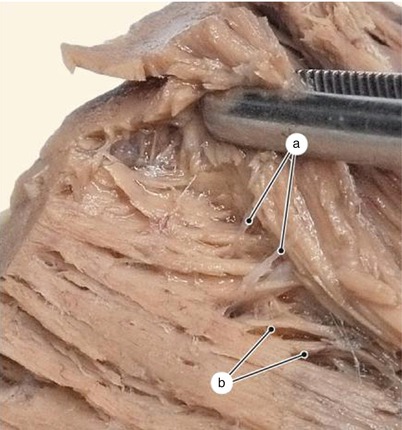
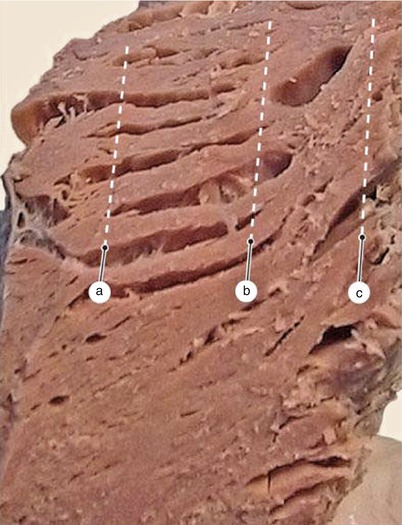
Fig. 7.23
Inter-lamellar muscular anastomoses are arranged like cohesion lines from the structure of a horizontal blind system. Between lamellae are three cohesion areas arranged from top to bottom in the depth of the ventricular wall. a internal cohesion area, b central cohesion area, c external cohesion area
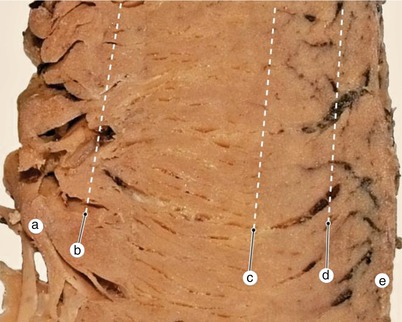
Fig. 7.24
Arrangement of the most important sights of inter-lamellar cohesion in the middle third of the left ventricular wall, on the pulmonary face. a trabeculae with origin in different lamellae, b internal cohesion area, c central cohesion area, d external cohesion area, e diaphragmatic face of the left ventricle
