Fig. 29.1
SEIPS Model of Systems in Healthcare: safety and harm are emergent properties of systems (Redrawn with permission from Carayon Qual Saf Health Care 2006)
There are two important implications of this model. First, safe or unsafe outcomes are an emergent property resulting from the interaction of these five elements. However, none of the elements alone can guarantee good outcomes. Second, changes to any of the elements affect all the other elements and the ultimate outcomes. For instance, a team may be adept at emergently cannulating a patient with low cardiac output with good outcomes. However, simply changing the environment from the ICU to the parking structure will have dramatic influence on the outcomes, regardless of keeping the other elements the same. Similarly, a team that is skilled at performing emergent cardiac bypass on adults is not necessarily qualified to perform a palliative procedure on a child with hypoplastic left heart syndrome. Thus, whether any tool used to enhance PCCC outcomes is beneficial or not is contingent on the how well the tool fits in the larger system. As with another common tool, your mileage may vary. With this in mind, the following sections illustrate several categories of tools and technologies: monitoring, mechanical assist devices and medication and care delivery.
Monitoring Technologies and Tools
Arguably, the perioperative and postoperative care of children with cardiac disease has been enhanced through the development of both invasive and non-invasive monitoring tools. However, like any tool, monitoring can be used correctly or incorrectly. And, applying the Systems Engineering Initiative for Patient Safety (SEIPS) model, a monitoring tool without the skilled people to interpret the data and an environment conducive to using the tool is essentially useless or may even create a false sense of security thus increasing risk to patients. Further, as the “people” component of the SEIPS model includes patients, safe and effective use of monitoring technologies requires that appropriate alarm ranges and “normal” values be used. This requirement creates a major challenge for the pediatric cardiac population. The range of normal heart rates differs widely based on age range and the presence or absence of dysthymias. Similarly, normal values of oxygenation depend significantly on whether a patient has pathophysiology that leads to mixing of deoxygenated blood or not. Thus “one size fits all” is a potentially dangerous approach to the use of monitors that can produce false alarms (and thus desensitization to alerts) as well as failure to detect true hazards.
The clinical electrocardiogram (ECG) is considered one of the most valuable and widely used diagnostic tools in modern medicine and is considered the standard of care in the postoperative pediatric cardiac critical care population [2, 3]. Guidelines have been published for ECG monitoring in both adults and children by the American Heart Association, which states that all children after cardiac surgery have an indication for ECG monitoring, given they can be prone to arrthythmias, ischemia, conduction defects, and low cardiac output [3, 4]. Common pediatric postoperative arrhythmias following surgical repair include atrial flutter, junctional ectopic tachycardia, and ventricular tachycardia, all of which can be diagnosed with ECG monitoring and if diagnosed and treated early, can minimize adverse outcomes [5]. There have been numerous adult clinical trials demonstrating that an aggressive approach to treatment of myocardial ischemia, evidenced by ST elevation, improves patient outcomes by decreasing incidence of infarction and cardiac arrest [5]. This finding has not been clearly demonstrated in the pediatric studies, but similar assumptions can be extrapolated. Despite several advantages and benefits of ECG monitoring, it isn’t without its downsides. Human oversight is essential for interpretation of the data to filter out erroneous alarms, which can be frequent and distracting. Cardiac monitor algorithms are purposely designed to have a high sensitivity for the value being measured, but at the expense of specificity, thus leading to numerous false alarms [5]. It is essential that well-trained individuals interpret the data prior to initiating any interventions to avoid overtreatment, particularly when that treatment is invasive with associated risks. Waveform artifact can contribute to false alarms, which can arise from muscle activity such as shivering, seizures, or fasiculations, thus leading to inaccurate heart rate displays [3]. Though there are no pediatric studies evaluating the frequency of false readings, it could lead to inaccurate interpretations of patient condition and possibly unnecessary or missed interventions. A potential risk associated with ECG monitoring is sustaining burns at the electrode sites, which have been reported in adult studies, one such situation resulted in second to third degree burns for a patient who underwent magnetic resonance imaging (MRI) with supposedly “MRI-safe” ECG leads in place [6]. There is also potential for skin breakdown at the lead placement sites, thus necessitating routine changes by the nursing staff, and could lead to venues for infection if breakdown occurs [2]. Finally, there are reports of electrocution when the leads are inadvertently connected to line power (120 volts alternating current) [7]. While none of the real or potential harm from ECG monitoring would justify abandoning wide scale monitoring of cardiac (and even non cardiac) patients, this technology nicely illustrates the importance of understanding both benefits and risks of tools in healthcare.
