Historical Review
It is often difficult to ascertain where concepts begin. It is not obvious who first used the term rotator cuff or musculotendinous cuff. Credit for first describing ruptures of this structure is often given to J.G. Smith, who described the occurrence of tendon ruptures after shoulder injury in the London Medical Gazette in 1834 . In 1924, Meyer published his attrition theory of cuff rupture. In his 1934 classic monograph, Codman summarized his 25 years of observations on the musculotendinous cuff and its components, and discussed rupture of the supraspinatus tendon. Beginning 10 years after the publication of Codman’s book and for the next 20 years, McLaughlin wrote on the etiology of cuff tears and their management. Arthrography, with air used as the contrast medium, was first carried out by Oberholtzer in 1933. Lindblom and Palmer used radiopaque contrast and described partial-thickness, full-thickness, and massive tears of the cuff.
Codman recommended early operative repair for complete cuff tear and carried out what may have been the first cuff repair in 1909. Current views of cuff tear pathogenesis, diagnosis, and treatment are quite similar to those that he proposed. Codman and numerous well-known surgeons, including Armstrong, Hammond, McLaughlin, Moseley, Smith-Petersen et al., and Watson-Jones suggested that subacromial abrasion may be an element in rotator cuff disease. Some of these surgeons proposed complete acromionectomy for relief of these symptoms, whereas others advocated lateral acromionectomy.
The term impingement syndrome was popularized by Charles Neer in 1972. Neer emphasized that the supraspinatus insertion to the greater tuberosity and the bicipital groove lie anterior to the coracoacromial arch with the shoulder in the neutral position, and also noted that with forward flexion of the shoulder, these structures must pass beneath the arch, thereby providing the opportunity for abrasion. He suggested a continuum from chronic bursitis and partial tears to complete tears of the supraspinatus tendon that can extend to involve rupture of other parts of the cuff. He pointed out that physical examination and plain radiographic findings were not reliable in differentiating chronic bursitis and partial tears from complete tears. Importantly, he emphasized that patients with partial tears seemed more prone to increased shoulder stiffness and that surgery in this situation was inadvisable until the stiffness had resolved.
In 1972, Neer described the indications for acromioplasty as complete tears of the supraspinatus or long-term disability from chronic bursitis and partial tears of the supraspinatus tendon. He described operating on only 50 shoulders over a 5-year period, demonstrating his great discretion regarding the removal of “impinging” bone. If surgery was performed, Neer pointed out the importance of preserving a secure acromial origin of the deltoid, performing a smooth resection of the undersurface of the anteroinferior acromion, carefully inspecting for other sources of abrasion (e.g., the undersurface of the acromioclavicular joint), and ensuring careful postoperative rehabilitation. He also reported that patients with an irreparable rotator cuff tear could be made more comfortable and could gain surprising function after acromioplasty, as long as the deltoid origin was preserved.
He described the lateral acromion as “an innocent part,” and recommended removing only the anterior edge. But what if all of the acromion is innocent? In his commentary on Neer’s article, Zarins stated:
Neer believed that impi n gement causes rotator cuff tears. This hypothesis does not appear to have withstood the test of time. It is more likely that rotator cuff dysfunction results in upward displacement of the humeral head and causes impingement of the humeral head against the acromion with shoulder use rather than the reverse.
Over time, strict adherence to Neer’s original indications for acromioplasty has relaxed. With the advent of arthroscopic acromioplasty initially popularized by Ellman, the frequency with which this procedure was performed had increased dramatically, and became one of the most common of all orthopedic procedures to treat “impingement syndrome.” Additional approaches to subacromial abrasion had been proposed, including section of the coracoacromial ligament, resection arthroplasty of the acromioclavicular joint, extensive acromionectomy, and combined procedures such as acromioplasty, incision of the coracoacromial ligament, acromioclavicular resection arthroplasty, and excision of the intra-articular portion of the biceps tendon with tenodesis of the distal portion to the bicipital groove.
Subsequently, a vast body of evidence over the next couple of decades did not support extrinsic compression of the rotator cuff as the cause for cuff pathology but rather age-related intrinsic degeneration of the tendon. Numerous studies on the outcomes of isolated acromioplasty and rotator cuff repair with and without acromioplasty have demonstrated no difference in outcomes. With the most recent evidence demonstrating its limited role, the enthusiasm for and prevalence of acromioplasty for impingement syndrome has since diminished.
Recently, Papnikodolakis et al. summarized:
The concept of impingement syndrome was originally introduced to cover the full range of rotator cuff disorders, as it was recognized that rotator cuff tendinosis, partial tears, and complete tears could not be reliably differentiated by clinical signs alone. The current availability of sonography, magnetic resonance imaging (MRI), and arthroscopy now enable these conditions to be accurately differentiated. Nonoperative and operative treatments are currently being used for the different rotator cuff abnormalities. Future clinical investigations can now focus on the indications for and the outcome of treatments for the specific rotator cuff diagnoses. It may be time to replace the nonspecific diagnosis of so-called impingement syndrome by using modern methods to differentiate tendinosis, partial tears, and complete tears of the rotator cuff.
Relevant Shoulder Anatomy
Deltoid Muscle
The deltoid arises from the lateral half of the clavicle, the acromion, and posteriorly from the scapular spine. The deltoid has an important and constant tendon of origin separating its anterior and lateral thirds. This tendon attaches to the anterolateral corner of the acromion, in which location it provides the key to the anterior deltoid-splitting approach to the cuff ( Fig. 14-1 ).

Open rotator cuff surgery can usually be accomplished with this deltoid split approach through cosmetically acceptable incisions in the lines of the skin without taking down the deltoid origin. By splitting the deltoid down the center of the tendon, the surgeon can be assured of having strong tendinous handles on the muscle to use in closing the deltoid. Although it is often said that the location of the axillary nerve is on average 5 cm distal to the acromion, its anterior branches turn upward, so it is desirable to limit the inferior extent of the deltoid split to minimize the risk of injury to the axillary nerve branches.
Rotator Cuff
The rotator cuff is a complex of four muscles that arise from the scapula and whose tendons blend in with the subjacent capsule as they attach to the tuberosities of the humerus. The subscapularis arises from the anterior aspect of the scapula and attaches over much of the lesser tuberosity. It is innervated by the upper and lower subscapular nerves. The supraspinatus muscle arises from the fossa that lies superior to the scapular spine on the posterior portion of the scapula, passes beneath the acromion and the acromioclavicular joint, and attaches to the superior aspect of the greater tuberosity. The suprascapular nerve innervates the supraspinatus after the nerve passes through the suprascapular notch. The infraspinatus muscle arises from the fossa below the scapular spine on the posterior portion of the scapula and attaches to the posterolateral aspect of the greater tuberosity. The suprascapular nerve innervates the infraspinatus after the nerve passes through the spinoglenoid notch. The suprascapular nerve is at risk of compression from paraglenoid cysts at both the suprascapular and spinoglenoid notches. The teres minor arises from the lower lateral aspect of the scapula and attaches to the lower portion of the greater tuberosity. It is innervated by a branch of the axillary nerve. The tendons of the rotator cuff blend together to form a continuous cuff around the humeral head.
Histologic studies by Clark and Harryman described the rotator cuff tendons as having five distinct layers ( Fig. 14-2 ). The most superficial layer is the coracohumeral ligament fibers, which are obliquely oriented to the axis of the muscle and span the interval between the subscapularis and supraspinatus tendons. The second layer is composed of tendon fibers grouped into large bundles that extend from the supraspinatus tendon and form a layer over the biceps tendon in its groove. The third layer contains tendon fascicles that are smaller in size and less tightly packed than the bundles in the second layer and also have less uniform orientation. The fourth layer is composed of loose connective tissue with thick bands of collagen fibers. This tissue merges with the coracohumeral ligament at the anterior edge of the supraspinatus. The fifth and last layer is the continued sheet of collagen fibrils that composes the superior joint capsule.

The footprint of the rotator cuff has been a subject of numerous cadaveric studies. While the rotator cuff muscles were often thought to run in parallel and insert onto discrete portions of the greater and lesser tuberosity, we now know that there is significant overlap of the tendinous portions of each rotator cuff onto the tuberosities. Clark and Harryman have demonstrated significant interdigitation of the supraspinatus and infraspinatus tendons occurring near the footprint ( Fig. 14-3 ). Minagawa et al. also demonstrated a significant overlap of the posterior supraspinatus with the infraspinatus cuff tendon. Mochizuki et al. studied 113 cadaveric specimens, and found that the portion of the greater tuberosity occupied by the supraspinatus insertion was much smaller than previously believed, and that the infraspinatus insertion occupied the preponderance of the footprint on the greater tuberosity.

The long head of the biceps tendon may be considered a functional part of the rotator cuff. It attaches to the supraglenoid tubercle of the scapula, runs between the subscapularis and the supraspinatus, exits the shoulder through the bicipital groove under the transverse humeral ligament, and attaches to its muscle in the proximal part of the arm. The coracohumeral ligament and the transverse humeral ligament keep the biceps tendon aligned in the groove. Although electromyographic studies on the long head of the biceps have demonstrated that it may be inactive during shoulder movements, it may serve as a passive restraint during most shoulder motions and contribute to anterior stability in abduction and external rotation. Changes in elbow position and loading of the biceps can lead to altered shoulder motion and muscle recruitment. This tendon also has the potential for guiding the head of the humerus as it is elevated, with the bicipital groove traveling on the biceps tendon like a monorail on its track. This mechanism helps explain why the humerus is capable of substantial rotation when it is adducted and allows very little rotation when it is maximally abducted (in which position the tuberosities are constrained as they straddle the biceps tendon near its attachment to the supraglenoid tubercle).
Coracoid and Coracoacromial Ligament
The coracoid arises from two or three ossification centers. It provides the medial attachment site for both the coracohumeral and coracoacromial ligaments. In that their muscle bellies lie medial to it, the neighboring supraspinatus and subscapularis tendons must be able to glide by the coracoid with their full excursion during shoulder movement. Scarring of one or both of these tendons to the coracoid can inhibit passive and active shoulder motion. Although the coracoid does not normally contact the anterior subscapularis tendon, forced internal rotation, particularly in the presence of a tight posterior capsule, can produce such contact as a result of the obligate translation.
The coracoacromial ligament spans from the undersurface of the acromion to the lateral aspect of the coracoid and is continuous with the less dense clavipectoral fascia. It forms a substantial part of the superficial aspect of the humeroscapular motion interface ( Figs. 14-4 and 14-5 ). This ligament may be thought of as the spring ligament of the shoulder in that it maintains the normal relationships between the coracoid and the acromion. Separation of these two scapular processes has been observed on sectioning this ligament in cadavers. Calcification in the acromial end of this ligament can give the appearance of a subacromial spur ( Fig. 14-6 ). Radiographic studies have associated the presence of such an acromial spur with full-thickness rotator cuff tears.



