© Springer International Publishing Switzerland 2015
Amy Firth and Jason X.-J. Yuan (eds.)Lung Stem Cells in the Epithelium and VasculatureStem Cell Biology and Regenerative Medicine10.1007/978-3-319-16232-4_1515. The Role of Stem Cells in Vascular Remodeling in CTEPH
(1)
Laboratory of Genetics, The Salk Institute for Biological Studies, La Jolla, CA 92037, USA
(2)
Department of Medicine, Arizona Health Sciences Center, University of Arizona, Tucson, AZ, USA
Keywords
Pulmonary hypertensionNicheDifferentiationMesenchymal stem cellsPericytesEmbolismAbbreviations
BM-MSC
Bone marrow-derived mesenchymal stem cell
CD11b
Cluster of differentiation factor 11b; aka integrin alpha m
CD14
Cluster of differentiation factor 14
CD19
Cluster of differentiation factor 19; aka B-Lymphocyte antigen
CD34
Cluster of differentiation factor 34
CD45
Cluster of differentiation factor 45; aka lymphochyte common antigen
CD73
Cluster of differentiation factor 73; aka 5′-nucleotidase
CD79α
Cluster of differentiation factor 79alpha; aka B-cell antigen receptor complex-associated protein alpha chain and MB-1 membrane glycoprotein
CD90
Cluster of differentiation factor 90; aka Thy-1
CD105
Cluster of differentiation factor 105; aka Endoglin
CFU
Colony-forming unit
CTEPH
Chronic thromboembolic pulmonary hypertension
EnMT
Endothelial to mesenchymal transition
EPC
Endothelial progenitor cell
eNOS
Endothelial nitric oxide synthase
EPC
Endothelial progenitor cells
HLA-DR
MHC class II cell surface receptor encoded by the human leukocyte antigen complex
HMGB1
High-mobility group protein B1
HO
Hemeoxygenase
MAPC
Multipotent adult progenitor cells
MCT
Monocrotaline
miRNA
Micro ribonucleic acid
MSC
Mesenchymal stem cell
MSC-MV
Mesenchymal stem cell-derived micro vesicle
PAE
Pulmonary endarterectomy
PAH
Pulmonary arterial hypertension
PE
Pulmonary embolism
PVR
Pulmonary vascular resistance
RAGE
Receptor for advanced glycation end products
SMC
Smooth muscle cells
TGFβ
Transforming growth factor beta
UTR
Untranslated region
VEGF-R2
Vascular endothelial growth factor receptor 2
vWF
von Willebrand factor
15.1 Introduction
Chronic Thromboembolic Pulmonary Hypertension or CTEPH is caused by the failure of a pulmonary embolism to resolve. CTEPH is a notoriously underdiagnosed and severe form of pulmonary hypertension characterized by stenosis or complete obliteration of the pulmonary artery by an intraluminal thrombus that has become fibrotic. Such persistence of pulmonary thromboemboli occurs in 0.1–9.1 % of all cases (Guerin et al. 2014; Becattini et al. 2006; Poli et al. 2010; Pengo et al. 2004). Obstruction of the pulmonary vascular bed by non-resolving thromboemboli leads to increased pulmonary vascular resistance (PVR). Progressive pulmonary hypertension and right heart failure then ensue. In the absence of intervention, CTEPH prognosis is poor. While the clinical and hemodynamic features of the disease are robustly defined (Banks et al. 2014), there is still a lack of understanding of the associated pathophysiology leading to the progression of the disease. Pulmonary endarterectomy (PAE) surgery remains the frontline treatment for patients diagnosed with CTEPH; however, a number of patients are inoperable or develop persistent pulmonary hypertension subsequent to PAE (D’Armini et al. 2014; Hoeper et al. 2014; Hill et al. 2008; Jamieson et al. 2003). Furthermore, lung reperfusion injury and persistent pulmonary hypertension remain serious complications of PAE (Hsu et al. 2007; Lee et al. 2001).