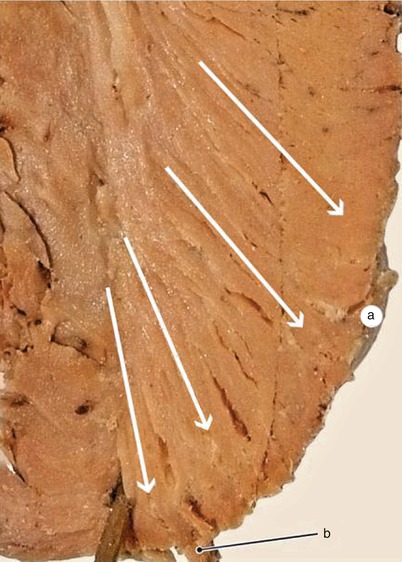
Fig. 7.25
Sagittal section through the wall of the left ventricle, on the pulmonary side. Approaching the apex the lamellae orientation (arrows) in the ventricular wall becomes oblique, until in the apex area the lamellae are almost vertical. a diaphragmatic side of the left ventricle, b apex
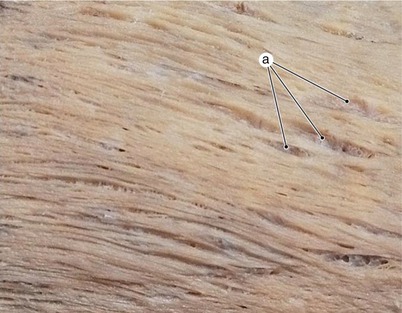
Fig. 7.26
Tangential section in the wall of the left ventricle on the diaphragmatic surface. The lamellar structure of the wall and extra-cellular matrix are obvious. a extra-cellular matrix