The coracoacromial arch is the inferiorly concave smooth surface consisting of the anterior undersurface of the acromion and the coracoacromial ligament. It provides a strong ceiling for the shoulder joint, along which the cuff tendons must glide during all shoulder movements (see Fig. 14-5 ). Passage of the cuff tendons and the proximal end of the humerus under this arch is facilitated by the subacromial-subdeltoid bursa, which is not normally a space, as often shown on diagrams, but rather two serosal surfaces in contact with each other, one on the undersurface of the coracoacromial arch and deltoid and the other on the cuff. These sliding surfaces are lubricated by the bursal surfaces and synovial fluid.
Scapular and Acromial Anatomy
The acromion is a scapular process arising from four separate centers of ossification: a basi-acromion, a meta-acromion, a meso-acromion, and a pre-acromion ( Fig. 14-7 ). The basi-acromion typically fuses to the scapular spine by 12 years of age, and the other centers of ossification are usually united by 22 years of age but may occur as late as 25 years.

When these centers fail to unite, the ununited portion is referred to as an os acromiale . The unfused segment directly anterior to the nonunion site defines the type of os acromiale. An unfused segment between the meso- and pre-acromion ossification centers is termed a pre-acromiale. The most common lesion is a failure of fusion of the meso-acromion to the meta-acromion. Most studies have reported the incidence of os acromiale to be approximately 2% to 8%, and they often occur bilaterally. It can be difficult to differentiate a fused os acromiale from a free ossicle in the clinical setting. Clinicians must be cautious about diagnosing and surgically treating an os acromiale before the age of 25 years given the potential for the ossification centers to unit. Resection of a large fragment creates a challenge for deltoid reattachment.
The notion that an os acromiale is a pathologic condition and the association of os acromiale with rotator cuff tears is controversial. Norris et al. and Bigliani et al. pointed to an association of cuff degeneration and an unfused acromial epiphysis. Boehm et al. assessed 62 patients with an os acromiale in the axillary radiographs of 1000 patients undergoing rotator cuff repair. The average number of tendons and average age was similar between those with and without the os acromiale. They concluded that the prevalence of os acromiale in patients with tears is comparable to that in a standard population with an unknown rotator cuff status. A subsequent MRI study found similar results.
Our understanding of the individual anatomy of the scapula and acromion and its association with rotator cuff pathology is continuing to evolve. Bigliani et al. described three acromial shapes: type I (flat) in 17%, type II (curved) in 43%, and type III (hooked) in 40%. In this study, 58% of the cadavers had the same type of acromion on each side. Thirty-three percent of the shoulders had full-thickness tears, 73% of which were seen in association with type III acromia, 24% with type II, and 3% with type I. The anterior slope of the acromion in shoulders with cuff tears averaged 29 degrees, slightly more than the slope of those without cuff tears, which averaged 23 degrees. The clinical significance of this relatively small difference is unknown. Numerous other authors have reported that patients with cuff defects are more likely to have hooked or angled acromia.
Subsequent studies of acromial morphology have not found the same relationships, and have suggested that the initial correlations between acromial morphology and cuff disease may be confounded by age. Nicholson et al. demonstrated in a review of 420 scapulae that spur formation on the anterior acromion was an age-related process such that persons younger than 50 years had less than one-fourth the prevalence of those older than 50 years. The status of the cuffs of these shoulders is unknown. A study by Gill et al. showed that in patients older than 50 years, no significant association between acromial morphology and rotator cuff pathology could be found. However, there was a significant correlation with age and rotator cuff tears. Older patients were more likely to have type II and III acromia, although this study did not comment on whether this trend was significant. These findings imply that apparent correlations between acromial morphology and rotator cuff tears may be exaggerated because of the confounding variable of age.
Other studies have suggested that type II and III acromia are acquired rather than developmental. Because most acromial hooks lie within the coracoacromial ligament ( Figs. 14-8 and 14-9 ), it seems likely that they are actually traction spurs in this ligament ( Fig. 14-10 ). The traction loads producing this hook can result from loading of the arch by the cuff, and may be increased with increasing dependency on the coracoacromial arch for superior stability in the presence of cuff degeneration. The concept of the hook as a traction phenomenon was first put forward by Neer more than 30 years ago. Because this hook lies within the ligament and points toward the coracoid (see Fig. 14-9 ), it seems unlikely that it would jeopardize passage of the cuff beneath the coracoacromial arch. Even in cases of cuff tear arthropathy, the undersurface of the coracoacromial arch commonly presents a smooth articulating concavity (see Fig. 14-10 and Fig. 14-11 ).




More recent radiographic and biomechanical studies have suggested that scapular morphology may predispose certain individuals to rotator cuff tears. Moor et al. performed a radiographic study that suggested that the individual anatomy of the scapula seen on plain radiographs is associated with degenerative rotator cuff tears. They found that the acromion index, the lateral acromion angle, and the “critical shoulder angle” (an angle that accounts for both glenoid inclination and lateral acromial extension) could accurately predict the presence of a degenerative cuff tear, but that acromial slope as described by Bigliani et al. was not predictive. A biomechanical study suggested that overloading of the supraspinatus tendon can occur with a higher critical shoulder angle. Considering all the available evidence, it seems most likely that loading of the coracoacromial arch over the years by the rotator cuff and proximal humerus can cause age-related calcification within the coracoacromial ligament. This intraligamentous calcification can present the radiographic appearance of an acromial “spur,” but because the calcification does not extend beyond the ligament, it does not “impinge” on the rotator cuff.
Our practice is to downplay the importance of the appearance of the acromion on radiographs and to perform acromial surgery only if there is a substantial prominence on the inferior aspect of the coracoacromial arch.
Humeroscapular Motion Interface
Matsen et al. described the humeroscapular motion interface as an articulation (see Figs. 14-4 and 14-5 ) between the cuff, the humeral head, and the biceps on the inside and the coracoacromial arch, the deltoid, and the coracoid muscles on the outside. Up to 4 cm of gliding can occur at this articulation in normal shoulders in vivo. It is instructive to consider the humeroscapular articulation as consisting of two concentric spheres, the glenohumeral sphere and the sphere represented by the articulation between the inferior surface of the coracoacromial arch and the proximal humeral convexity. Together, these two spheres enhance both shoulder stability and the surface available for scapulohumeral load transfer ( Fig. 14-12 ). Normally, the spheres of the humeral head and the coracoacromial arch share the same center. The difference in radius of the two spheres ( R and r in Fig. 14-12 ) is provided by the thickness of the rotator cuff, which serves as a spacer.

Contact beneath the coracoacromial arch is present in normal shoulders. Investigations have pointed out the importance of contact and load transfer between the rotator cuff and the intact coracoacromial arch in the function of normal shoulders, including the provision of superior stability against superiorly directed loads applied to the humerus. This backstop preventing superior translation of the proximal humerus is particularly important when upward loads are applied parallel to the face of the glenoid such as when the arms are used to press up from a seated lying position or in performing exercises like a bar dip.
Posterior capsular stiffness can lead to higher contact pressures under the subacromial arch. In the presence of posterior capsular tightness, shoulder flexion or internal rotation (or both) cause obligate anterosuperior translation of the humeral head and loss of the concentricity of the two spheres ( Fig. 14-13 ). As a result, the proximal humeral convexity is forced against the anterior undersurface of the concave coracoacromial arch rather than rotating concentrically beneath it (see Figs. 14-12 and 14-13 ). In the presence of degeneration of the cuff tendon, the shoulder can lose the concentricity of the humeral head and coracoacromial arch spheres ( Figs. 14-14 and 14-15 ). Taken together, these observations reinforce the shoulder’s need for normal posterior capsular laxity; a smooth, concentric, and congruent coracoacromial undersurface; and a normally thick and uniform cuff interposed between the humeral head and the coracoacromial arch.



Acromioclavicular Joint
Theoretically, osteophytes from the acromioclavicular joint may encroach on the space normally occupied by the cuff tendons ( Figs. 14-16 and 14-17 ). However, these spurs are usually too medial to contact the tendons. In a series of 47 patients with arthrographically confirmed supraspinatus tendon rupture, Peterson and Gentz found that 51% had distally pointing acromioclavicular joint osteophytes. A similar incidence was found in their series of 170 autopsy specimens with cuff defects. The incidence of distally pointing acromioclavicular osteophytes in normal shoulders was 14% in the clinical studies and 10% in the cadaver studies. It is recognized, however, that degenerative changes in the cuff and degenerative changes in the acromioclavicular joint can coexist in the aging population without the former being causally related to the latter. Steinbeck et al. pointed out in their retrospective study of 102 patients in whom cuff repair was performed with resection of the lateral end of the clavicle in 20, that the results were not significantly influenced by the clavicle resection. Again, it is recognized that acromioclavicular osteophytes are usually sufficiently medial that they do not jeopardize the cuff.


Vascular Anatomy
The vascular anatomy of the cuff tendons has been described by numerous investigators. Lindblom described an area of relative avascularity in the supraspinatus tendon near its insertion. Rothman and Parke found contributions to the cuff vessels from the suprascapular, anterior circumflex, and posterior circumflex arteries in all cases. The thoracoacromial artery contributed to the cuff’s blood supply in 76%, the suprahumeral in 59%, and the subscapular in 38%. These authors found the area of the supraspinatus just proximal to its insertion to be markedly under vascularized in comparison to the remainder of the cuff. Recent studies have suggested an age-related decrease in vascularity that is consistent with the increasing prevalence of cuff pathology with aging. Uhthoff et al. observed relative hypovascularity of the deep surface of the supraspinatus insertion as compared with its superficial aspect. Nixon and DiStefano suggested that the critical zone of Codman corresponds to the area of anastomoses between the osseous vessels (the anterolateral branch of the anterior humeral circumflex and the posterior humeral circumflex) and the muscular vessels (the suprascapular and subscapular vessels).
An additional anatomic feature of importance is the acromial branch of the thoracoacromial artery. This artery is closely related with the coracoacromial ligament, and is often transected in the course of acromioplasty and section of the coracoacromial ligament. This artery is invariably encountered during arthroscopy if sectioning of the coracoacromial ligament is performed.
Biomechanics of the Rotator Cuff
The rotator cuff musculature may be thought of as having three functions: rotation of the humerus with respect to the scapula, muscular balance, and compression of the head into the concavity of the glenoid fossa. This concavity compression concavity mechanism is important in providing glenohumeral stability through range of motion of the shoulder in the many functional positions where the capsular ligaments are lax.
The concept of the rotator cuff “force couple” has been described in the coronal and transverse planes. In the coronal plane, the pull of the deltoid must be balanced with the inferior portion of the rotator cuff including the infraspinatus, teres minor, and subscapularis. This couple will be balanced only if the line of action of the inferior cuff is below the center of rotation of the humeral head to oppose the deltoid moment. The transverse force couple is composed anteriorly of the subscapularis and posteriorly of the infraspinatus and teres minor ( Fig. 14-18 ). Clinically, these force couples are important in the treatment of massive rotator cuff tears in which the goal is not necessarily to repair all torn tendons to the cuff insertion but rather to balance the coronal and transverse force couples so that the humeral head is stable within the glenoid concavity and a stable fulcrum is maintained throughout range of motion. Without adequate stability imparted by the force couples, the power of the deltoid muscle cannot be used efficiently for elevation of the shoulder.

