Fig. 1
Function of NADPH oxidase in oxidative burst of neutrophils
Superoxide is not the only reactive oxygen species formed during oxidative burst, as further cascade of chemical reactions leads to the generation of other oxidative agents. Superoxide may dismutate in the reaction either catalyzed by superoxide dismutase (SOD) or spontaneously into hydrogen peroxide. It is possible to generate hydroxyl radical from hydrogen peroxide in the Fenton reaction, catalyzed by iron, although this mechanism is deficient in neutrophils as they lack ferrous ions (Britigan et al. 1990). After neutrophils’ activation azurophilic granules release myeloperoxidase (MPO) into the phagosome, where MPO catalyzes the synthesis of hypochlorous acid from hydrogen peroxide as a substrate. HOCl then reacts with amino acids, giving chloramines. Furthermore, superoxide may react with hypochlorous acid, which results in the formation of hydroxyl radical (Ramos et al. 1992). In the reaction between hydrogen peroxide and hypochlorous acid singlet oxygen is formed. Another source of ROS is the reaction between hydrogen peroxide and superoxide, but low amounts of synthesized singlet oxygen and hydroxyl radical seem not to be of high importance in biological systems (MacManus-Spencer and McNeill 2005).
Generation of ROS is supported by the production of RNS, which are also involved in the clearance of pathogens. Enhanced synthesis of nitric oxide (NO) is associated with the process of inflammation, when mRNA for inducible form of nitric oxide synthase (iNOS) is being transcribed. The substrates for NO synthesis are L-arginine, NADPH, and oxygen, with the tetrahydrobiopterin as a cofactor. NO diffuses easily across cellular membranes and its antimicrobial properties are significantly enhanced when it reacts with superoxide, forming peroxynitrite, a potent pro-inflammatory and cytotoxic molecule (Cuzzocrea et al. 2001). Other RNS that might be formed during reactions of endogenous NO with oxidants are nitrogen dioxide and dinitrogen trioxide. Both ROS and RNS are strong oxidants and these compounds can react with many biomolecules including DNA, proteins, lipids, and thiols. Reactions of oxidation and nitration/nitrosylation result in the formation of toxic products and, in turn, they facilitate clearance of pathogens (Eiserich et al. 1998).
3 ROS and RNS in Chemotaxis, Neutrophil Adhesion, Rolling, and Phagocytosis
It has been observed that ROS are crucial regulators of neutrophil chemotaxis. In the process of chemotaxis, a neutrophil forms pseudopods. The well-directed pseudopods are maintained, whereas pseudopods facing the wrong direction are destroyed enabling migration toward chemoattractants. It has been proposed that disruption of misoriented pseudopods may be, in large part, mediated by ROS production (Hattori et al. 2010).
As mentioned above, compounds released during oxidative burst are able to move across cell membranes. Therefore, even if produced within cell, they may affect processes occurring extracellularly. Neutrophils migrating to tissues release ROS, which, in turn, activate endothelial cells (Fialkow et al. 2007). It creates a specific loop, as ROS induce the prolonged expression of the glycoprotein ligand for polymorphonuclear adhesion GMP140 on endothelial cell surface. ROS are able to regulate the expression of endothelial cell adhesion molecules (CAM) by a direct activation of CAMs on the surface and also by a transcription-dependent mechanism which involves transcription factors sensitive to oxidation (Patel et al. 1991).
Not only reactive oxygen species but also nitrogen species may regulate endothelium – neutrophil adhesion. Depletion of NO causes increased oxidative stress within endothelium and mast cells. It results in a promotion of neutrophil adhesion to the blood vessel wall (Niu et al. 1996). Other studies revealed that treatment of neutrophils with NOS inhibitors enhanced carrageenan-induced rolling/adhesion of neutrophils to endothelial cells. Additionally, NOS inhibitors block the apoptosis process in migrating neutrophils (Dal Secco et al. 2003). Moreover, NO can modulate migration of neutrophils by inducing neutrophil-derived microparticles (Nolan et al. 2008).
