Fig. 6.1
CDC and flow methods for cross-matching and determination of circulating HLA alloantibodies Abbreviations: CDC Complement Dependent Cytotoxicity, AHG Anti-human Globulin (Reused with permission from Tinckam and Chandraker [29])
More recent techniques using flow cytometry or enzyme-linked immunosorbent assay (ELISA) [30] are much more sensitive for the detection of antibodies and generally provide more reproducible results. The Luminex® test allows for simultaneous detection of multiple antibodies, as up to 100 color-coded, antigen-coated microspheres can be detected in a single well [32]. The FlowPRA® test is a flow cytometry based technique which consists of a pool of microparticle beads coated with full HLA Class I or Class II phenotype derived from purified HLA-bearing cell lines [33]. The percentage of PRAs can be determined by calculating the percentage of beads that react positively with patient sera. Due to variability in results between techniques, many laboratories will utilize multiple confirmatory tests.
While these assays are useful screening tools for determination of PRA, further tests are required to determine specificity and quantification of alloantibodies. The importance of the strength of circulating antibody is increasingly recognized as an important factor determining the risk of a cytotoxic response. For determination of multiple antibody specificities and quantification of antibodies, single-antigen bead (SAB) assays are now commonly deployed [34]. These assays contain beads individually coated with a specific purified recombinant HLA molecule that identifies antibody specificity.
The ability to detect and quantify the strength of specific antibodies has allowed estimation of which recipient circulating antibodies are present at a strength which may prove to be cytotoxic for a potential donor organ. Laboratories will typically perform validation studies to determine the relationship between antibody levels determined by single antigen beads and flow crossmatch. For this purpose, the strength of antibody binding on single antigen beads as represented by Mean Fluorescence Intensity (MFI) is compared to the degree of the flow crossmatch reported as median channel shift (MCS). However, it is important to note that MFI is a measure of antibody-antigen binding or HLA molecule bead saturation rather than a direct measure of antibody titer.
MFI is also affected by several technical and biologic factors. Antigen density on beads may vary depending on type of HLA molecule, between different assay manufacturers, assay batches, and antigen density on beads may not reflect the natural expression of HLA molecules on cells in vivo. Antibodies against public HLA epitopes (antigenic determinants produced by more than one gene) may be under-estimated and appear as a weak antibody due to a dilutional effect resulting from a single antibody being distributed across many beads. The presence of endogenous C1q inhibitors can also mask detection of HLA antibodies. This is known as the “prozone effect”. These C1q inhibitors may be diluted out or eliminated by heat inactivation or denaturing with dithiothreitol (DTT) or ethylenediaminetetraacetic acid (EDTA) [35–37]. The process of HLA molecule purification and coating on to the bead surface may also alter the natural conformation of antigens leading to exposure or loss of antigenic epitopes. Therefore, HLA antibodies may remain undetected if they are unable to bind to the distorted HLA molecule. Conversely, clinically irrelevant antibodies may be detected that bind to the denatured but not intact antigen. In one study, a fifth of patients awaiting a heart transplant had at least one antibody to cryptic epitopes that led to a false increase in calculated PRA (cPRA) by 5% [38]. Importantly, the technique allows detection of low level antibodies which may trigger a strong amnestic immune response upon re-exposure to the antigen.
Virtual Crossmatch
In the past, sensitized patients needed a prospective cross-match to ensure allograft compatibility before transplantation. A prospective crossmatch identifies donor hearts which may be at risk of exposure to circulating cytotoxic antibodies. This method however can be logistically challenging as recipient blood has to be available close the donor in order for the test to be performed in a timely manner. The test also requires local expertise and samples also need to be kept updated as clinical conditions change for the recipient who may be challenged with further potentially sensitizing events such as blood transfusions or ventricular assist device placement while awaiting transplantation. Recipient blood from sensitized patients has to be sent out to several locations where donors could potentially become available. The need for a prospective cross-match inherently limits the geographical area from which sensitized patients may qualify for organ donors and therefore substantially increases the waiting time to transplant.