This concept of force couples around the shoulder is important but may oversimplify the complex interactions between the cuff and the different heads of the deltoid, trapezius, latissimus dorsi, and pectoralis muscles. The timing and magnitude of these balancing muscle effects must be precisely coordinated to avoid unwanted directions of humeral motion. In a specified position, activation of a muscle creates a unique set of rotational moments. For example, the anterior deltoid can exert moments in forward elevation, internal rotation, and cross-body movement ( Fig. 14-19 ). If forward elevation is to occur without rotation, the cross-body and internal rotation moments of this muscle must be neutralized by other muscles, such as the posterior deltoid and infraspinatus ( Fig. 14-20 ). As another example, use of the latissimus dorsi in a movement of pure internal rotation requires that its adduction moment be neutralized by the superior cuff and deltoid. Conversely, use of the latissimus dorsi in a movement of pure adduction requires that the posterior cuff and posterior deltoid muscles neutralize their internal rotation moment. Thus the simplified view of muscles as isolated motors, or as members of force couples, must give way to an understanding that all shoulder muscles function together in a precisely coordinated way, with opposing muscles canceling out undesired elements and leaving only the net torque that is needed to produce the desired action. This degree of coordination requires a preprogrammed strategy of muscle activation that must be established before the motion is carried out. The rotator cuff muscles are critical, but not the only, elements of this shoulder muscle balance equation.


The humeral torque resulting from contraction of a cuff muscle is determined by the moment arm (the distance between the effective point of application of this force and the center of the humeral head) and the component of the muscle force that is perpendicular to it ( Fig. 14-21 ). The magnitude of force that can be delivered by a cuff muscle is determined by its size, health, and condition, as well as the position of the joint. The cuff muscles’ contribution to shoulder strength has been evaluated by Colachis et al., who used selective nerve blocks and found that the supraspinatus and infraspinatus provide 45% of the abduction and 90% of the external rotation strength. Howell et al. found that the supraspinatus and deltoid muscles are equally responsible for producing torque about the shoulder joint in the functional planes of motion. Generation of force in abduction of the arm decreased only 5% after a simulated tear of one-third and two-thirds of the supraspinatus. Detachment of the entire tendon resulted in a 17% loss of strength. Simulation of retraction of tears of one-third, two-thirds, and the entire supraspinatus resulted in losses of 19%, 36%, and 58%, respectively. These losses were almost completely regained with side-to-side repair. Other estimates of the relative contributions of the rotator cuff to shoulder strength have been published.

Bey et al. found that the strain within the rotator cuff increased in positions of greater abduction, even when the same force was applied through the rotator cuff. No significant difference in strain on the superficial and deep portions of the cuff was detected. The compressive stiffness of the articular side of the supraspinatus tendon was found to be significantly less than that of its bursal side. This difference in stiffness might be one reason that most tears begin on the articular surface.
At least three factors complicate analysis of the contribution of a given muscle to shoulder strength. The moment arms of each rotator cuff muscle vary with the increasing angles of elevation and abduction, and therefore the torque that a muscle can generate varies with joint position. Muscles are usually stronger near the middle of their excursion and weaker at the extremes ( Figs. 14-22 to 14-24 ). The direction of a given muscle force is determined by the position of the joint. For example, the supraspinatus can contribute to abduction or external rotation, or both, depending on the initial position of the arm. The effective humeral point of application for a cuff tendon wrapping around the humeral head is not its anatomic insertion but rather at the point where the tendon first contacts the head, a point that usually lies on the articular surface ( Fig. 14-25 ).




Early studies reported that the rotator cuff, specifically the supraspinatus, contributed most to abduction of the arm at low abduction angles, and that cuff contribution was insignificant at higher abduction angles. However, subsequent data have demonstrated that the supraspinatus and infraspinatus have smaller torque in the scapular plane than previously reported. A significant increase in the middle deltoid torque is required to initiate abduction in the presence of supraspinatus paralysis, but glenohumeral kinematics and motion are not affected when the transverse force couple is intact. The subscapularis and infraspinatus are not only internal and external rotators of the glenohumeral joint but also play a role in elevation in the plane of the scapula. The subscapularis enhances abduction in external rotation while the infraspinatus enhances abduction in internal rotation.
The concept of the “rotator cable” and “suspension bridge” should be considered when evaluating the biomechanics of a shoulder with a rotator cuff tear. A thickening of tissue seen on the articular side of the rotator cuff that runs in a crescent shape was originally described as a “transverse band” by Clark and Harryman and termed the “rotator cable” by Burkhart et al. ( Fig. 14-26 ). This thickening of tissue runs from within the anterior supraspinatus at the biceps groove towards the posterior infraspinatus near the middle facet of the greater tuberosity. The “suspension bridge” concept proposes a protective role of the rotator cable in which the free margins of the tear correspond to the supports of the cable ( Fig. 14-27 ). A tear that occurs within the crescent tissue more lateral to the cable should be shielded from stress as long as the thicker cable tissue is intact. In these tears, the function of the superior cuff would still be intact due to distribution along the length of the cable of the suspension bridge.


Consideration of the biomechanics of the rotator cuff is not limited to the glenohumeral joint but must also include the complex interaction between several other articulations, including the scapulothoracic, acromioclavicular, and sternoclavicular joints. The humeroscapular interface is of particular importance. Smooth, unrestricted motion must occur at this articulation, which consists of the proximal humeral convexity formed by the tuberosity and rotator cuff on one side and the inferior surface of the coracoacromial arch. Because the scapula is the origin of the rotator cuff muscles and the deltoid, it serves as the base for glenohumeral motion.
Nerve lesions, muscle damage, fatigue, or weakness can lead to dyskinetic movement of the scapula on the chest wall. This can lead to a mechanical disadvantage for the cuff muscles, and treatment that involves strengthening the scapular stabilizers can improve rotator cuff function. The scapula is the origin of the cuff musculature, and is supported and stabilized only by muscular and ligamentous (acromioclavicular and coracoclavicular) attachments. The position of the scapula is controlled by scapulothoracic muscles, including the trapezius, levator scapulae, serratus anterior, pectoralis minor, and rhomboids. Fatigue of these stabilizers can lead to inability of the scapula to rotate, subsequently preventing the acromion from clearing when the arm is elevated. Patients treated for scapular dyskinesia with strengthening of the scapular stabilizers can show improvement in rotator cuff mechanics.
Basic Science of Cuff Tendon and Healing
Only recently has the basic science behind rotator cuff pathogenesis and healing been investigated. Much of the focus has been on the tendon-to-bone insertion of the cuff, and recent advances and innovation in molecular biology have allowed us to investigate biologic changes that occur during the progression of disease as well as during the healing process. Numerous animal models have been utilized to study healing of this particular enthesis.
Tendon Composition and Structure
The rotator cuff tendons are dense fibrous connective tissue structures with approximately 85% of its dry weight composed of collagen. Of the various collagen types, the majority (95%) is type I with lower levels of type III collagen. Collagen molecules in tendon typically consist of a triple-chain helix composed of a characteristic tripeptide sequence of glycine, proline, and hydroxylproline. Covalent intramolecular and intermolecular cross-links are produced by the action of various enzymes—this process is the key characteristic that is responsible for the high tensile strength of tendons. Collagen molecules are aligned with the longitudinal axis of the tendon to form a collagen fibril, which is the basic structure of tendons. The multiunit hierarchical structure of the tendon proceeds from a collagen fibril to a collagen fiber to a tendon bundle and a tendon fascicle that is bound by endotenon. Tendon fascicles are then grouped to form the tendon unit ( Fig. 14-28 ).

The predominant cell types in tendon are tendon fibroblasts, or tenocytes, which are elongated spindle-shaped cells that are located between the collagen bundles. These fibroblasts have prominent cytoplasmic processes that allow for a complex three-dimensional structural network and cell-to-cell communication. This may be important in the tendon’s ability to change structure, composition, and mechanical properties in response to mechanical loading. When mechanically loaded, the tendon cells can alter their gene expression and protein synthesis, which subsequently leads to changes in the composition of the extracellular matrix proteins. These cellular mechanisms for mechanotransduction are still being elucidated.
Between these collagen fibers and tendon fibroblasts are numerous noncollagenous matrix components. This extracellular matrix plays a crucial role in the ability of the tendon to transfer large loads from muscle to bone. The matrix/cell ratio in tendon is very high when compared with that in other musculoskeletal tissues. An integral part of the extracellular matrix of tendon is proteoglycans, which are glycoproteins composed of glycosaminoglycan chains. These chains are important for maintaining the hydration and viscoelastic behavior of the tendon. Larger proteoglycans such as versican and aggrecan hold water within the tendon and are particularly important at the transition of tendon to bone. Small proteoglycans such as decorin and biglycan, which are members of the small leucine-rich proteoglycan family, play key roles in the biologic activity and remodeling of tendons.
The tendon-bone junction, or enthesis, is a complex area of transition that plays a very important role in transferring load from muscle to bone. The rotator cuff insertion is a direct fibrocartilaginous structure in which the tissue composition changes through a transition of four zones ( Fig. 14-29 ). The first zone is similar to the midsubstance of the tendon, and is primarily composed of well-aligned type I collagen fibers. In the transition to the second and third zones, the collagen type changes to fibrocartilage composed of types II and III collagen to mineralized fibrocartilage composed predominantly of type II and X collagen. The last zone is primarily bone and contains type I collagen and minerals. Because tendon and bone have significantly different mechanical properties, this gradual transition and change in collagen composition is crucial in dissipating mechanical load as stress is applied to the insertion site. These specialized transition zones are typically not recreated after a rotator cuff repair, and therefore the strength and durability of the repair does not emulate the native enthesis.