Invasive hemodynamic monitoring encompasses intraarterial lines, central venous lines, pulmonary artery catheters and intra-cardiac lines. When used to measure pressures as an indication of volume status or cardiac function, these intravascular catheters are attached to a transducer, which converts the pressure waves obtained by the catheter into electrical signals to be analyzed and converted to value, e.g., central venous pressure (CVP), or left atrial pressure (LAP). The potential value of these tools comes from providing real time data on filling pressures which may assist in monitoring and decision making of a patient’s status. Theoretically, a range of clinical conditions that manifest through changes in intravascular pressures could be detected early allowing timely intervention.
However, like ECG monitoring, invasive hemodynamic monitoring requires providers who can interpret the data provided by the monitor and place this information in the larger context of the patient condition. A high CVP associated with high PCO2 in an agitated and hypoxic patient with known pulmonary hypertension requires different interventions than a patient with high CVP and signs of low cardiac output due to a disconnect in a epinephrine infusion. When multiple pieces of information are available, providers may be faced with selecting which data to include or discard in their decision-making. This, coupled with theoretic limits of information processing [8, 9] may result in incorrect interpretations of large numbers of variables.
Beyond the issues of misinterpretation of monitoring data, invasive lines, whether venous or arterial, have been associated with a range of other complications including ischemia distal to insertion site, vascular trauma, arterial and venous thrombosis, thromboembolism, pneumothorax, hemothorax, dysrhythmias, bleeding and local and blood stream infections [10, 11]. Applying a concept of hazard reduction, while multiple steps can be taken to try and minimize these complications, the most effective interventions to improve outcomes include never placing lines unless truly indicated, and then removing the line as soon as no longer needed.
Capnography involves measuring carbon dioxide concentration. End tidal and transcutaneous carbon dioxide (CO2) can be measured episodically or continuously, the latter graphically displayed over time. The use of capnography is well accepted in the context of delivering anesthetics, and can aid in assessing alveolar ventilation, confirmation of endotracheal tube placement and determining the effectiveness of cardiopulmonary resuscitation [4]. Further, the slope of the displayed waveform can provide information about airway resistance. At the same time, concerns have been expressed about the added dead space an inline sensor can add. Despite this, numerous societies have stated support for use of capnography on mechanically ventilated patients [12, 13]. Though there may not be randomized controlled trials proving the value of capnography, these authors agree with publications calling for the routine use of capnography in ICUs barring a contraindication [4, 14].
Pulse oximetry has been used widely in ICUs for decades despite the lack of robust clinical trial. Clinical experience has demonstrated this technology has important component of assuring safe care, leading to expert consensus advocating the routine use of pulse oximetry in ICU settings [4].
A more controversial tool is that of near-infrared spectroscopy (NIRS) monitoring devices for non-invasive continuous regional tissue oxygenation (rSO2) monitoring at the bed side. NIRS devices utilize the principle that oxygenated hemoglobin and deoxygenated hemoglobin molecules have different absorption of light at different wavelengths. The calculated absorption of near-infrared light by hemoglobin molecule in tissues reflects the oxygenation of venous weighed capillary blood in NIRS monitoring. Unlike pulse oximetry, NIRS devices do not analyze for an arterial pulsatile signal, but for the average or nonpulsatile optical component, which is highly related to capillary–venous hemoglobin saturation [15]. This venous-weighted measurement provides an estimation of the regional oxygen supply– demand relationship.
Cerebral NIRS monitoring is most commonly used in intraoperative monitoring of infants and children who undergo congenital cardiac surgery. Interventions based on intraoperative neurophysiologic monitoring including cerebral NIRS appear to decrease the incidence of postoperative neurologic sequelae and reduce the length of stay [16]. In a series of 79 infants who underwent stage 1 palliation, somatic NIRS measurements less than 60 %, and a progressive somatic ischemia indicated by a somatic– cerebral NIRS value difference approaching zero predicted biochemical shock, complications, and longer intensive care unit length of stay [17].