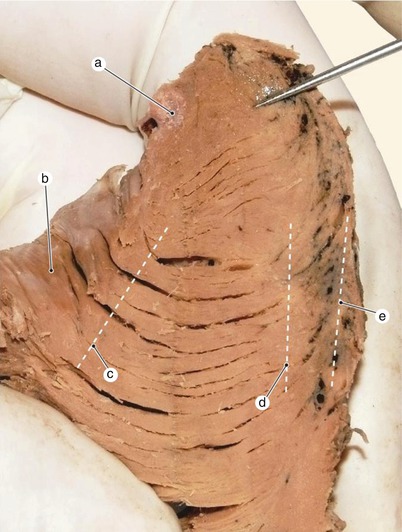
Fig. 7.27
Sagittal section in the wall of the left ventricle, on the diaphragmatic surface, near the ventricular base. The top of the needle is placed above a lamellar system of the ventricular base, that assures the semi-sphincter behavior of the left ventricular base. a mitral fila coronaria, b aortic vestibule, c internal cohesion area
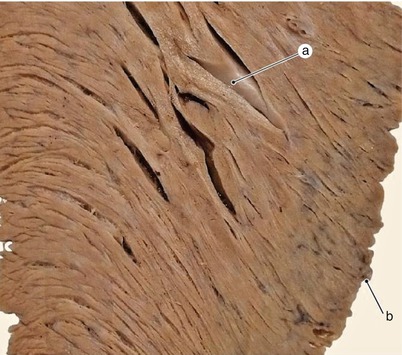
Fig. 7.28
Sagittal section in the wall of the left ventricle the diaphragmatic surface in the inferior third. The lamellar structure is evident. The muscular trabeculae have origin in the nearby lamellae. a trabecula with origin in different lamellae, b diaphragmatic side