Biomechanical Properties
The rotator cuff must be able to transmit large forces with minimal deformation. The biomechanical characteristics of a tendon can be described by its structural and material properties. Structural properties refer to the tensile capacity of the overall structure, and are dependent on the size and shape of the tendon. Material properties refer to the intrinsic quality of the tendon itself, and therefore characterize the tendon independent of size and shape. The structural properties of a tendon are represented by a load displacement curve ( Fig. 14-30 ), while the material properties are best described by their stress-strain relationship ( Fig. 14-31 ). The slope between two defined points of elongation on a load displacement curve represents the stiffness. The slope between two limits of strain represents the elastic modulus of the tendon.


An important characteristic of tendon is its viscoelastic nature. Viscoelastic properties allow for smooth movement during normal joint motion. This characteristic is dependent on the extracellular composition of the tendon. Hysteresis is a viscoelastic characteristic that represents energy that is dissipated during cyclic loading of tendon.
A fundamental biologic response of living musculoskeletal tissue is the ability of the cells to respond to mechanical loading. As load is transferred from the muscle unit through the tendon to bone, the tendon senses external force and will adjust its biomechanical properties in a coordinated response. Physiologic loads are typically healthy and are required to maintain tendon homeostasis. Excessive, repetitive loading or excessive immobility can both have detrimental effects on the structural and material properties of tendon; repetitive loading can result in a catabolic response leading to tendinopathy. This also becomes particularly important when we consider the benefits of controlled loading in prescribing rehabilitation after rotator cuff repair. Determining the optimal magnitude, frequency, and duration of controlled loading has been the focus of numerous animal studies on the pathogenesis of tendinopathy and the healing response after tendon repair.
Tendon Healing
The local environment of the tendon dictates its healing potential. Because rotator cuff tendons are intrasynovial, they do not undergo the spontaneous healing that can be seen in extrasynovial tendons such as the Achilles tendon.
Healing of tendon or ligament typically progresses through three phases: an inflammatory phase that lasts a few days, a proliferative phase that lasts a few weeks, and then a longer remodeling phase that lasts months. In the first phase, inflammatory cells release cytokines and growth factors that lead to recruitment of tendon fibroblasts that proliferate and produce, deposit, and crosslink collagen fibrils. Despite some remodeling, the resultant collagen scar is relatively disorganized and fails to replicate the normal zonal transition at the tendon-bone insertion site.
Modulation of the healing environment has the potential to improve healing rates. The primary targets are the biologic environment and the mechanical environment. Biologic factors can be used to stimulate healing at the time and after surgical repair. Basic fibroblast growth factor can improve angiogenesis and matrix synthesis. Transforming growth factor-β (TGF-β), various bone morphogenetic proteins, vascular endothelial growth factor, and platelet-derived growth factor are all involved in the healing response. Much effort has gone into investigating methods of modulating these growth factors in order to convert a typically reparative scar-forming process to the regenerative scarless healing process that is seen in fetal tendon healing. Additionally, numerous tissue engineering approaches have sought to improve cuff healing using various types of aligned scaffolds aimed at delivering biofactors or promoting cellular ingress to the tendon healing site.
The mechanical environment can be affected by modifying the activity level of the shoulder postoperatively, and has been studied in many in vitro and animal model studies. Because cuff healing is a mechanosensitive process, it can be altered by adjusting the mechanical load to the repair site. Tendons have biochemical and biomechanical properties that respond and adjust to mechanical loading. Appropriate exercise-related loading can enhance tendon mechanical properties, while repetitive overuse or complete load removal from tendon can lead to deterioration.
When mechanical load is imparted onto a tendon, biochemical changes within tenocytes are evident, along with increased collagen deposition and upregulation of growth factors important in collagen synthesis. Expression of TGF-β and scleraxis, both of which are involved in differentiation and proliferation, is altered with mechanical load. On the other hand, chronic, repetitive loads on tendons (overuse/overload) can lead to microtrauma and the production of inflammatory mediators such as PGE 2 and LTB 4 , which causes further tendon degeneration and soft tissue edema. The magnitude of load is also important; repetitive mechanical load can have two opposite effects that are dependent on load magnitude. A smaller magnitude decreases MMP-1, COX-2, and IL-1β and has an anti-inflammatory effect, while the opposite is seen with larger magnitudes.
Disuse and immobilization can decrease the stiffness and tensile strength of tendons. Stress deprivation at the repair site induces a catabolic state. mRNA expression of collagenase (MMP-1) is markedly increased in load-deprived tendon cells but significantly inhibited with increasing load to the tendon cells. This suggests that upregulation of MMP-1 expression can be inhibited through a cytoskeletal-based mechanotransduction pathway. A subsequent study by the same group has suggested that load deprivation decreases the mechanoresponsiveness of tendon cells.
Classification of Rotator Cuff Pathology
The requisites for normal cuff function are stringent, and include healthy, strong cuff muscles, normal capsular laxity, intact cuff tendons, a smooth contour of the undersurface of the coracoacromial arch, a thin, lubricating bursa, a smooth upper surface of the cuff and tuberosities, and concentricity of the glenohumeral and cuff-coracoacromial spheres of rotation. Disorders of this complex mechanism are the most common source of shoulder problems.
Rotator cuff pathology spans a spectrum of severity that includes rotator cuff tendinopathy, partial-thickness tears, full-thickness tears, and rotator cuff arthropathy. Physical examination can assist in making the diagnosis, but oftentimes, advanced imaging is required to distinguish tendinosis, partial-thickness tears, and small full-thickness tears—all of which can cause anterolateral shoulder pain.
The terms rotator cuff “tendinitis” and “tendinopathy” are often used interchangeably to describe a broad range of painful conditions of the shoulder. The term “tendinitis” implies inflammation and may be misleading however, because numerous studies have shown that little inflammation is actually present in these syndromes. Symptoms related to acute bursitis or tendinitis may respond well in the short term to oral or local antiinflammatories. However, there is no clear evidence that antiinflammatory medications can reliably improve symptoms related to more chronic “tendinitis,” indicating that the terms “tendinosis” or “tendinopathy” may be more appropriate in this setting. Treatment should be focused on therapeutic exercises that can relieve pain and improve function.
Numerous different classification systems have been devised to try to better describe rotator cuff pathology. Neer initially described three different stages of cuff lesions :
- •
Stage 1: Reversible edema and hemorrhage are present in a patient younger than 25 years.
- •
Stage 2: Fibrosis and tendinitis affect the rotator cuff of a patient typically in the 25- to 40-year-old age group. Pain often recurs with activity.
- •
Stage 3: Bone spurs and tendon ruptures are present in a patient older than 40 years.
Neer’s original classification of rotator cuff pathology has been subsequently modified by many other classification systems to include the structural integrity of the tendon and also to differentiate between articular-sided partial-thickness tears, bursal-sided partial-thickness tears, and intrasubstance delamination. As imaging techniques and technology advanced with time, classification systems began incorporating more tear characteristics, including tear size, tear shape, and muscular atrophy.
Partial-Thickness Tears
A commonly referenced classification system for partial-thickness tears was described by Ellman, and is based on tear location, depth, and area ( Table 14-1 ) ; this is considered a subclassification of the stage 3 cuff lesion described by Neer. Grade 1 involves less than 3 mm of tendon or a quarter of the footprint thickness, grade 2 involves 3 to 6 mm or less than half of the footprint thickness, and grade 3 involves greater than 6 mm of the footprint. Tears are also divided into articular sided, bursal sided, or interstitial.
Location | Grade | Size | |
---|---|---|---|
A | Articular | 1 | <3 mm |
B | Bursal | 2 | 3-6 mm |
C | Interstitial | 3 | >6 mm |
Snyder et al. devised a more detailed classification system based on arthroscopic findings. In this system, A, B, and C represent articular-sided, bursal-sided, and complete tears. Grade 0 represents a normal rotator cuff, grade 1 is superficial fraying less than 1 cm in size, grade 2 involves fraying 1 to 2 cm in size, grade 3 is tissue disruption in 2 to 3 cm of tendon, and grade 4 is a substantial cuff tear that involves a sizable flap and more than one tendon.
Habermayer et al. also developed a classification for articular-sided tears based on arthroscopic findings that not only accounts for size in the coronal plane but also accounts for sagittal tear extension. In this classification, type A tears involve the coracohumeral ligament up to the medial border of the supraspinatus, type B tears are isolated within the crescent tissue, and type C tears extend from the lateral border of the pulley system over the medial border of the supraspinatus to the crescent tissue.
Despite the numerous types of classifications for partial-thickness tears, studies have demonstrated poor interobserver reliability in distinguishing tears that are greater than and less than 50% using both MRI and arthroscopic examinations. Interobserver reliability for distinguishing full-thickness from partial-thickness tears in these studies was much higher.
Full-Thickness Tears
There are numerous classification schemes of full-thickness tears, all of which are extremely diverse in their attempts to describe the tear size, number of tendons involved, tear shape, chronicity, tendon quality, and muscle atrophy.
Tear Size/Number of Tendons Torn
One of the most commonly used classifications for the size of full-thickness rotator cuff tears was described by DeOrio and Cofield. Groups are stratified by tear size from anterior to posterior: less than 1 cm is small, 1 to 3 cm is medium, 3 to 5 cm is large, and more than 5 cm is massive.
A classification system described by Harryman et al. is based on the size and number of tendons involved. Stage 0 is an intact rotator cuff, stage IA is a partial-thickness tear of the supraspinatus, and stage IB is a full-thickness tear involving only the supraspinatus. Stage II includes the supraspinatus and a portion of the infraspinatus. Stage III includes the supraspinatus, infraspinatus, and subscapularis tendons. Stage IV is used to describe rotator cuff arthropathy.
Tear Shape
A classification developed by Ellman and Gartsman attempted to characterize tears by their three-dimensional shapes. This classification includes crescent, reverse L , L -shaped, trapezoidal, and massive tears ( Fig. 14-32 ).