Recent investigations striving to elucidate the mechanisms involved in the formation and resolution of a venous thrombosis have led to an increased understanding of clot interactions with the surrounding vasculature. Genetic and pathophysiological studies have focused on determining the cellular and molecular pathways including those involving regulation of growth factors, cytokines, metabolic signaling, elastases, proteases, and microRNAs (miRNA) (Zabini et al. 2014; Feng et al. 2014; Wynants et al. 2013; Wang et al. 2013; Ogawa et al. 2009). Furthermore, an increased recognition of stem and progenitor properties in cells resulted in identification of cells, both circulating and within the vascular wall that have a high degree of plasticity.
While the vessel is initially occluded by a pulmonary embolism, in patients who develop CTEPH this becomes a fibrotic coagulant material blocking the central artery. Subsequently, this leads to vascular remodeling of the arterial segments that are both surrounding and distal to the occluded regions and the development of elevated PVR. The process of pulmonary arterial remodeling has been associated with a number of changes in vascular function including; (1) increased proliferation and migration of vascular smooth muscle cells; (2) endothelial dysfunction; adventitial thickening due to excessive adventitial fibroblast and myofibroblast; and (3) a build-up of circulating inflammatory cells. Most recently, both resident vascular progenitor cells and circulating progenitor/precursor cells which become encompassed in the fibrotic clot have been identified and proposed to play a role in the pathogenesis of CTEPH. The abnormal microenvironment/niche that the fibrotic clot provides is thus speculated to drive misguided differentiation and proliferation of these cells contributing to stabilization of the clot and associated pulmonary vascular remodeling (Firth et al. 2010).
This chapter will introduce CTEPH as a form of pulmonary hypertension and provide a summary of the pathogenesis of CTEPH and the postulated contribution of progenitor and stem cells. Further detail on myofibroblasts and endothelial progenitor cells can be found in other chapters in this book.
15.2 Pathogenesis of CTEPH
It is established that CTEPH develops subsequent to a pulmonary embolism arising from a deep vein thrombosis. Studies also suggest that in situ lung thrombosis may contribute to disease progression by promotion of the growth and stabilization of the thromboemboli. Why the PE fails to resolve remains a question to be fully elucidated. As mentioned above, a prothrombotic mutation in fibrinogen has been associated with the incidence of CTEPH (Chen et al. 2010; Morris et al. 2009; Laczika et al. 2002). This was discovered after it was reported that the fibrin in patients with CTEPH was relatively resistant to fibrinolysis in vitro (Li et al. 2013; Morris et al. 2006). Studies have investigated the impact of such mutations and found that they are associated with a fibrinogen that, in vitro, is partially resistant to plasmin-mediated lysis when compared to fibrinogen from healthy controls. If such resistance to fibrinolysis occurs in vivo, one could postulate that the fibrin may persist in the thromboemboli and stimulate the remodeling process. Indeed, we observed a significant effect of chronic exposure of fibrin and fibrinogen on the regulation of intracellular calcium in both primary pulmonary artery smooth muscle and endothelial cells (Firth et al. 2009). Intracellular calcium regulation is central to the control of cell migration, proliferation, and contraction, all components of vascular remodeling process. Further studies are necessary to fully understand the impact of mutant fibrinogen on the pathogenesis of CTEPH. Association of mutations in fibrinogen leading to differences in the structure of fibrin in the clot may account for the development of CTEPH in a subpopulation of CTEPH patients; however, it cannot currently account for all cases. Identification of such a polymorphism may be valuable in acting as a biomarker for identifying CTEPH risk.
It has also been proposed that CTEPH could also arise due to small vessel disease leading to a secondary thrombosis. Clinical evidence does suggest that there is endothelial cell damage in the small pulmonary vessel and intimal hyperplasia likely leading to the development of proximal vascular lesions.
15.3 Identification of Stem and Progenitor Cells Associated with CTEPH
Stem and progenitor are terms that are often used interchangeably. By definition, a progenitor cell is like a stem cell with the capacity for both self-renewal and differentiation, but that has already become more committed differentiating into its target cell. It is difficult to categorize the cell types involved in the pathogenesis of CTEPH until we fully understand their origin and differentiation potential. Here we will describe what is currently known with regard to this. Indeed, recently, specific vasodilator compounds are demonstrated to have some clinical efficacy in inoperable PAE patients, indicating some level of vascular disease (Seyfarth et al. 2010).