The ability to perform high resolution antibody screening with solid phase assays has fortunately simplified appropriate donor selection by the use of a process termed “the virtual crossmatch.” In a virtual crossmatch, the recipient antibody profile is determined at the time of listing and potentially cytotoxic antibodies to HLA antigens identified. Cytotoxicity is presumed from the strength of the antibody following correlation studies with flow cytometry cross match as described above [39]. The corresponding antigens are then documented as unacceptable on the transplant list. The principle advantage of this strategy is that it obviates the need to send out recipient blood and therefore substantially increases the geographical region from which a donor may be accepted. This approach has been shown to substantially decrease the waiting time to transplant [40]. The virtual crossmatch also helps identify patients who are potentially at elevated risk of rejection in whom immunosuppression may need to be augmented after transplantation. The use of the virtual crossmatch has been validated in several studies. In pediatric heart transplant recipients the use of the virtual crossmatch was associated with a significantly decreased waiting time to transplant and improved survival compared to patients listed with a prospective crossmatch [41]. In another study the accuracy of virtual crossmatch was compared to prospective AHG-CDC crossmatch [42]. Based on analysis of 257 T-cell AHG-CDC crossmatch tests, the positive predictive value of virtual crossmatch (the likelihood of an incompatible virtual crossmatch resulting in an incompatible T-cell CDC-AHG crossmatch) was 79%, and the negative predictive value of virtual crossmatch (the likelihood of a compatible virtual crossmatch resulting in a compatible T-cell CDC-AHG crossmatch) was 92%. When used prospectively in a cohort of 28 sensitized patients awaiting heart transplantation, 14 received allografts based on a compatible virtual crossmatch alone from donors in geographically distant locations. Compared with the other 14 sensitized patients who underwent transplant after a compatible prospective serologic crossmatch, the rejection rates and survival were similar. About 65% of heart transplant centers now utilize the virtual crossmatch [43].
It is however unlikely that all classes and types of HLA antibodies have an equal impact on their ability to mount allograft rejection. The role of HLA-C and HLA-DP mismatches, for example, in allograft survival and their consideration in virtual crossmatch is still undetermined.
In contrast with CDC assays, conventional solid-phase assays do not discriminate between complement-fixing and non–complement-fixing antibodies. Avoidance of non-complement fixing and therefore non-cytotoxic antibodies in the virtual crossmatch may therefore unnecessarily restrict the donor pool. A novel C1q assay developed to detect the sub-set of immunoglobulin G (IgG) antibodies capable of fixing complement may allow further expansion of the donor pool by allowing exclusion of only complement fixing antibodies in the virtual crossmatch [44]. In this study the identification of complement fixing antibody in a standard virtual crossmatch correlated with a higher incidence of AMR compared to a virtual crossmatch with no complement fixing antibodies. Another important observation is that the ability of antibody to fix complement is independent of the strength of antibody and C1q fixation is independent of MFI values [45]. The C1q assay appears to be much more sensitive than the standard CDC at detecting complement fixing antibodies.
Given the variety of testing now available to evaluate the sensitized heart transplant candidate, an algorithm for assignment of unacceptable antigens for the virtual crossmatch has been used to allow transplantation of highly sensitized patients across the DSA barrier with survival rates comparable to DSA negative heart transplant recipients [46]. In this protocol, 4 antibody detection methods were used to prioritize unacceptable antigens: Luminex single antigen (LSA), LSA with 1:8 dilution, C1q LSA and CDC panel.
Non-HLA Antibodies
Antibody responses to non-human leukocyte antigens (HLA) have been reported in solid organ transplantation and may occur as alloantibodies or autoantibodies. In thoracic organ transplantation, several have been implicated in acute and chronic allograft rejection. The antibodies identified include those against major histocompatibility class I chain-related gene A (MICA) [47, 48], angiotensin II type 1 receptor (ATIR) [49], endothelin-1 type A receptor (ETAR) [50], endothelial cell antigens [51], vimentin [52], K-alpha-1-Tubulin (KA1T) [53], and collagen-V [54] and other non-HLA IgM antibodies. In cardiac transplantation, anti-MICA and anti-endothelial antibodies have been associated with increased antibody mediated rejection [55] and development of cardiac allograft vasculopathy [47]. AT1R and ETAR have been implicated with development of ACR, AMR and early onset microvasculopathy [50].
The detection of these antibodies in the setting of acute and chronic rejection however at this time remains for investigational use and none of the assays used to detect these antibodies have been routinely used for clinical evaluation. The clinical significance and the role of these antibodies in mediating thoracic allograft injury currently remains chiefly undetermined.
Calculated PRA (cPRA)
Not all sensitized patients require treatment to decrease antibody burden. The need for therapy is dictated by the calculated PRA (cPRA). The cPRA value represents the percentage of donor hearts in a given population to which a heart transplant candidate will have cytotoxic anti-HLA antibodies.
The cPRA is determined based on the antibody strength threshold (MFI) for cytotoxicity determined by the individual center’s laboratory. For example, if the laboratory determines that antibodies with MFI >5000 correlate with cytotoxicity, only those corresponding antigens will be entered into the calculator available online at the Organ Procurement and Transplantation Network website from the US Department of Health and Human Services. The current cPRA data is based upon HLA frequencies derived from the HLA phenotypes of deceased kidney donors recovered over a 2 year period in 2003–04. Ethnic frequencies were derived from data in 2006–07.