On the surface of neutrophils there are two types of Fc receptors: FcγRIIa and FcγRIIIb. They are responsible for phagocytosis and killing of opsonized pathogens within the cell. Activation of FcγRIIa may be induced by crosslinking of FcγRIIIb, which, in turn, leads to neutrophil degranulation and generation of reactive oxygen intermediates (Salmon et al. 1995). Under physiological conditions, respiratory burst is involved in amplification of Fc receptor-mediated phagocytic function and in patients suffering from chronic granulomatous disease Fc receptor-mediated phagocytosis is abrogated. This pathway requires hydrogen peroxide, lactoferrin, and superoxide anion, but not hydrogen peroxide-MPO-halide system, which underscores the pivotal role of ROS in the amplification of phagocytosis (Gresham et al. 1988). Interestingly, it has been recently shown that inducible nitric oxide synthase interacts with Rac2 and is translocated to phagosomes after phagocytosis. As a consequence, ROS and RNS are produced to kill microbes within this cellular compartment (Jyoti et al. 2014).
4 Role of Oxidative Stress in NETs Formation
Convincing evidence on the role of ROS in NETosis has come from studies on neutrophils isolated from CGD-patients. Lack of NADPH-oxidase in cell membrane impairs the production and release of NETs in response to different stimuli (Fuchs et al. 2007). In line with those findings, murine model of CGD confirmed this observations as neutrophils isolated from gp91 −/− mice do not release extracellular traps (Ermert et al. 2009). In the same study, NET formation interrelated with the amount of ROS produced. It has been shown that the mechanism underlying ROS-induced NETosis is based upon translocation of neutrophil elastase (NE) to the nucleus, where it degrades histones and facilitates chromatin decondensation. Myeloperoxidase acts synergistically with NE in this process. Further support for the role of ROS in NETosis comes from the fact that phorbol myristate acetate (PMA) – a potent stimulant of NETosis becomes inactive when exposed to diphenyleneiodonium chloride (DPI), an NADPH oxidase inhibitor (Papayannopoulos et al. 2010). Some studies were undertaken to establish the clear role of different oxidants in NETosis. Due to difficulties with targeting appropriate cell compartment using oxidant scavengers and enzymes inhibitors, and multiple reactions involved in the generation of ROS, there are no apparent conclusions (Parker and Winterbourn 2012). Yet, it has been shown that mitochondrial ROS are not involved in NETosis (Kirchner et al. 2012).
Notably, in some cases of NETs formation, ROS are not involved. For instance, in cystic fibrosis airway inflammation traps may be released upon activation of G protein-coupled receptor CXCR2 and this process is independent of NADPH oxidase (Marcos et al. 2010). Furthermore, early formation of NETs observed after exposure to S. aureus is also independent of ROS produced by NADPH oxidase (Pilsczek et al. 2010). Accordingly, it is possible that ROS might account for most but not all NETs.
To date, the role of NO and nitrosative stress in NETs formation has not been largely studied. However, it has been recently discovered that NO is an inducer of NETosis and acts via augmenting free radical generation. It has been suggested that oxidative stress and iNOS induction may act in concert during inflammation to promote NETosis (Patel et al. 2010).
5 Reactive Oxygen and Nitrogen Species in Cell Signaling Pathways
Nuclear factor kappa B (NF-κB) is a transcription factor that once activated regulates the process of transcription of numerous genes. Their products (e.g., cytokines, protein p53, growth factors, and cell adhesion molecules) are involved in cell cycle regulation, inflammation, and immune response (Celec 2004). The influence of ROS on NF-κB regulation varies in different compartment of a cell. ROS in the cytoplasm stimulate the signal transduction pathways for NF-κB activation and translocation into the nucleus. On the other hand, ROS inhibit the activity of this transcription factor in the nucleus. Oxidative and nitrosative stress may regulate NF-κB pathway in many ways. The sulphydryl group of Cys62 in NF-κB molecule is a determinant of DNA recognition by the p50 subunit and it is especially sensitive to oxidation (Matthews et al. 1993). It cannot be excluded that oxidation is followed by the S-glutathiolation, as changes in the GSH/GSSG ratio are responsible for inhibition of binding of p50 subunit to DNA (Klatt and Lamas 2000). It has also been shown that NF-κB/RelA Ser(276) phosphorylation induced by tumor necrosis factor-α (TNF-α), a modification critical for NF-κB transcriptional activity, is mediated by the ROS signaling pathway (Jamaluddin et al. 2007).