Burkhart also described a classification system based on tear pattern. Type 1 tears are crescent tears that are short on the coronal image but wide on the sagittal image. Type 2 tears are U – or L -shaped tears that are wide on the coronal image and short on the sagittal image. Type 3 tears are massive tears that are wide on both coronal and sagittal imaging. Type 4 tears are massive tears with concomitant glenohumeral arthrosis. The advantage of this classification is the potential for guiding repair technique: type 1 tears may benefit most from tendon-to-bone repair, while type 2 tears may benefit from margin convergence.
Patte Classification
Patte developed a complex and comprehensive classification system that incorporates both tear size coronal and sagittal imaging, tendon retraction, muscle atrophy, and integrity of the long head of the biceps tendon ( Box 14-1 ). Topography in the frontal plane is grouped into three stages ( Fig. 14-33A ): stage 1 is minimal retraction, stage 2 is tendon retraction to the level of the humeral head, and stage 3 is tendon retraction at the level of the glenoid. In the sagittal plane, tears were grouped into six segments (see Fig. 14-33B and 14-33C ).
1. Extent of Tear
Group I: Partial tears and full-thickness tears <1 cm in sagittal diameter
A: Deep, partial tears
B. Superficial tears
C. Small, full substance tears
Group II: Full substance tears of the entire supraspinatus
Group III: Full substance tears involving more than one tendon
Group IV: Massive tears with secondary osteoarthritis
2. Topography of Tear in Sagittal Plane
Segment 1: Subscapularis tear
Segment 2: Coracohumeral ligament tear
Segment 3: Isolated supraspinatus tear
Segment 4: Entire supraspinatus and half of infraspinatus tear
Segment 5: Entire supraspinatus and infraspinatus tear
Segment 6: Subscapularis, supraspinatus, and infraspinatus tear
3. Topography of Tears in Frontal Plane
Stage 1: Proximal stump close to bony insertion
Stage 2: Proximal stump at level of humeral head
Stage 3: Proximal stump at level of glenoid
4. Trophic Quality of Muscle of Torn Tendon
5. State of Long Head of Biceps

Muscle Atrophy and Fatty Infiltration
The most common reference for classification of fatty infiltration of the rotator cuff musculature is that described by Goutallier et al. Advanced muscle atrophy typically indicates chronic retraction of a tear, which has been shown to be of prognostic importance and should be considered in all rotator cuff tears. Table 14-2 shows the successive stages of fatty infiltration that were originally described by Goutallier on a sagittal CT cut that incorporates the tip of the coracoid and the inferior glenoid. This classification has since been adapted for use on T1 sagittal oblique imaging on MRI, and Fuchs has simplified the original Goutallier classification to three categories by combining grades 0 and 1 as normal and grades 3 and 4 as advanced degeneration.
Grade 0 | Normal muscle without fatty streaks |
Grade 1 | Muscle has some fatty streaking |
Grade 2 | Fatty infiltration present, but less than muscle |
Grade 3 | Equal amount of fat and muscle tissue |
Grade 4 | More fat than muscle |
Two other methods have been described to characterize the amount of muscle atrophy. Zanetti’s “tangent sign” is a simple binary method of defining atrophy. A line is drawn from the superior border of the coracoid to the superior border of the scapular spine ( Fig. 14-34 ). If the muscle belly of the supraspinatus fails to intersect this line; the “tangent sign” is positive and indicates significant muscular atrophy. Thomazeau et al. described an “occupation ratio” that takes into account the cross-sectional area of the muscle belly in relation to the size of the fossa. This measurement is performed on a sagittal oblique cut at the level of the medial border of the coracoid process. An occupation ratio between 0.6 and 1.0 is considered normal or indicates slight atrophy; 0.4 to 0.6 indicates moderate atrophy; and less than 0.4 indicates severe atrophy.

Subscapularis Tears
A grading system specific for subscapularis tears was described by LaFosse et al. Type I involves a partial-thickness tear of the superior third of the subscapularis, type II involves a complete tear of the superior one-third, type III involves a complete tear of the superior two-thirds, type IV is a complete tear of the entire tendon with a well-centered head and less than 50% fatty infiltration, and type V is a complete tear with significant fatty infiltration.
Clinical Evaluation and Physical Examination
A comprehensive history is the first and arguably the most important aspect of a complex decision-making process in evaluating a patient with a suspected rotator cuff tear. It will form the foundation of any ongoing diagnostic and therapeutic decisions.
It is now recognized that rotator cuff tears—even ones of substantial size—can be asymptomatic. Furthermore, it is known that the amount of shoulder discomfort experienced by the patient is not related to the size of the tear. Pain may not be the primary symptom of rotator cuff failure, which may also produce weakness, stiffness, crepitus, or instability.
The patient’s physiologic and chronologic age should be considered throughout history and examination. Degenerative tearing typically occurs in older patients, while a greater injury is required to tear the cuff of persons at the younger end of the age distribution. Traumatic glenohumeral dislocations in persons older than 40 years have a strong association with rotator cuff tears. Age is thought to be a strong predictor of rotator cuff healing if operative intervention is a consideration—older patients are less likely to achieve a durable repair.
Chronicity is one of many important tear characteristics that have an impact on healing potential. Acute rotator cuff tears from a distinct injury causing weakness often do well, and have good healing potential with early surgery. In these patients, the acute component of their shoulder pain must be differentiated by history and imaging from more chronic symptoms that may have preceded the injury. More often, patients who present with a painful shoulder problem have endured their symptoms for months or years as a result of cuff degeneration rather than injury.
Activity level and expectations of treatment are an essential part of the overall decision-making process and discussion with the patient. An inactive patient without high functional demands will likely be able to perform activities of daily living without difficulty even with a full-thickness rotator cuff tear treated nonoperatively. On the other hand, even a small full-thickness tear could present difficulties to a young laborer with high functional demands who requires overhead strength. Hand dominance, smoking, genetic predisposition, medical comorbidities, and social factors affecting rehabilitation after potential surgery are variables that should be taken into account when deciding between nonoperative and operative care.
In all patients, prior treatment including physical therapy, injections, and previous surgery should be noted. Also, any neck pain, numbness and tingling in the arm, symptoms radiating below the elbow, or medial scapular pain may be a sign of cervical radiculopathy. In these patients, a comprehensive cervical spine examination should follow.
Physical Examination
A standardized physical examination should include inspection, range of motion, and strength testing in order to assess the various clinical manifestations of cuff disease including stiffness, weakness, instability, and roughness. An especially relevant point in the physical examination is an accurate assessment of the strength of the rotator cuff musculature, deltoid muscle, and scapular stabilizers. This physical assessment often forms the basis of any ongoing management program.
The patient’s shoulder should be exposed for inspection and palpation. If cuff dysfunction has been present for more than a few months, it may be accompanied by supraspinatus and infraspinatus muscle atrophy. Subtle atrophy can be seen most easily by casting a shadow from a light over the patient’s head. Clear visualization of the supraspinatus and infraspinatus fossae can be a distinct sign of chronic atrophy. Additionally, the location of any surgical incisions can help the examiner determine the nature of any previous surgeries, particularly if the patient’s history of previous shoulder surgery was remote.
Crepitus should be noted with palpation of the shoulder during range of motion. Roughness associated with cuff disease is manifested as symptomatic crepitus on passive glenohumeral motion. Bursal hypertrophy ( Fig. 14-35 ), adhesions ( Fig. 14-36 ), secondary changes in the undersurface of the coracoacromial arch, loss of integrity and smoothness of the upper aspect of the cuff tendons ( Figs. 14-37 and 14-38 ), and uncovering of the tuberosities ( Fig. 14-39 ) can all contribute to subacromial abrasion. Crepitus from subacromial abrasion is easily detected by placing the examiner’s thumb and fingers on the anterior and posterior aspects of the acromion while the humerus is moved relative to the scapula ( Figs. 14-40 and 14-41 ). Because many shoulders demonstrate asymptomatic subacromial crepitus, it is important during the examination to ask whether the crepitus noted by the examiner reproduces the patient’s complaints.







Tendon defects can also be assessed by palpation of the shoulder. As pointed out by Codman, defects in the cuff can often be palpated by rotating the proximal end of the humerus under the examiner’s finger placed at the anterior corner of the acromion. The perimeters of the divot left by a defect in the supraspinatus are particularly easy to palpate. The defect is usually just posterior to the bicipital groove and medial to the greater tuberosity ( Figs. 14-42 to 14-44 ).