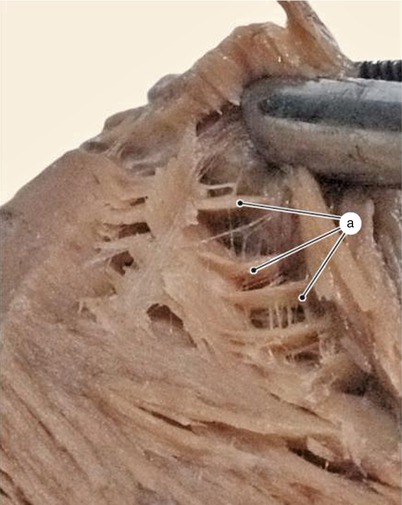
Fig. 7.29
Besides the most important areas of cohesionm, the lamellae are interconnected on their entire length through small inter-lamellar fascicles. a uniting inter-lamellar fascicles and extra-cellular matrix
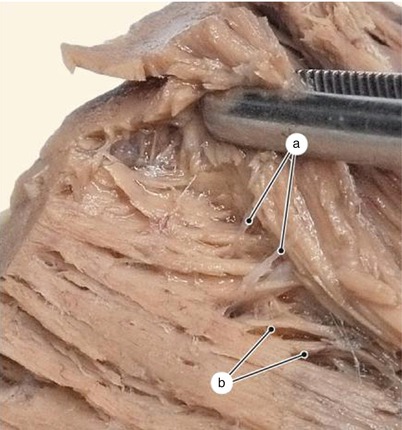
Fig. 7.30
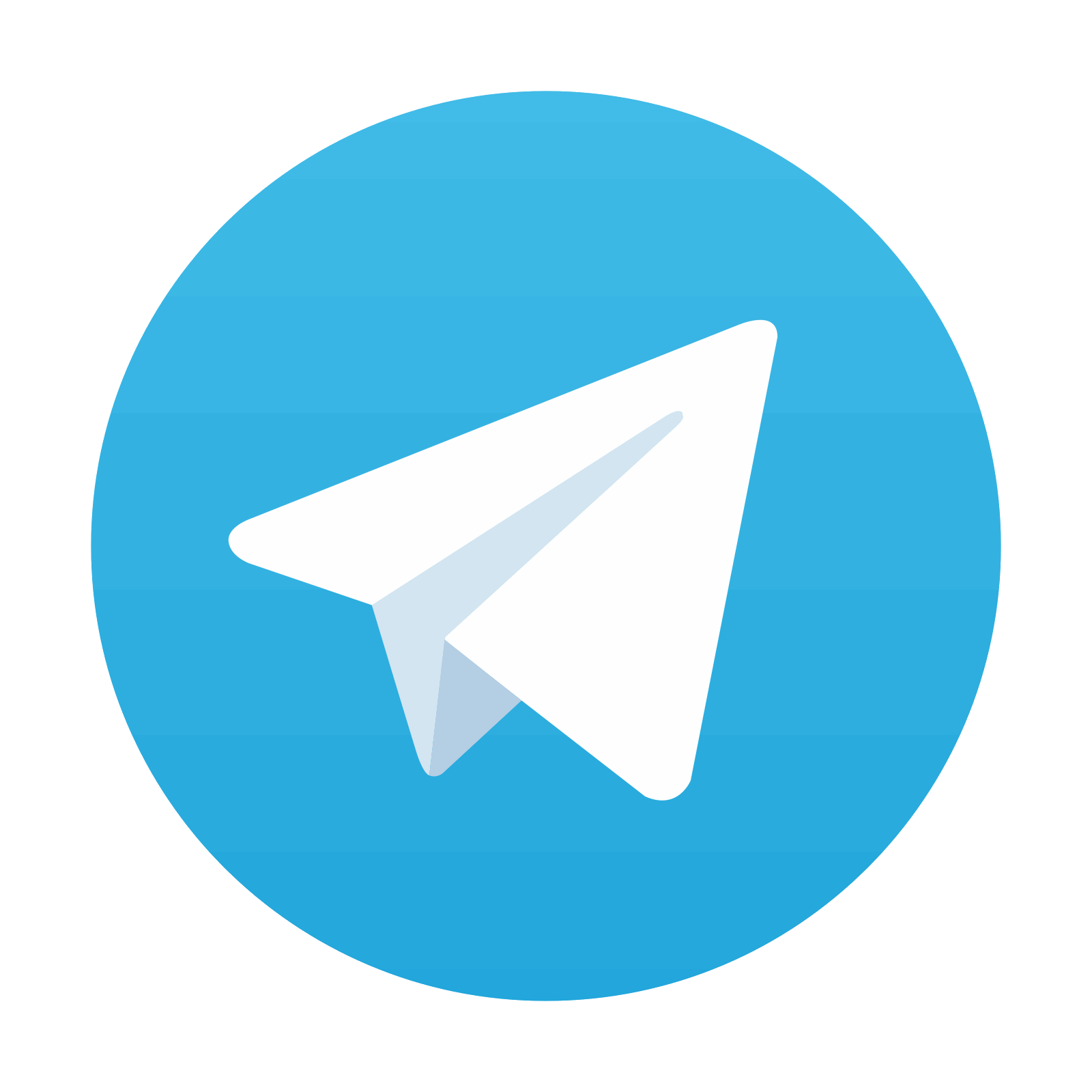
Connecting inter-lamellar fascicles. a extra-cellular matrix, b uniting inter-lamellar fascicles
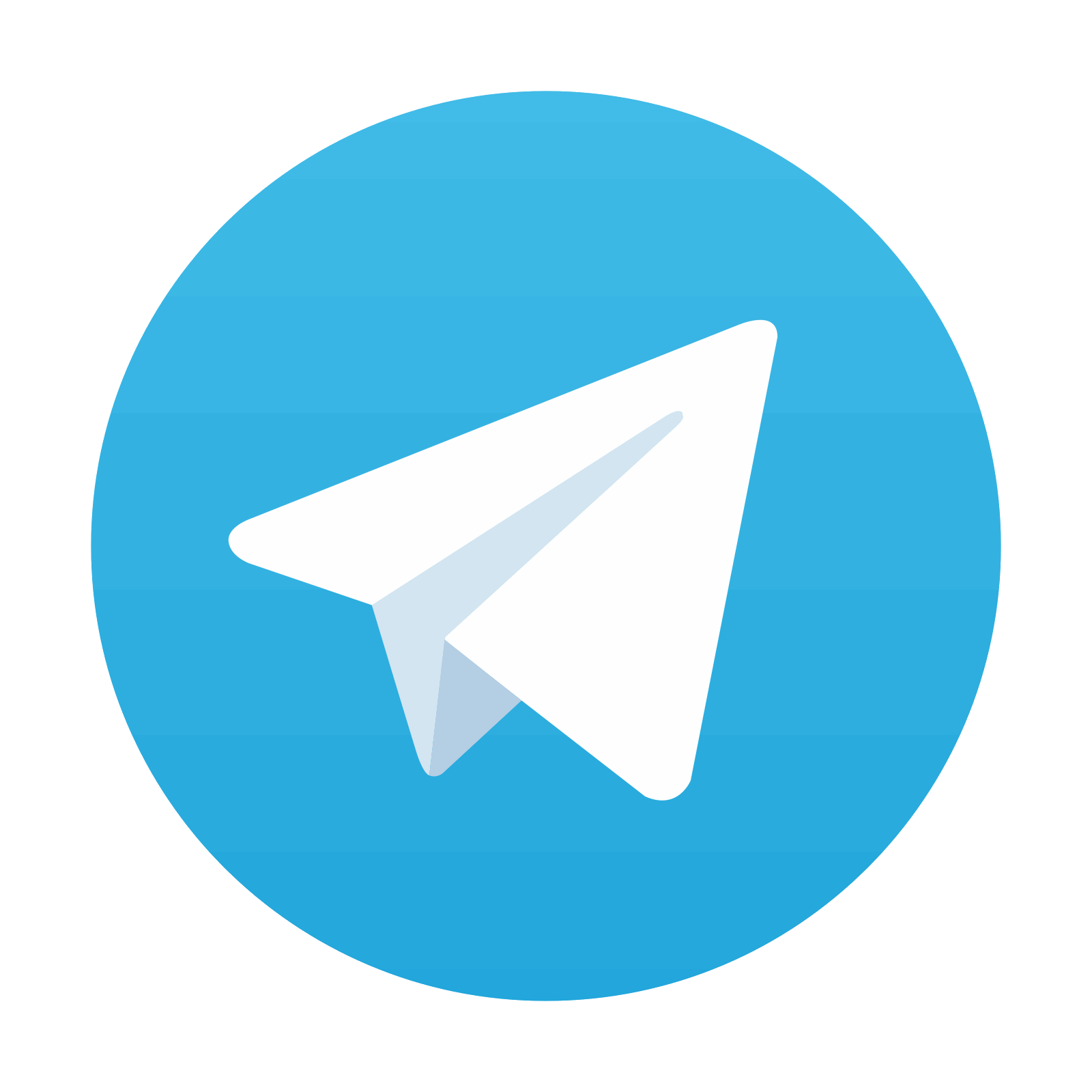
Stay updated, free articles. Join our Telegram channel

Full access? Get Clinical Tree
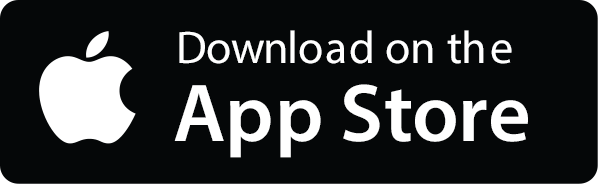
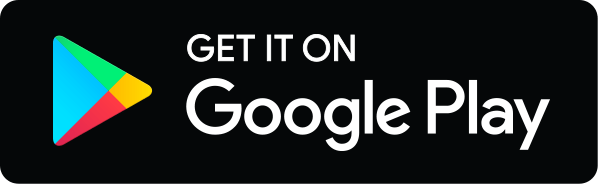