As the cPRA is an estimation of the proportion of the donors not suitable for the sensitized patients, generally patients with a cPRA >50% may be considered for desensitization therapies as less than half the donor pool would be suitable for transplantation without treatment. The aim of desensitization therapy is to reduce antibody burden to facilitate a reduction in the cPRA and increase the chances of obtaining a suitable organ.
Therapeutic Options for the Sensitized Patient
The humoral response involves B cells, plasma cells, antibodies and complement. All of these have been commonly targeted as therapeutic options for desensitization (Table 6.1). Strategies for desensitization continue to evolve but published clinical data remain sparse and protocols in heart transplantation have been adapted from experience in renal transplantation. The general approach is to use multiple complementary therapies which are aimed at removing or neutralizing alloantibodies and suppress further production.
Table 6.1
Strategies to prevent antibody-mediated rejection
Approaches | Therapies |
---|---|
Antibody removal | Therapeutic plasma exchange, immunoadsorption |
To alter antibody production | Rituximab, ATG, Bortezomib |
B-cell modulation | |
Plasma cell depletion | |
Immunomodulation (Ab inactivation) | IVIG |
Suppression of the T-cell response | Steroids, ATG, MMF, CNI, PSI |
Complement blockade | Eculizumab |
Plasmapheresis and Immunoadsorption
Plasmapheresis allows physical removal of circulating antibodies. In one study, sensitized heart transplant candidates treated with pre-operative plasmapheresis and intravenous immunoglobulin (IVIg) had similar rates of rejection and allograft survival compared to non-sensitized controls [56]. Antibody rebound due to rapid diffusion from the extravascular space and reflex stimulation of plasma cells leading to increased production can occur, and multiple treatments are usually needed to achieve low circulating antibody levels. Large bore intravenous access is required and filtration of clotting factors with plasmapheresis may require replacement with fresh frozen plasma instead of albumin to correct coagulopathy. Immunoadsorption allows targeted removal of allo-antiobodies and may be more effective than plasmapheresis [57].
Intravenous Immune Globulin (IVIg)
Intravenous immunoglobulin (IVIg) is a product of pooled IgG antibodies (immunoglobulin) extracted from the plasma of up to 100,000 blood donors. Originally developed for the treatment of primary immunodeficiency disorders, the product was found to have significant immunomodulatory effects. Its use was therefore expanded to treat autoimmune and inflammatory diseases and subsequently organ transplantation. IVIg has multiple immune effects including Fc receptor blockade, inhibition of complement deposition, enhancement of regulatory T cells, inhibition or neutralization of cytokines and B cell growth factors, accelerated clearance of autoantibodies, modulation of adhesion molecules and cell receptors, cross-linking B cell receptor and FcγRIIB, which reduce APC activity and induce B cell apoptosis and activation of regulatory macrophages [58, 59] (Fig. 6.2). A randomized placebo-controlled trial in sensitized patients awaiting renal transplantation revealed efficacy of high dose IVIg in reducing PRA leading to improved transplant rates but had no effect on rejection or graft loss [60]. In a study of sensitized LVAD recipients awaiting heart transplant [61], patients received monthly courses of either IVIg or plasmapheresis, in conjunction with cyclophosphamide. Prolongation in transplant waiting time was related to the presence of Class I antibodies. Infusion of IVIg (2 g/kg) caused a mean reduction of 33% in anti-HLA class I alloreactivity within 1 week. Waiting time to transplantation was significantly reduced by IVIg therapy and subsequently matched nonsensitized patients. Although plasmapheresis caused a similar reduction in antibodies, this effect was achieved after longer treatment. Plasmapheresis was associated with an unacceptably high frequency of infectious complications. In this study IVIg appeared to be more effective than plasmapheresis in reducing PRA with a superior safety profile.


Fig. 6.2
Mechanism of Intravenous Immunoglobulin (IVIg) activity (Reused with permission from Schwab and Nimmerjahn [59])
Cyclophosphamide
Cyclophosphamide has been used to prevent B cell rebound. In one study [62], intravenous cyclophosphamide pulse therapy in conjunction with IVIg reduced waiting time and mortality in sensitized patients to levels in non-sensitized patients. After transplant, cyclophosphamide prevented induction of IgG anti-HLA class II, prolonged the rejection-free interval, and reduced cumulative rejections to levels in non-sensitized patients. The risk of rejection was 3.7-fold higher in patients treated with mycophenolate mofetil than patients treated with cyclophosphamide. There were no differences in infectious or other significant complications. However, concerns for direct cardiac toxicity and other adverse effects have limited widespread use.