Limitations in passive and active range of motion should be documented. Restriction in motion due to arthritis or adhesive capsulitis needs to be ruled out. Stiffness often presents as a result of partial-thickness cuff lesions, but it can also be associated with full-thickness cuff defects. Stiffness may be demonstrable as limitations in the following:
- •
Flexion—degrees from the neutral position ( Fig. 14-45 )
FIGURE 14-45
Limited forward elevation. A, Tightness of the posteroinferior capsule can limit glenohumeral forward elevation, also known as flexion. B, Tight portion of the capsule.
(From Matsen FA III, Lippitt SB. Shoulder Surgery: Principles and Procedures . Philadelphia: Saunders, 2004;50.)
- •
External rotation—degrees from the neutral position ( Fig. 14-46 )
FIGURE 14-46
Limited external rotation in adduction. A, Tightness of the anterior-superior capsule and coracohumeral ligament can limit external rotation of the arm at the side. B, Tight portion of the capsule.
(From Matsen FA III, Lippitt SB. Shoulder Surgery: Principles and Procedures . Philadelphia: Saunders, 2004:52.)
- •
Internal rotation reaching up the back—posterior segment reached with thumb ( Fig. 14-47 )
FIGURE 14-47
Limited internal rotation. A, Tightness of the posterior-superior capsule can limit glenohumeral internal rotation. B, Tight portion of the capsule.
(From Matsen FA III, Lippitt SB. Shoulder Surgery: Principles and Procedures . Philadelphia: Saunders; 2004:51.)
- •
External rotation in abduction—degrees from neutral position ( Fig. 14-48 )
FIGURE 14-48
Limited external rotation in abduction. A, Tightness of the anterior-inferior capsule can limit external rotation of the arm in abduction. B, Tight portion of the capsule.
- •
Internal rotation in abduction—degrees from neutral position ( Fig. 14-49 )
FIGURE 14-49
Limited internal rotation in abduction. A, Tightness of the posterior-inferior capsule can limit internal rotation of the abducted arm. B, Tight portion of the capsule.
(From Matsen FA III, Lippitt SB. Shoulder Surgery: Principles and Procedures . Philadelphia: Saunders; 2004:55.)
- •
Cross-body adduction—centimeters from the ipsilateral antecubital fossa to the contralateral acromion or coracoid ( Fig. 14-50 ).
FIGURE 14-50
Limited cross-body adduction. A, Tightness of the posterior capsule can limit adduction of the arm across the body. B, Tight portion of the capsule.
(From Matsen FA III, Lippitt SB. Shoulder Surgery: Principles and Procedures . Philadelphia: Saunders; 2004:53.)
Although patients with full-thickness cuff defects might still retain the ability to actively abduct the arm, significant tendon fiber failure is usually manifested by weakness on manual muscle testing. Isometric testing of muscle strength prevents confusion with symptoms that can arise from shoulder movement (such as those associated with subacromial abrasion). Although individual cuff muscles are difficult to specifically isolate given the interdigitation of their tendon insertions, the following isometric tests are reasonably selective:
- •
Supraspinatus: Isometric elevation of the arm held in 90 degrees of elevation in the plane of the scapula and in mild internal rotation ( Fig. 14-51 )
FIGURE 14-51
Supraspinatus test. The supraspinatus is tested by positioning the arm in 90 degrees of abduction in the plane of the scapula and in slight internal rotation; this puts the supraspinatus tendon on top of the humeral head. The patient holds this position while the examiner attempts to adduct the arm.
(From Matsen FA III, Lippitt SB. Shoulder Surgery: Principles and Procedures . Philadelphia: Saunders; 2004:286.)
- •
Infraspinatus: Isometric external rotation of the arm held at the side in neutral rotation with the elbow flexed to 90 degrees ( Fig. 14-52 )
FIGURE 14-52
Infraspinatus test. The infraspinatus is tested by positioning the humerus with the elbow at the side and the forearm pointing straight ahead. The patient holds this position while the examiner attempts to push the arm into internal rotation.
(From Matsen FA III, Lippitt SB. Shoulder Surgery: Principles and Procedures . Philadelphia: Saunders; 2004:287.)
- •
Teres minor: Isometric external rotation of the arm in 90 degrees of abduction with the elbow flexed to 90 degrees ( Fig. 14-53 )
FIGURE 14-53
Teres minor test. The teres minor is tested by positioning the arm in 90 degrees of abduction in the plane of the scapula and in 90 degrees of external rotation. Inability to hold this position is considered a positive test result.
- •
Subscapularis: Isometric internal rotation of the arm with the elbow flexed to 90 degrees and the hand pressing in toward the stomach ( Fig. 14-54 ); alternatively, isometric internal rotation of the arm with the back of the hand on the lumbar area ( Fig. 14-55 )
FIGURE 14-54
Subscapularis test. The subscapularis is tested by having the patient press the hand in toward the stomach.
(From Matsen FA III, Lippitt SB. Shoulder Surgery: Principles and Procedures . Philadelphia: Saunders; 2004:287.)
FIGURE 14-55
Lift-off test. The subscapularis can also be tested by having the patient push the hand away from the lumbar area posteriorly against resistance.
(From Matsen FA III, Lippitt SB. Shoulder Surgery: Principles and Procedures . Philadelphia: Saunders; 2004:287.)
These simple manual tests are helpful for characterizing the size of the tendon defect, from single tendon tears involving only the supraspinatus to two-tendon tears involving the supraspinatus and infraspinatus, as well as three-tendon tears involving the subscapularis. Some have suggested that weakness from pain inhibition can be distinguished from weakness from a tendon defect by subacromial injection of a local anesthetic. It is important to keep in mind, notes Kibler et al., that apparent supraspinatus weakness on clinical examination can depend on scapular position. The weakness can result from other factors besides supraspinatus muscle weakness, such as a lack of a stable base in the kinetic chain or scapula.
Differential Diagnosis
Traditionally, it has been stated that rotator cuff tears must be differentiated from cuff tendinitis and bursitis , and that tests such as arthrography and ultrasonography are necessary to make this distinction. Perhaps a more realistic view is that many of the symptoms often attributed to tendinitis and bursitis are, in actuality, episodes of acute fiber failure that are not clinically detected.
Patients with a frozen shoulder demonstrate, by definition, a restricted range of passive motion with normal glenohumeral radiographs. Patients with partial-thickness cuff defects might similarly demonstrate motion restriction, whereas patients with major full-thickness defects usually have a good range of passive shoulder motion but may be limited in strength or range of active motion. An arthrogram in the case of frozen shoulder shows a diminished volume and obliteration of the normal recesses of the joint.
A snapping scapula can produce shoulder pain on elevation and a catching sensation somewhat reminiscent of the subacromial snap of a cuff tear. However, the latter can usually be elicited with the scapula stabilized while the arm is rotated in the flexed and somewhat abducted position. Scapular snapping generally arises from the superomedial corner of the scapula and produces local discomfort; scapular movement without glenohumeral motion elicits it.
Glenohumeral arthritis can also produce shoulder pain, weakness, and catching. This diagnosis can be reliably differentiated from rotator cuff disease by a careful history, physical examination, and roentgenographic analysis.
Acromioclavicular arthritis can imitate cuff disease. Characteristically, however, the shoulder is most painful with cross-body movements and with activities requiring strong contraction of the pectoralis major. Tenderness is commonly limited to the acromioclavicular joint. Relief of pain on selective lidocaine injection and coned-down radiographs can help establish the diagnosis of acromioclavicular arthritis.
Suprascapular neuropathy and cervical radiculopathy are common imitators of cuff disease. The suprascapular nerve and the fifth and sixth cervical nerve roots supply two of the most important cuff muscles: the supraspinatus and infraspinatus. Thus patients with involvement of these structures can have lateral shoulder pain, and can lack strength of elevation and external rotation.
In the presence of weakness, the neurologic examination should test the cutaneous distribution of the nerve roots from C5 to T1. The biceps reflex and the triceps reflex help screen C5-C6 and C7-C8, respectively. The next component of the neurologic examination requires verifying the integrity of the segmental innervation of joint motion: Abduction, C5; adduction, C6, C7, and C8; external rotation, C5; internal rotation, C6, C7, and C8; elbow flexion, C5 and C6; elbow extension, C7 and C8; wrist extension and flexion, C6 and C7; finger flexion and extension, C7 and C8; and finger adduction and abduction, T1.
A set of screening tests checks the motor and sensory components of the major peripheral nerves; the axillary nerve: the anterior, middle, and posterior parts of the deltoid and the skin just above the deltoid insertion; the radial nerve: the extensor pollicis longus and the skin over the first dorsal web space; the median nerve: the opponens pollicis and the skin over the pulp of the index finger; the ulnar nerve: the first dorsal interosseous and the skin over the pulp of the little finger; and the musculocutaneous nerve: the biceps muscle and the skin over the lateral aspect of the forearm. The long thoracic nerve is checked by having the patient elevate the arm 60 degrees in the anterior sagittal plane while the examiner pushes down on the arm and looks for winging of the scapula posteriorly. Observing the strength of the shoulder shrug checks the nerve of the trapezius. Lesions of the suprascapular nerve produce weakness of elevation and external rotation without sensory loss.
Clinical conditions affecting these structures include cervical spondylosis involving C5 and C6, brachial plexopathy involving the suprascapular nerve, traction injuries (as in the mechanism of Erb’s palsy), suprascapular nerve entrapment at the suprascapular notch, pressure on the inferior branch of the suprascapular nerve from a ganglion cyst at the spinoglenoid notch, traumatic severance in fractures, or iatrogenic injury. *
* References .
Cervical spondylosis involving the fifth and sixth cervical nerve roots can imitate or mask rotator cuff involvement by producing pain in the lateral aspect of the shoulder as well as weakness of shoulder flexion, abduction, and external rotation. Cervical radiculopathy is suggested if the patient has pain on neck extension or on turning the chin to the affected side. Pain of cervical origin more commonly includes the area of the trapezius muscle along with the area of the deltoid, and can radiate down the arm to the hand. Sensory, motor, or reflex abnormalities in the distribution of the fifth or sixth cervical nerve root provide additional support for the diagnosis of cervical radiculopathy. Inasmuch as many asymptomatic patients have degenerative changes at the C5-C6 area, cervical spine radiographs are not a specific diagnostic tool. When mild cervical spondylosis is suspected, it is practical to implement a rehabilitation program without an extensive diagnostic workup. This program includes gentle neck mobility exercises, isometric neck-strengthening exercises, home traction, and protection of the neck from positions that aggravate the condition during sleep. If the condition is unresponsive or severe, additional evaluation by electromyography or MRI, or both, may be indicated.Suprascapular neuropathy is characterized by dull pain over the shoulder that is exacerbated by movement of the shoulder, weakness in overhead activities, wasting of the supraspinatus and infraspinatus muscles, weakness of external rotation, and normal radiographic evaluation. This condition can arise from suprascapular nerve traction injuries, suprascapular nerve entrapment, brachial neuritis affecting the suprascapular nerve, or a ganglion cyst in the spinoglenoid notch. The first three should involve the nerve supply to both the supraspinatus and infraspinatus, and are most easily differentiated by the history. Traction injuries to the suprascapular nerve are usually associated with a history of a violent downward pull on the shoulder, and may be a part of a larger Erb’s palsy–type injury to the brachial plexus. Suprascapular nerve entrapment can produce chronic recurrent pain and weakness aggravated by shoulder use. Brachial neuritis often produces a rather intense pain lasting for several weeks, with the onset of weakness being noted as the pain subsides.
A ganglion cyst in the spinoglenoid notch usually arises from a defect in the posterior shoulder joint capsule, and can press on the nerve to the infraspinatus as it passes through the notch. These cysts are well seen on MRI ( Fig. 14-56 ). Depending on the site of the suprascapular nerve lesion, electromyography can indicate involvement of the infraspinatus alone or involvement of this muscle along with the supraspinatus.

None of these conditions should produce cuff defects on shoulder MRI, ultrasonography, or arthrography. However, as shown by Albritton et al., the degree of rotator cuff muscle atrophy commonly observed after a massive tear may be explained by increased tension on the suprascapular nerve due to muscle retraction.
Imaging of the Rotator Cuff
Plain radiographs of the shoulder can be obtained as a standard evaluation of any patient with suspected rotator cuff abnormality. These include an anteroposterior (AP) view, true AP in the scapular plane (Grashey view) in 30 degrees of abduction, a scapular Y view, and an axillary view. Superior displacement of the humeral head in relation to the acromion and glenoid suggests that the rotator cuff is compromised. A Grashey view in 30 degrees of abduction allows for interpretation of plain films with an activated deltoid muscle. The superiorly directed force of the activated deltoid can make proximal humeral migration more evident in the setting of a large, chronic tear ( Fig. 14-57A and 14-57B ). The scapular Y view can characterize acromial morphology. An axillary view should be obtained with the arm in the scapular plane in neutral rotation. Adequate visualization of “the eye” or the spinoglenoid notch is important in ensuring correct rotation. This axillary view is important in assessing joint space narrowing associated with arthritis and anterior or posterior joint subluxation.