Concerns about this technology include cost, accuracy and thus utility in an ICU setting [18]. Additionally, it is unclear what threshold or level of reading translates into harm to organs [15]. Despite these concerns, a 2011 evaluation made a recommendation for selected use of NIRS based on Class II, level B evidence [15].
The bispectral index (with the dyslexic acronym BIS) monitor is a processed electroencephalographic parameter that integrates time domain, frequency domain, and higher-order spectral analysis of electroencephalogram (EEG) signals (obtained from two electrodes placed over the frontal cortex), which creates a univariate descriptor of the patient’s level of sedation, represented as a numerical value from zero (isoelectric) to 100 (awake with eyes open) [19, 20]. The BIS monitor was initially developed with data from the adult population, but now has widespread use intraoperatively in the pediatric population with a goal to assess conscious level and avoid prolonged periods of cerebral hypoperfusion during the procedure [20]. More recently, the BIS monitor has been utilized in other venues in pediatrics including the emergency room as well as the intensive care unit (ICU) to more objectively titrate sedation to desired effect prior to encountering any adverse sequelae from the medications. A BIS index of less than 40 denotes a deep hypnotic state; 40–60 is general anesthesia, which has been verified in several intraoperative studies; and 70–90 is light to moderate sedation [19, 21]. There is some debate amongst intensivists whether or not this modality of monitoring aids in sedation management decisions for critically ill children given it has some inherent limitations and costs.
The notion that BIS monitoring can improve patient outcomes, decrease medication costs, and reduce the incidence of withdrawal is enticing, though this has not been clearly demonstrated in literature. BIS monitoring provides a way to objectively assess a patient’s sedation level in the face of altered heart rate, blood pressure, and physical examination to avoid possible over- or under-sedation, which may be associated with increased morbidity and costs [22]. There have been a limited amount of studies evaluating BIS monitoring in the pediatric ICU, which had similar results emphasizing that BIS scores can be an adjunct to already established methods of assessing level of sedation, but may be beneficial when physiologic parameters are altered by other medications (sedation, inotropes) or with neuromuscular blockade [20, 22].
There are some limitations to BIS monitoring which need to be considered to justify its use. Erroneous measurements have been widely reported, particularly with facial movements and eternal stimuli, which can be difficult to control at times in a busy and noisy ICU [21]. In a pediatric ICU case series, the sedation agent chosen impacted the utility of the BIS scores showing that patients receiving certain sedating medications showed a less accurate correlation with BIS scores to sedation scores [20]. Another question is whether or not BIS monitoring is sensitive enough to differentiate between the different levels of sedation, which would be it’s main utility in the pediatric intensive care environment. In a pediatric prospective study, BIS scores reliably differentiated between inadequate and adequate sedation, but was relatively insensitive for differentiating between adequate and over-sedation [22]. It should also be noted that BIS monitoring was developed using adult EEG data and was not designed to incorporate the developmental changes of the pediatric EEG. There have been no documented injuries associated with BIS monitoring, but given the electrodes in place for potentially prolonged periods of time, the risk for skin breakdown must always be a concern for the provider. The are a limited amount of cost-benefit analyses of BIS monitoring in cardiac and noncardiac surgical patients, which find the costs of the technology trivial when weighed against the potential benefits of more precise sedation, shorter intubation times, and less hemodynamic sequelae as a results of over-sedation [23].
Studies incorporating the use of BIS monitoring for critically ill children are promising given the possibility of improving patient outcomes, reducing costs, and being able to objectively titrate sedation, though further evaluation is needed to justify its use in the ICU environment.
Mechanical Support Technologies
Mechanical circulatory support, the mainstay being extracorporeal membrane oxygenation (ECMO) and the ventricular assist device (VAD), may be used in children as a bridge to recovery or a bridge to cardiac transplantation [24].