Rituximab
Rituximab is a chimeric monoclonal antibody against CD20 expressed on pre-B and mature B lymphocytes and was developed for the treatment of lymphoma. CD20 has an important role in B cell maturation, regulating the early stages of cell cycle initiation and differentiation. Rituximab causes B cell depletion by complement dependent antibody dependent cytotoxicity and apoptosis. In sensitized patients awaiting renal transplantation, rituximab in conjunction with IVIg has been shown to significantly reduce PRA, shorten time to transplant and provide excellent 12 month graft and patient survival [63]. In heart transplantation [64], 21 patients treated with a combination of plasmapheresis, IVIg and rituximab had a mean reduction of PRA from 70.5 to 30.2%, resulting in a negative prospective donor specific crossmatch and successful heart transplantation. Compared with the control group (PRA <10%), the treated sensitized group had similar 5-year survival and freedom from cardiac allograft vasculopathy. In a prospective study, 14 sensitized pediatric patients awaiting heart transplant underwent desensitization with high dose IVIg and rituximab [65]. Eight patients had a significant decrease in cPRA, although six required multiple doses for a response. The total number of unacceptable antigens decreased for all eight responders leading to a median increase in the percentage of potential donors in the overall population from 10% pre-treatment to 85% post-treatment.
Bortezomib
Although plasmapheresis, IVIg and rituximab variably reduce antibody burden, these modalities have no suppressive effect on the cell responsible for antibody production, the mature plasma cell. Bortezomib is a selective 26S proteosome inhibitor used in the treatment of multiple myeloma, a plasma cell neoplasm. In vitro, bortezomib demonstrated plasma cell apoptosis and blocked anti-HLA antibody production [66]. In contrast, IVIg, rituximab and anti-thymocyte globulin had no effect on suppressing antibody production by plasma cells. In one study, 34 highly sensitized patients awaiting living-related donor renal transplant underwent desensitization with a combination of plasmapheresis, bortezomib, rabbit-ATG, mycophenolate mofetil (MMF) and IVIg [67]. In total 29/34 patients responded within 1 month with a significant reduction in AHG-CDC and flow cytometry crossmatches. Side effects were noted in 38% of patients and were manageable. Two patients lost a graft at 1 year and acute rejections were noted in a quarter of the patients, which responded to steroids and rATG.
In heart transplantation, bortezomib in conjunction with plasmapheresis was studied in seven sensitized patients awaiting heart transplantation, who despite prior treatment with rituximab, IVIG and/or plasmapheresis continued to have high antibody levels and cPRA [68]. In this study, bortezomib appeared to be generally well tolerated, with treatable infection being the most common adverse effect. The protocol was effective at significantly reducing antibody concentrations. Mean cPRA was reduced from 62 to 35% following bortezomib with plasmapheresis (p = 0.02). 6/7 patients demonstrated significant decline in antibody levels. One patient remained refractory to desensitization therapy. Four patients successfully underwent cardiac transplantation without evidence of rejection or graft dysfunction. One patient developed early post-transplant graft dysfunction and died at 1 month from sepsis. All but one patient were listed Status 1A at the time of desensitization. In a subsequent follow-up of 22 patients [69], plasmapheresis and bortezomib was confirmed to be effective at reducing HLA antibody burden in a majority of sensitized patients, including patients with high levels of antibodies as determined by 1:8 dilution LSA and C1q assay. Patients who received prior desensitization therapies with plasmapheresis, IVIg and rituximab appeared to have a greater response suggesting that combination therapies appear to be more effective. The majority of patients were able to undergo transplant with excellent 1 year survival and low treated rejection rates.
The primary route of synthesis of HLA class I molecules is dependent on peptide generation by the proteasome, whereas that of class II is not. Therefore, patients with Class I antibodies appear to have a greater response to desensitization compared to patients with Class II antibodies [70].
The combination of plasmapheresis with bortezomib may be more effective as removal of circulating antibody by plasmapheresis results in increased metabolic demands on B-cells, memory B-cells and plasma cells to produce more antibody. This metabolic stress enhances the sensitivity of plasma cells to proteasome inhibition. Plasmapheresis during bortezomib therapy also provides the additional benefit of removing pre-existing circulating antibody. As this combination of treatment may be provided over a period as short as 2 weeks, it may be particularly useful for patients listed Status 1A for heart transplant.