MRI
MRI is now the most often used imaging modality for comprehensive assessment of the shoulder. It can be used to assess the rotator cuff, labrum, capsule, and cartilage. A standard protocol for the rotator cuff should include T1-weighted, fat-saturated proton-density (PD)-weighted, and fat-saturated T2-weighted imaging in the oblique coronal, oblique sagittal, and axial planes. PD- and T2-weighted imaging are useful in assessing the integrity of the rotator cuff tendons. Fat saturation is useful to highlight fluid edema only. T1-weighted images highlight fat, and are useful for assessing fatty infiltration associated with chronic rotator cuff tears.
The rotator cuff tendons should demonstrate low signal intensity on all pulse sequences. Cuff tendinopathy shows intermediate signal intensity on T2-weighted images, and partial- and full-thickness tears demonstrates high signal intensity. It should be noted that in many asymptomatic patients over the age of 60 years, intermediate signal will be seen in the tendon and does not necessarily imply a pathologic state. Rotator cuff tears typically initiate in the superior cuff and can be initially assessed on PD-weighted and T2-weighted coronal imaging ( Fig. 14-58, A ). Additional evaluation of sagittal and axial imaging can allow for three-dimensional characterization of the tear. If a tear is identified, fatty infiltration and atrophy of the muscle belly should be evaluated on the T1-weighted sagittal oblique images as a method to grade chronicity and the likely reparability of the tear (see Fig. 14-58, B ).

The accuracy of MRI as a diagnostic tool for full-thickness rotator cuff tears is excellent, and it has high sensitivity and specificity. The utility of MRI for partial-thickness tears is more limited, but because of the limitations of physical examination for diagnosing partial-thickness tears, it does provide useful information for the clinician. MR arthrography may improve the diagnosis of subtler cuff pathology such as partial-thickness rotator cuff tears, but the necessity of injecting gadolinium through normal cuff structures may occasionally lead to interpretative errors.
Ultrasonography
Ultrasound is an imaging modality that can be utilized to perform dynamic evaluation of the cuff and long head of the biceps tendon. It is less expensive than MRI but is more highly dependent on the operator and the interpretation of the ultrasound images. High frequency (10 to 18 MHz) transducers are used for the shoulder. Because ultrasound is more subject to interpretation errors, the physician evaluating the images should be aware of artifacts that can cause false-negative or false-positive interpretations.
Normal tendon should appear as uniform echogenicity with a fibrillar pattern when the transducer is perpendicular to the tendon axis. Because normal tendon is anisotropic, when the beam is not perpendicular to the tendon, a hypoechoic signal will appear. This should be differentiated from tendinosis, which also produces a heterogeneous echotexture. The tendon should be interpreted as abnormal only if the area maintains a hypoechoic signal as the transducer is moved perpendicular to the tendon axis. A focal area of hypoechogenicity can diagnose partial- and full-thickness tearing with discontinuity of the normal fibrillation pattern of tendons ( Fig. 14-59 ). Compression of the transducer into the area in question can increase the gap size between the tendon fibers and bone and assist in evaluation.

Ultrasonography has also been reported to have similar accuracy to MRI in the detection of full-thickness rotator cuff tears. As mentioned previously, this modality has more significant variability in interpretation, and experience may play a larger role in decreasing the number of false-positive and false-negative interpretations.
Natural History of Rotator Cuff Pathology and Implications on Surgical Indications
Considerable controversy exists on the optimal management of rotator cuff pathology. Orthopedic surgeons have significant variation in their decision-making process for the management of rotator cuff tears. This is evidenced by the lack of high-quality evidence available to surgeons to help guide management. The majority of the clinical practice guidelines set out by the American Academy of Orthopaedic Surgeons are limited or inconclusive. Similarly, the Cochrane reviews fail to provide an evidence basis for the treatment of cuff disease.
There is a need to understand the epidemiology and natural history of rotator cuff disease. Numerous studies have demonstrated a high prevalence of rotator cuff pathology in the aging population. While the exact prevalence varies among studies, a consistent finding is that increased age is associated with a higher prevalence of rotator cuff pathology. In a study using bilateral shoulder ultrasound in patients with unilateral shoulder pain, the average age of patients with bilaterally intact cuffs, unilateral cuff tears, and bilateral cuff tears formed an almost perfect 10-year distribution of 48.7, 58.7, and 67.8 years, respectively. Population-based studies have shown that one-quarter of patients above 60 years of age and half of patients above 80 years will have a rotator cuff tear. These and many other studies have suggested that tendon degeneration and tearing occur with aging.
A strong genetic influence may also contribute to the development of cuff pathology. An investigation utilizing the Utah Population Database has demonstrated a significant heritable predisposition to rotator cuff disease in first- and second-degree relatives of patients with cuff pathology. Other studies have demonstrated increased risk in siblings as well as a genetic predisposition to tear progression.
Natural History of Asymptomatic Rotator Cuff Tears
Studying the natural history of rotator cuff disease is difficult, as patients may or may not have related symptoms. Those with symptoms may request treatment that interrupts disease progression. Ideally, asymptomatic patients that do not require physiotherapy, injections, or surgery can be followed to better understand the natural history of rotator cuff disease. As mentioned previously, the prevalence of bilateral rotator cuff pathology is substantial, and often, patients presenting with pain in one shoulder will have a rotator cuff tear on the contralateral shoulder that is asymptomatic. By performing bilateral ultrasounds, a large number of asymptomatic rotator cuff tears “at risk” for pain development and tear progression can be identified. This model has been used to prospectively follow a large cohort of asymptomatic tears, and has given us a wealth of information regarding tear initiation, location, enlargement, and symptomatic development.
Essential to characterizing rotator cuff pathology is to understand the location and initiation of degenerative rotator cuff tears. The common understanding of rotator cuff tears was that they initiated at the anterior edge of the supraspinatus adjacent to the biceps tendon and propagated posteriorly ( Fig. 14-60 ). This conventional theory has been challenged by ultrasound data from Kim et al. measuring the distance from the posterior edge of the biceps to the anterior edge of a rotator cuff tear as well as the sagittal plane width of a rotator cuff tear. By analyzing the frequency of tear involvement at each millimeter posterior to the biceps tendon, the area around 15 mm posterior to the biceps tendon was found to be most frequently involved, suggesting that degenerative rotator cuff tears initiate at this location ( Fig. 14-61 ).


Anatomically, this location of initiation is interesting from two aspects. First, depending on the anatomic definition of the insertion of the supraspinatus and infraspinatus that is used, 15 mm posterior to the biceps tendon may correlate to the posterior supraspinatus and even anterior infraspinatus. Clark and Harryman demonstrated that significant interdigitation of the supraspinatus and infraspinatus fibers exists in this region of the rotator cuff. Mochizuki et al. have shown that the area of the anterior cuff footprint that involves only the supraspinatus tendon is relatively small, and that the infraspinatus tendon footprint is larger than previously thought. The average AP width of the medial and lateral margins of the supraspinatus were 12.6 ± 2.0 mm and 1.3 ± 1.4 mm, respectively. Using measurements from this study, 15 mm posterior to the biceps tendon involves the anterior infraspinatus. This could potentially explain why isolated infraspinatus muscle atrophy can sometimes be encountered in tears that appear to involve the supraspinatus.
Second, this area of the rotator cuff is within the “rotator crescent” tissue described by Burkhart et al. ( Fig. 14-62 ). He described the “rotator cable” as a thicker band of rotator cuff tissue spanning from the anterior supraspinatus to the posterior supraspinatus and the “rotator crescent” as a thinner tissue lateral to the cable. This crescent tissue is not only thinner but more avascular. The “suspension bridge” configuration of this cable theoretically shields the crescent tissue from stress. The ultrasound data suggest that these rotator cuff tears initiate towards the middle of the crescent tissue and propagate in both directions from the initiation site.