Several studies highlighted that ROS affect the phosphorylation of I-κBα and independently induce NF-κB activation and translocation to the nucleus (Schoonbroodt et al. 2000; Takada et al. 2003). Contradictory findings on the influence of ROS on IKK have been reported. Some studies show that ROS activate IKK kinase complex, the core element of the NF-κB cascade, while others found that ROS may have an inhibitory effect on this kinase (Byun et al. 2002; Herscovitch et al. 2008). Moreover, catalytically active subunit β of IKK is a site for S-nitrosylation by nitric oxide. Following inhibition of NF-κB provides an explanation for anti-inflammatory effect of NO (Reynaert et al. 2004). As mentioned above, release of NO down-regulates neutrophil migration, as it decreases rolling and adhesion of neutrophils to endothelium and induces apoptosis (Dal Secco et al. 2003).
Oxidants may also modulate cell signaling pathway involving the mitogen-activated protein kinase (MAPK) family. They are activated to induce the production of cytokines, like Il-6, and inflammatory mediators (Craig et al. 2000; Lang et al. 2006). Exogenous oxidants induce tyrosine phosphorylation and activation of ERK, which is a member of MAPK. This effect is enhanced by the inhibition of CD45, which also is a result of oxidative influence. Oxidized CD45 cannot efficiently dephosphorylate and inactivate MAP kinase (Fialkow et al. 1994). It has been recently demonstrated that PMA activates both extracellular signal-regulated kinases (ERK) and p38 MAPK and the process of phosphorylation of these two kinases is ROS-dependent (Keshari et al. 2013).
ROS are capable of regulating the activity of protein tyrosine kinases and phosphatases. The balance between these two activities is responsible for the level of tyrosine phosphorylation. Signaling pathways involving these enzymes are of great importance for neutrophil inflammatory response and its components, such as adherence, chemotaxis, and phagocytosis (Kobayashi et al. 1995). Invariant cysteine residue in protein tyrosine phosphatase superfamily (PTP) functions as a nucleophile during catalysis. The active site Cys may be oxidized, which abrogates its nucleophilic properties and inhibits PTP activity. When oxidation by ROS occurs upon activation, it leads to inactivation of PTP and facilitates the response to the activating stimulus (Rhee et al. 2003). It has also been found that oxidative burst can inhibit expression of CD45, a tyrosine phosphatase commonly found on the surface of leukocytes. This inhibition is, at least partially, mediated by NOX (Fialkow et al. 1997). Cysteine residue is also a target for oxidants in protein kinase C (PKC). This family of proteins is responsible for regulation of cell growth, differentiation, apoptosis, transformation, and tumorigenesis. Cysteine is located within the zinc-binding domain of autoinhibitory function and when oxidized it leads to the activation of PKC (Cosentino-Gomes et al. 2012).
6 Reactive Oxygen/Nitrogen Species and Neutrophils in Pathology
It is known that patients with human immunodeficiency virus (HIV) suffer from systemic oxidative stress. It leads to the activation of NF-κB and, as a consequence, increases the HIV replication (Nakamura et al. 2002). Increased ROS production is due to changes in the expression of Bcl-2 and thioredoxin, antiapoptotic and antioxidant molecules. Spontaneously increased production of ROS by phagocytes (both macrophages and neutrophils generate high amounts of H2O2) can participate in the oxidative injury in the course of HIV infection. Despite the production of hydrogen peroxide by neutrophils, these cells show decreased release of ROS after the specific stimulation when exposed to oxidative burst inducers such as TNF-α, Il-8, or bacterial N-formyl peptides. That may contribute to the enhanced susceptibility to bacterial infections in HIV-infected patients (Elbim et al. 2001).
In patients suffering from acute respiratory distress syndrome, bronchoalveolar lavage contains a high number of neutrophils, which are a source of inflammatory mediators and superoxide anion. Peroxynitrite generated from NO and superoxide, nitrates, and oxidized amino acids inhibit function of proteins, such as surfactant protein A. Improper balance between antioxidants and reactive oxygen and nitrogen species aggravates the disease (Lang et al. 2002). Oxidant/antioxidant imbalance is observed also in patients with chronic obstructive pulmonary disease (COPD). Numerous neutrophils in the airways of these patients produce oxidants and the amount of free radicals is additionally increased in patients smoking cigarettes. Evidence for enhanced oxidative stress in smoking patients are also supported by the presence of nitrated proteins: ceruloplasmin, plasminogen, fibrinogen and transferrin, as well as elevated levels of lipid peroxidation products in blood (Morrison et al. 1999; Pignatelli et al. 2001). Oxidants can activate NF-kB, which, in turn, causes transcription of inflammation-related mediators. That results in increased sputum concentration of TNF-α, nitric oxide, and IL-8. Oxidation also silences α-1-antitrypsin, disturbing the balance between proteases and antiproteases, which causes tissue injury (Dalle-Donne et al. 2005). It has been shown that during acute exacerbation of COPD the production of superoxide by neutrophils is increased (Rahman et al. 1997).