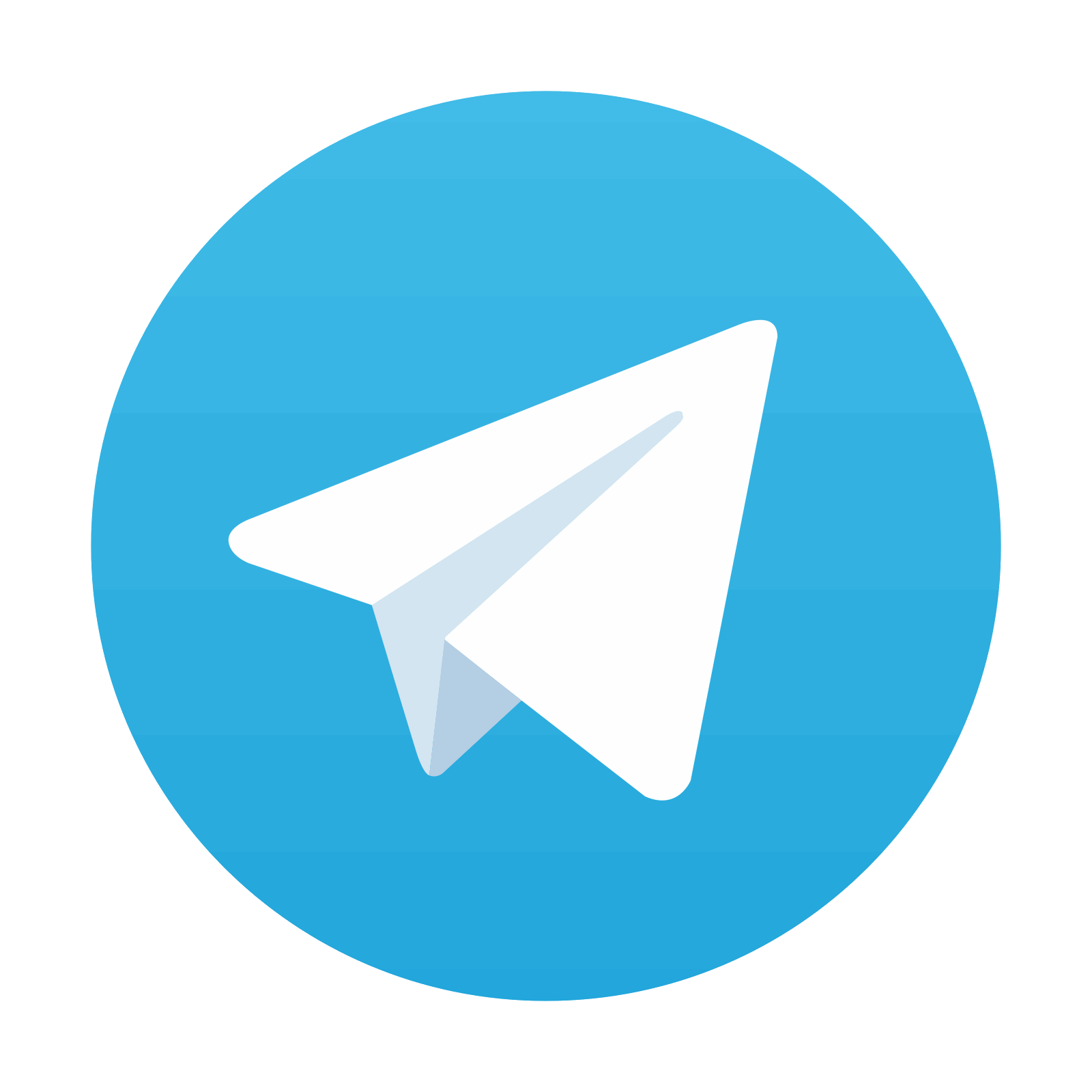
Stay updated, free articles. Join our Telegram channel

Full access? Get Clinical Tree
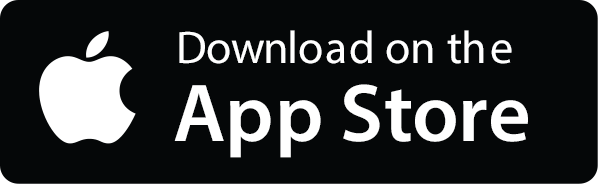
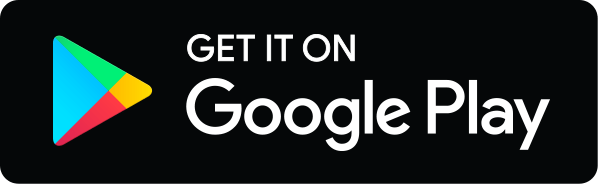