15.3.1 Mesenchymal Progenitor Cells
The precise definition of a mesenchymal progenitor or stem cells is still difficult to pinpoint due to a lack of specific cell surface markers. Currently, the minimal criteria as defined by the International Society for Cellular Therapy states that a mesenchymal stem cell (MSC) must be; (1) plastic-adherent; (2) express CD105, CD73, and CD90, and lack expression of CD45, CD34, CD14 or CD11b, CD79α or CD19, and HLA-DR; and (3) must have the capacity to differentiate to osteoblasts, adipocytes, and chondroblasts in vitro (Dominici et al. 2006). As further studies are completed, these criteria will become clearer and I refer you to a paper detailing identification of progenitor cells in the pulmonary vasculature (Firth and Yuan 2012).
Mesenchymal stem cells are currently being pursued as a therapeutic approach for other forms of PH. Administration of BM-MSC has been shown to attenuate the development of monocrotaline-induced PH and endothelial dysfunction in rats (Hansmann et al. 2012; Baber et al. 2007). Nanoscale MSC-derived microvesicles (MSC-MV) (Chen et al. 2014) or exosomes (Lee et al. 2012) have also been demonstrated to have pleiotropic effects in PH by inhibiting the hyperproliferative pathways. Furthermore, MSC over-expressing heme-oxygenase (HO) (Liang et al. 2011) or endothelial nitric oxide synthase (eNOS) (Kanki-Horimoto et al. 2006) are able to attenuate the development of chronic hypoxia-induced PAH and MCT-induced PAH, respectively. The role of MSC pathogenically or therapeutically in CTEPH has not currently been investigated. Other cells with a mesenchymal progenitor phenotype have, however, been identified in CTEPH and thought to contribute to its pathogenesis. These cells are discussed below.
Myofibroblasts
Myofibroblasts are cell type exhibiting significant plasticity with characteristics of both fibroblasts and smooth muscle cells. They are able to produce extracellular matrix and have known roles in wound healing and inflammation. A number of precursors or progenitors for the myofibroblast have been identified. Fibroblasts are the primary source, with smooth muscle cells, pericytes, bone marrow-derived circulating fibrocytes, and epithelial to mesenchymal transition all identified as potential sources.
Cells representing a myofibroblastic phenotype were first identified in endarterectomized tissues from patients with CTEPH in our laboratory (Firth et al. 2010) and are currently thought to be a predominant cell type found in the endarterectomized tissues from CTEPH patients (Sakao et al. 2011; Maruoka et al. 2012). After histological examination, our laboratory identified a population of cells expressing intermediate filaments vimentin and smooth muscle alpha actin in endarterectomized tissues from patients with significant neointimal formation. These cells generated single cell colonies resembling CFU-fibroblast, grew in a network like pattern, and had the capacity for at least adipogenic and osteogenic differentiation (Firth et al. 2010). In a subsequent study similar populations of cells with a “cancer-like” phenotype were identified in the endarterectomized tissue; these cells were hyper-proliferative, anchorage-independent, and invasive (Maruoka et al. 2012). Indeed, this cancer-like hypothesis was supported by a study by Jujo and colleagues (Jujo et al. 2012). After a second passage of isolated myofibroblast-like cells, they noted the presence of a pleomorphic cell type similar to those in pulmonary intimal sarcoma. This tumor is thought to originate from sub-endothelial-mesenchymal cells in the pulmonary vascular wall. These cells behaved like the ones in the aforementioned studies being hyper-proliferative, anchorage-independent, invasive, and serum-independent. The authors defined them as sarcoma-like cells (SCLs) and, when injected into C.B-17/lcr-scid/scidJcl mice, they developed solid, undifferentiated tumors at the site of injection (Jujo et al. 2012). Together, these studies would suggest that there are properties to the stabilized clot that generates a unique microenvironment promoting the differentiation/de-differentiation of cells to enhance intimal remodeling.