ECMO has been demonstrated to improve outcomes in select pediatric populations (acute respiratory failure, neonatal respiratory failure) though data on ECMO in the post operative cardiac population shows survival ranging from 33 to 64 % [25, 26]. A study of neurologic outcomes in a mixed pediatric population who underwent extracorporeal membrane oxygenation during cardiopulmonary resuscitation (E-CPR) showed favorable outcome in 95 % of patients [27]. Mahle et al. 2005 analysis of cost-utility of salvage ECMO in 32 children (84 % of whom had cardiac disease) demonstrated justifiable cost-utility [28]. Of note, of the technologies discussed so far in this chapter, ECMO is the one with such an analysis.
However, ECMO is not free of risk. Complications include bleeding, thromboembolic events including strokes, infection, and death [29–32]. While some of these complications are beyond the control of providers, when ECMO is considered from the standpoint of systems thinking, many of these risks are potentially manageable. Because of the interdisciplinary nature of ECMO (involving surgeons, intensivists, nurses, perfusionists, anesthesiologists, pharmacists and more), effective team performance is imperative [33]. Not surprisingly, safety tools such as failure modes and effects analysis (FMEA) have been successfully applied to the domain of ECMO with a reduction in risk [34]. Such applications may be appropriate for the other technologies considered.
VADs have been widely used in the adult population for years with very good outcomes, but the use of these devices in children has historically been limited, though the use has expanded in recent years, particularly in the congenital heart disease population. There are two main types of VADs used in children: centrifugal pump (the Bio-Pump) and pulsatile (Heartmate, Thoratec, Berlin Heart, ABIOMED BVS 5000, and MEDOS HIA). Indications for placement of a VAD are similar to that of ECMO including failure to separate from cardiopulmonary bypass (CPB) following surgical repair, or right, left, or biventricular failure, but the patient but still be able to adequately maintain oxygenation through their lungs [35]. There are pediatric studies reporting good outcomes with the use of VADs, particularly as a bridge to transplant. A study of the Pediatric Heart Transplant Study database found a survival to transplantation rate of 77 % using VADs from 1993 to 2003 with even better survival in the later years from 2000 to 2003 of 86 % [24]. But it is important to note that the success rate with VAD usage is significantly lower in patients with congenital heart disease and in the smaller, younger patients, which is where they are often utilized [24]. And there are numerous inherent risks and adverse outcomes associated with VADs in addition to their associated costs that must be considered when determining how beneficial they are to the pediatric cardiac surgical population.
There are limited studies with VAD use in pediatrics, but some have reported excellent outcomes of their use both with postoperative recovery or as a bridge to transplant [36]. There are studies with Thoratec usage in children, some reporting nearly 70 % survival to hospital discharge, though the best outcomes were seen with patients diagnosed with cardiomyopathy or myocarditis with only minimal survival and significant neurologic complications in patients with congential heart disease [37, 38]. The Berlin Heart VAD system is widely used and comes in five sizes as is suitable for patients of all ages ranging from neonates (>2.5 kg) to adolescents [24]. Survival to discharge with the Berlin Heart has been better in the older pediatric patients aged 6–16 years with a 52 % survival rate [35]. There are few long-term follow-up studies of children supported with VADs, but one was done at Children’s Hospital of Boston over a decade, which reported that 37 children supported with mechanical circulatory support (11 of them with VADs), the overall survival rate was 95 % with one reported death [39]. Of these survivors, they reported 80 % of them were in good to excellent health with 90 % having normal ventricular function on echocardiogram at follow up years later [39]. A major advantage of utilizing a VAD versus ECMO for a pediatric patient is the lower rates of neurologic complications associated with VADs, likely due to the decreased anticoagulation need for this modality of support resulting in fewer intracranial hemorrhages. However, often times ECMO is indicated over VADs due to the degree of complexity of illness in the child and if they need respiratory support as well as cardiac support due to pulmonary hypertension or hypoxemia.