Propagation or involvement of the anterior cable of the rotator cuff have been shown to have significant impact on the rate of irreversible muscle degeneration associated with rotator cuff tears. Based on the suspension bridge concept of the rotator cable, the anterior supraspinatus tissue is important for distributing the forces along the cable. Loss of integrity of this tissue may lead to accelerated retraction and degeneration of muscle tissue that is associated with lower rates of tendon healing. In ultrasound studies, tears involving the anterior cable tissue were associated with significantly greater rates of fatty infiltration of both the supraspinatus and infraspinatus. These data suggest that tears involving the anterior portion of the supraspinatus may need earlier surgical intervention, and that tears not involving but close to the cable may need to be more closely monitored if being treated nonoperatively.
Normal glenohumeral kinematics may be disrupted by larger rotator cuff tears. The infraspinatus may help maintain coronal plane kinematics, and increasing rotator cuff tear size may lead to the development of proximal humeral migration. Using a combination of ultrasound data for tear size and plain radiographs to measure relationships of the humeral head center to the glenoid, Keener et al. found that larger, symptomatic tears involving the infraspinatus had increased superior migration compared with asymptomatic, smaller tears. A critical tear area of 175 mm 2 , which correlates to tear dimensions of approximately 15 mm ×12 mm, was found to be associated with proximal humeral migration.
Longitudinal studies of tear degeneration and pain development in asymptomatic tears are helpful in defining the risks of nonoperative management of degenerative tears. Moosmayer et al. and Mall et al. have reported on longitudinal studies of tear degeneration in asymptomatic shoulders. Moosmayer et al. found that 18 of 50 tears (36%) developed symptoms and that an increase in tear size was associated with symptoms. These results are similar to the findings of Mall et al., who also found that tears that became symptomatic over time also significantly increased in size, while tears that did not become symptomatic did not increase in size. A subsequent study by Keener et al. demonstrated that approximately 50% of asymptomatic tears have a significant increase in size at a median time of 2.8 years. Full-thickness tears are more likely to enlarge, become painful, and progress to muscle degeneration compared with partial-thickness tears.
Natural History of Symptomatic Rotator Cuff Tears
Only a few studies have investigated the natural history of symptomatic rotator cuff tears. These patients are more difficult to study given the interruption of the natural history with any proposed treatment. Fucentese et al. reported on 24 patients who refused operative treatment for full-thickness supraspinatus tears, and found that there was no increase in the average size of the rotator cuff tears on MRI at 3.5 years after the initial MR arthrogram. Of the 24 patients, 8 (33%) had an increase in tear size, and 4 (17%) had no change in tear size. This group of patients treated nonoperatively had a high level of satisfaction.
Two other studies have provided us with additional information regarding the natural history of symptomatic rotator cuff tears. Maman et al. followed 59 full and partial-thickness tears with MRI for a minimum of 6 months. In those tears followed for at least 18 months, 48% were found to have progression of tear size, compared with only 19% in those tears followed for less than 18 months. Similar to findings in asymptomatic tears, full-thickness tears were more likely to progress than partial-thickness tears. Also, age was an important factor, with progression in 54% of tears in patients over the age of 60 years versus 17% in patients under 60 years. Safran et al. studied a cohort of patients under the age of 60 years that were treated nonoperatively for full-thickness rotator cuff tears, and found that 49% of the tears increased in size.
Valuable information has been derived from a longitudinal cohort of more than 400 patients with atraumatic, full-thickness rotator cuff tears followed by the Multicenter Orthopaedic Outcomes Network Shoulder Group. Data from this multicenter group have suggested that pain and duration of symptoms are not associated with the severity of rotator cuff tears. These data are in contrast to those from other studies that have shown stronger correlations between tear size and pain development. The differences are likely due to variations in study design. The Multicenter Orthopaedic Outcomes Network group has found that nonoperative treatment of these full-thickness tears is effective in 75% of patients after 2 years. A patient’s perception of whether physical therapy would be beneficial was a strong predictor of whether conservative treatment would be successful.
Indications for Rotator Cuff Repair
As noted previously, neither the clinical practice guidelines set out by the American Academy of Orthopaedic Surgeons nor the Cochrane systematic reviews provide guidance on the management of rotator cuff tears. Clinical decision-making for the management of rotator cuff tears can be complex and lacks consensus among orthopedic surgeons. As we continue to improve our understanding of the natural history of rotator cuff disease, this complex decision-making process will continue to be refined over time. Based on our current understanding of the natural history of rotator cuff disease and the results of operative repair in a multitude of age groups and tear characteristics, we can generate general guidelines regarding operative indications and the importance of surgical timing.
Patients can generally be divided into three main categories that are based on the potential risk of nonoperative treatments and the proposed benefits of surgical intervention. These categories include those patients needing urgent or early operative repair, those patients that can benefit from a trial of conservative treatment, and those patients that may be best suited for nonoperative treatment.
Group I: Early Operative Repair—Acute Tear
Perhaps the clearest indication for repair of the rotator cuff is the young patient that presents with shoulder pain and weakness after a distinct, acute injury and imaging consistent with an acute full-thickness rotator cuff tear. A thorough history should be performed and should evaluate for preexisting shoulder pain or dysfunction that could be a sign of an acute-on-chronic tear. Additionally, review of imaging should confirm the absence of fatty infiltration on ultrasound or T1 oblique sagittal MRI. Imaging with severe muscle atrophy would suggest more chronic rotator cuff disease that would not be as amenable to surgical repair. Although the supporting literature is limited to case series, urgent repair in the setting of an acute rotator cuff tear typically has positive results.
Operative repair should also be considered in acute subscapularis tears as well as more chronic subscapularis tears with biceps tendon instability. More urgent surgical repair should be considered for acute, retracted subscapularis tears due to the potential for muscle degeneration and fixed degeneration that can occur as a result of these injuries. Repair of more chronic subscapularis tears can be complicated by scar formation and the risk of neurovascular injury due to the proximity to the brachial plexus and associated vasculature. Concomitant biceps tenotomy or tenodesis at the time of subscapularis repair is associated with improved subjective and objective results.
Early surgical repair should be considered in select, higher functioning patients under the age of 60 to 65 years with a full-thickness tear. The impetus for repair comes from the established risk for tear enlargement, muscle degeneration, and change in glenohumeral kinematics over time. Around half of degenerative full-thickness tears will continue to enlarge more than 5 mm at a median of 2.8 years. Additionally, multiple studies have shown that the rates of rotator cuff healing decrease with aging. Therefore early repair may give the patient the best chance at healing. These data should be considered along with short-term follow-up data regarding symptomatic full-thickness tears. However, it is important to note that there have not been any studies that have demonstrated that operative repair for degenerative tears provides any long-term structural or functional advantage over nonoperative management of these degenerative tears. Thus while we can identify shoulders at risk for tear propagation, we cannot be confident that performing surgery on these shoulders will prevent progression of the cuff defect.
Therefore an individualized approach to patients with small full-thickness tears is required, and a thorough discussion with the patient regarding the risks of nonoperative and operative treatment is necessary for this subset.
Group II: Trial of Nonoperative Treatment—Nonacute Tear
A trial of nonoperative treatment aimed at retaining comfort, motion, and strength should be considered in any patient with chronic rotator cuff tendinitis, partial-thickness tear, or full-thickness tear. While such shoulders carry a risk for tear enlargement for full-thickness tears (61%), partial-thickness tears (44%), and intact cuffs (14%), there is time and opportunity to explore the effectiveness of a gentle progressive rehabilitation program.
Articular- and bursal-sided partial-thickness tears can be more painful than full-thickness tears, because in contrast to full-thickness tears, partial-thickness defects of the cuff give rise to stiffness and nonphysiologic tension on the remaining fibers. Because they are unlikely to progress acutely, these tears should be initially treated with nonoperative management. Articular- and bursal-sided partial-thickness should be differentiated, however, because the rates of improvement with nonoperative options in these two groups are different. Articular-sided tears often respond well to physical therapy. On the other hand, bursal-sided tears have a higher failure rate with nonoperative treatment. These lesions can be associated with subacromial abrasions , producing subacromial crepitus on passive joint motion. Because of the limited success with nonrepair strategies for bursal-sided tears, prolonged conservative treatment may be more appropriate for articular-sided tears than for bursal-sided tears.
There is an abundance of literature regarding operative treatment for full-thickness rotator cuff tears, but literature regarding nonoperative treatment of full-thickness tears was lacking until recently; it is now recognized that a nonoperative approach to full-thickness rotator cuff tears can lead to clinical improvement. This subset of patients requires an individualized approach in which the surgeon discusses the risks of tear progression and irreversible muscle changes with nonoperative treatment on one hand versus the risks and chances of a better outcome with surgery on the other.
Group III: Maximize Conservative Care—Irreparable Tear
It is of interest that elderly patients with massive defects in the rotator cuff can realize a comfortable and functional range of motion without surgery. Patients over the age of 60 years with large degenerative tears are unlikely to heal from an attempted cuff repair. In an elderly patient (60 years and older) with a complete or partial rotator cuff defect, administration of a physician-directed, home-based rehabilitation program has proved to be an effective, reproducible, and reliable method of attaining significant clinical and functional improvement.
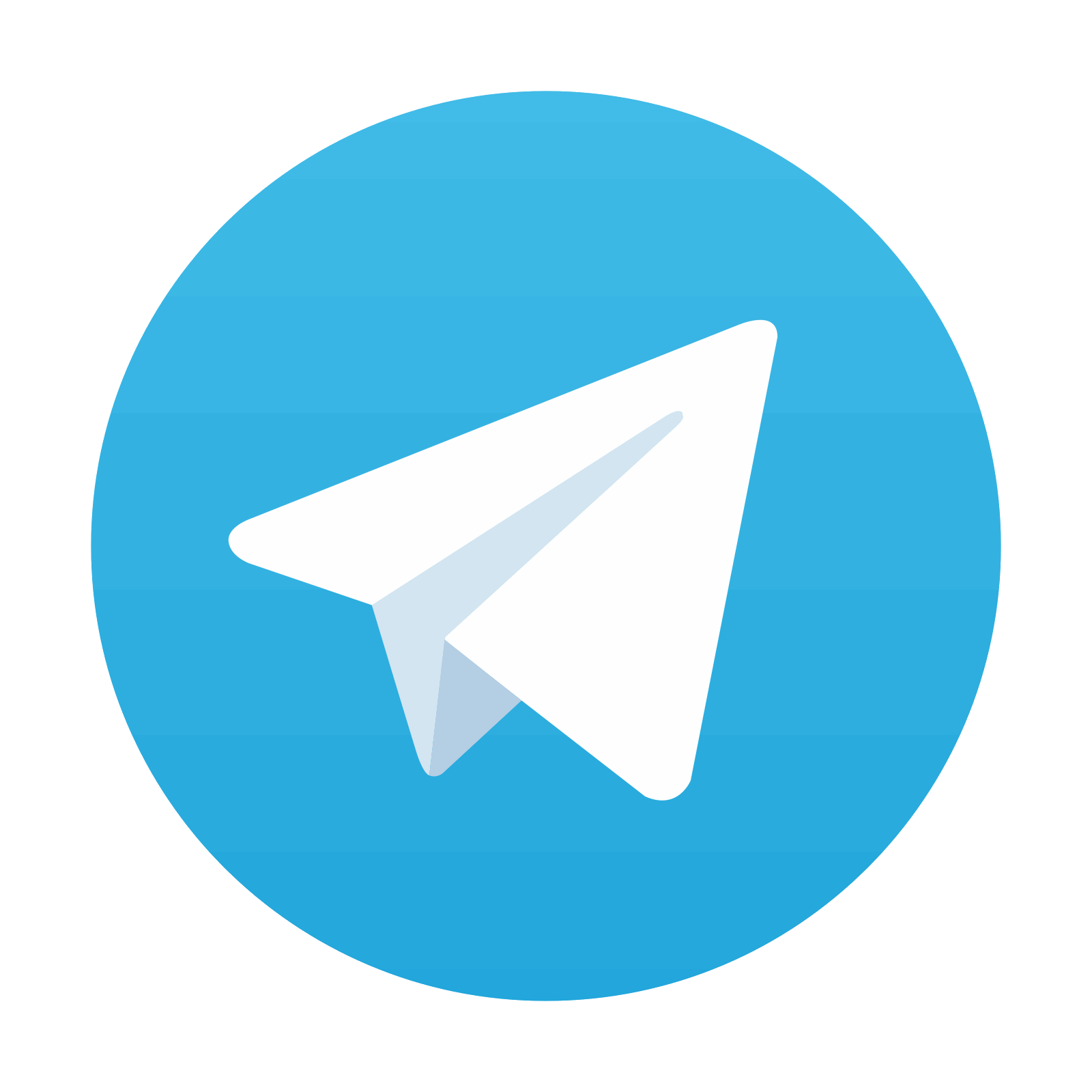
Stay updated, free articles. Join our Telegram channel

Full access? Get Clinical Tree
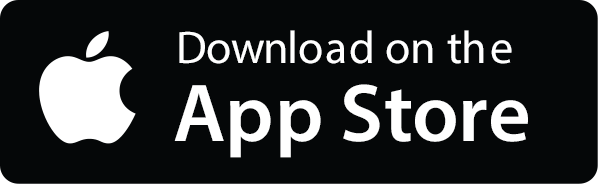
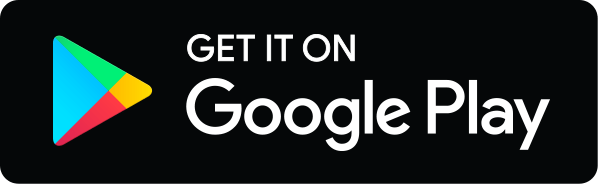