There is still a poor understanding of the cellular and molecular mechanisms that lead to the development of CTEPH. In a search for biomarkers, Moser and colleagues identified the expression of Receptor for Advanced Glycation Endproducts (RAGE) and High-Mobility Group Protein B1 (HMGB1) in myofibroblasts isolated from endarterectomized tissues (Moser et al. 2014). Additionally, HMGB1 was significantly elevated in serum of patients with CTEPH (Moser et al. 2014). It will be necessary to develop a more detailed understanding of the RAGE-HMGB1 axis in CTEPH and determine its potential to serve as a biomarker.
15.3.2 Endothelial Progenitor Cells
Endothelial progenitor cells (EPC) is a term that is now known to encompass a number of subpopulations of circulating cells with the capacity to differentiate into endothelial cells (Khakoo and Finkel 2005). Such EPC are considered: (1) derivatives of hemangioblasts expressing surface receptors CD34, VEGFR-2, and CD133; (2) subsets of bone marrow-derived multipotent adult progenitor cells (MAPC) expressing CD133 and VEGFR-2 lacking CD34 or vascular endothelial cadherin (VE-Cad); (3) bone marrow-derived myelo/monocytic cells expressing CD14 and forming vWF, VEGF-R2, and CD45+ endothelial cells. All of these EPC populations have the common ability to retain acetylated LDL. For a more detailed review of this cell type, please refer to Chaps. 9 and 17.
Although putative endothelial progenitor cells have been identified in endarterectomized tissues from patients with CTEPH (Yao et al. 2009), they do not appear to be significantly increased in the circulation (Smadja et al. 2010). This is contrary to what is known for PAH where consistently elevated levels of circulating endothelial progenitor cells have led to them being considered biomarkers (Smadja et al. 2009, 2010). Recruitment of EPC requires a cascade of signaling events which include the adhesion and migration and chemoattraction (Urbich and Dimmeler 2004); a final differentiation to endothelial cells drives neo-vascularization and re-endothelialization. Such events were observed by Yao et al. in endarteractomized tissues indicating, at minimum, a functional recruitment of EPC into the clot region (Yao et al. 2009).