< div class='tao-gold-member'>
Only gold members can continue reading. Log In or Register a > to continue
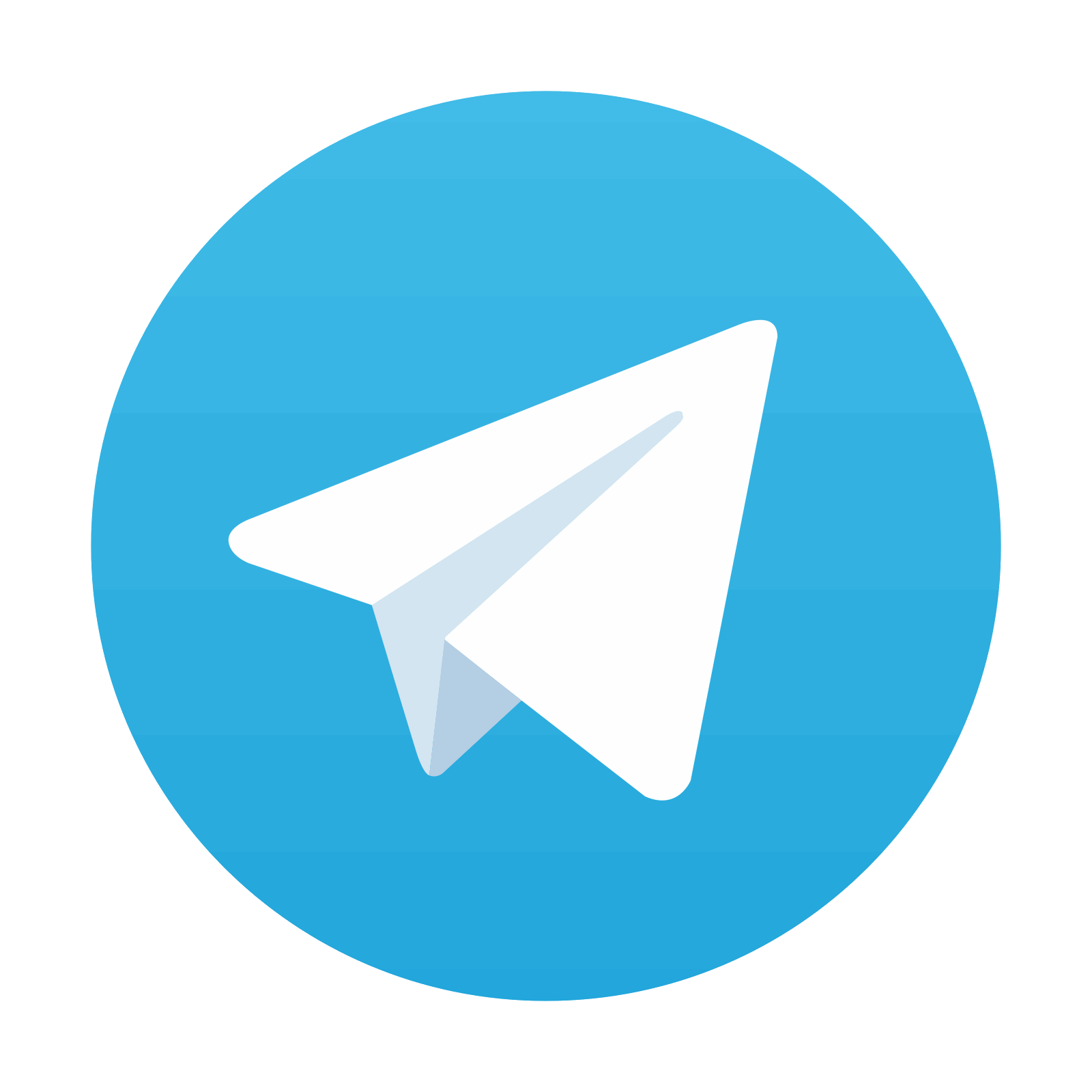
Stay updated, free articles. Join our Telegram channel

Full access? Get Clinical Tree
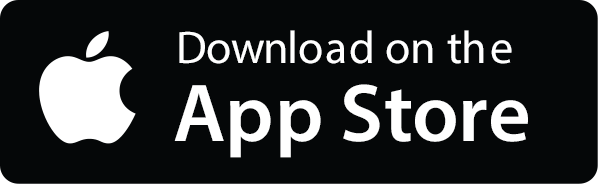
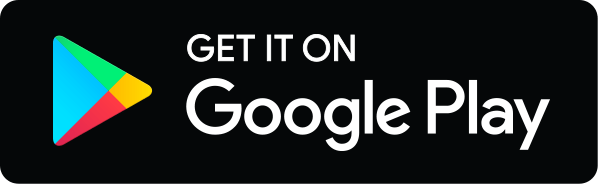