Endothelial to Mesenchymal Transition
Endothelial to mesenchymal transition or EnMT describes a phenotypic switch from an endothelial cell to a cell of mesenchymal origin. Indeed, pulmonary EnMT is thought to contribute to the development of pulmonary fibrosis (Almudever et al. 2013; Diez et al. 2010; Arciniegas et al. 2007). While we still have a relatively poor understanding of the contribution of this process in the development and pathogenesis of pulmonary hypertension, in particular, CTEPH studies do confirm the acquisition of mesenchymal cell markers like alpha-smooth muscle actin, sm22-alpha, and myocardin and EnMT-related transcription factors: slug, snail, zeb1, and endothelin-1 in EPC co-cultured with SMC (Diez et al. 2010) and in PAEC (Almudever et al. 2013). TGFβ and endothelin-1 signaling was key to the EnMT transition (Almudever et al. 2013; Diez et al. 2010). Consequentially, targeting the BH4 synthesis “salvage pathway” has been proposed to be a novel therapeutic strategy to attenuate pulmonary hypertension in idiopathic pulmonary fibrosis (Almudever et al. 2013). It is plausible that such an EnMT could contribute to the pathogenesis of CTEPH; both EPC and cells with a mesenchymal phenotype have been identified in endarterectomized tissues; however, their precise roles in the stabilization of the clot and in pulmonary vascular remodeling are not precisely defined (Firth et al. 2010; Yao et al. 2009; Jujo et al. 2012; Sakao et al. 2011)
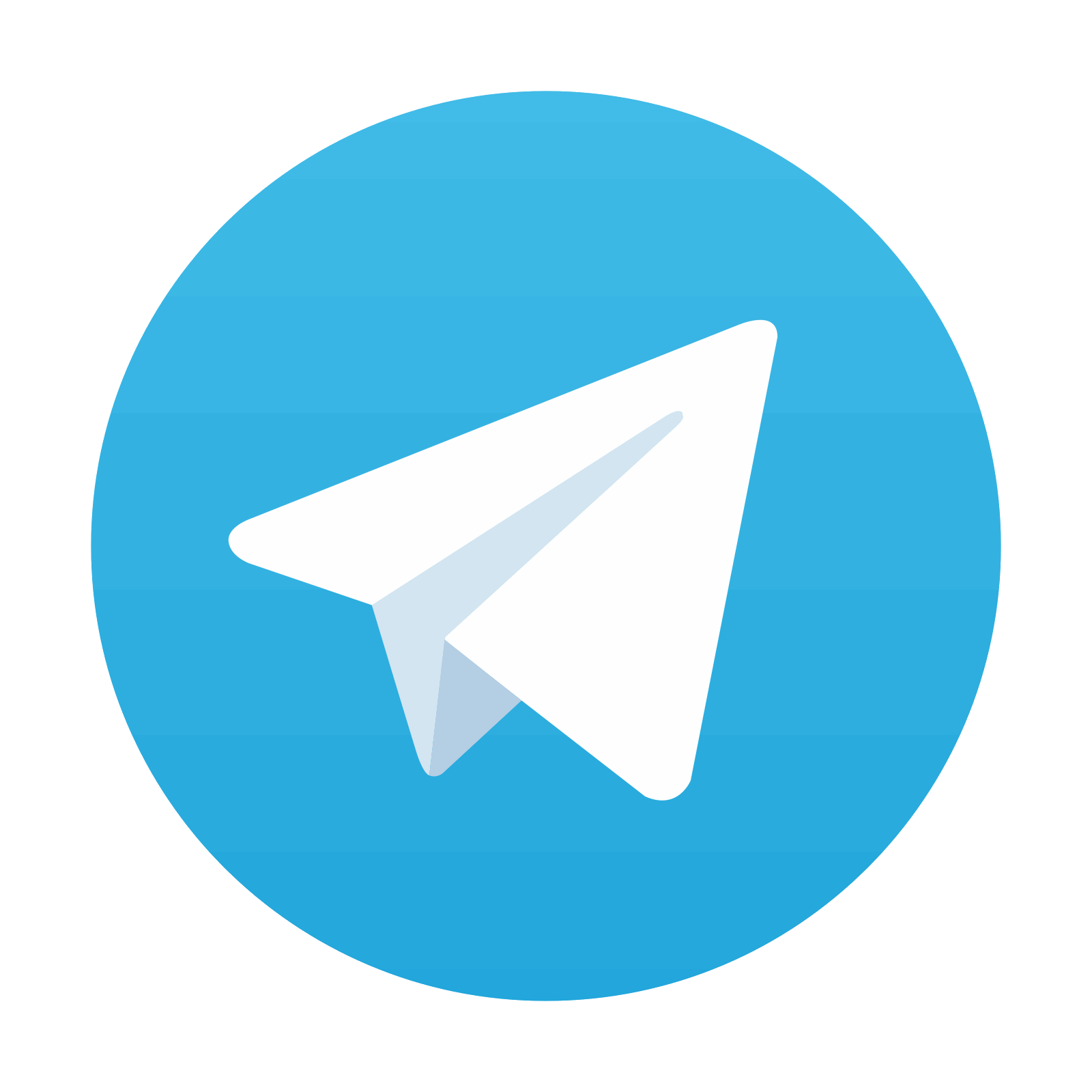
Stay updated, free articles. Join our Telegram channel

Full access? Get Clinical Tree
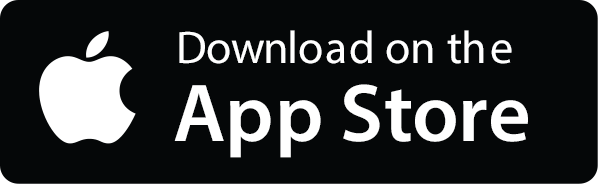
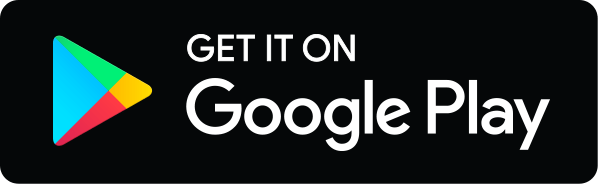