In rheumatoid arthritis, neutrophils present in inflamed joints produce both ROS and RNS, which can exacerbate the disease. Nitrotyrosine, which is a marker of peroxynitrate production, is elevated in serum and synovial fluid collected from patients with the disease and the level of 3-nitrotyrosine correlates directly with disease activity (Kaur and Halliwell 1994; Khan and Siddiqui 2006). The level of S-nitrosoproteins in synovial fluid is especially high when compared with plasma concentrations (Hilliquin et al. 1997). This supports a notion of NO being formed by inflammatory cells within the joint. Superoxide and hydroxyl radicals are also significantly raised in peripheral blood and synovial infiltrate collected from rheumatoid arthritis patients. ROS can serve as an indirect measure of the intensity of inflammation (Kundu et al. 2012). Similarly, superoxide dismutase activity is higher in the joint fluid of these patients than in healthy subjects and its activity may serve as a valid index of articular destruction and repair (Sumii et al. 1996).
Patients with ulcerative colitis have an elevated neutrophil count when compared with healthy subjects (Hanai et al. 2004). Moreover, polymorphonuclear granulocytes accumulate in the epithelial crypts of the intestinal mucosa in inflamed bowel. Increased production of ROS by neutrophils leads to tissue destruction (Ramonaite et al. 2013). It has been suggested that oxidative stress damages DNA in a damage-regeneration cycle and enhances the risk of carcinogenesis in ulcerative colitis patients (Seril et al. 2003). In patients with Crohn’s disease, neutrophils respond to stimulation less than in healthy subjects. Superoxide and lysozyme release by neutrophils, a possible mechanism limiting the extent of inflammation in the intestinal wall, is significantly reduced in patients with active Crohn’s disease (Maor et al. 2008).
ROS may also participate in the pathogenesis of preeclampsia, where oxidative stress contributes to endothelial dysfunction. It is suggested that generation of ROS and RNS is caused by reduced blood flow through the placenta. Neutrophils, in line with macrophages, can be activated by oxidative stress, inflammatory agents, or hypoxic conditions during their passage through placental blood vessels. Once activated, polymorphonuclear granulocytes are a source of ROS (Dalle-Donne et al. 2005). Neutrophils are more intensively adhering to the endothelium and infiltrating the intimal space of systemic blood vessels in preeclamptic compared to normal pregnant and non-pregnant women (Cadden and Walsh 2008). It has been shown that supplementation with vitamins C and E in women at increased risk of preeclampsia may be of benefit (Chappell et al. 1999).
7 Conclusions
The role of reactive oxygen and nitrogen species in the physiology of neutrophils is complex. These compounds are potent antimicrobial factors killing bacteria via oxidation, nitration, and nitrosylation. It is also evident that these cells regulate the immune response and the intercellular communication. Nonetheless, production of oxidants by polymorphonuclear phagocytes has been shown to exacerbate several diseases. Thus, further investigation is warranted to discern pathologies that dependent on nitrosative and oxidative insults.
Conflicts of Interest
The authors declare no conflicts of interest in relation to this article.
References
Brill A, Fuchs TA, Savchenko AS, Thomas GM, Martinod K, De Meyer SF, Bhandari AA, Wagner DD (2012) Neutrophil extracellular traps promote deep vein thrombosis in mice. J Thromb Haemost 10:136–144PubMedCentralPubMedCrossRef
Brinkmann V, Reichard U, Goosmann C, Fauler B, Uhlemann Y, Weiss DS, Weinrauch Y, Zychlinsky A (2004) Neutrophil extracellular traps kill bacteria. Science 303:1532–1535PubMedCrossRef
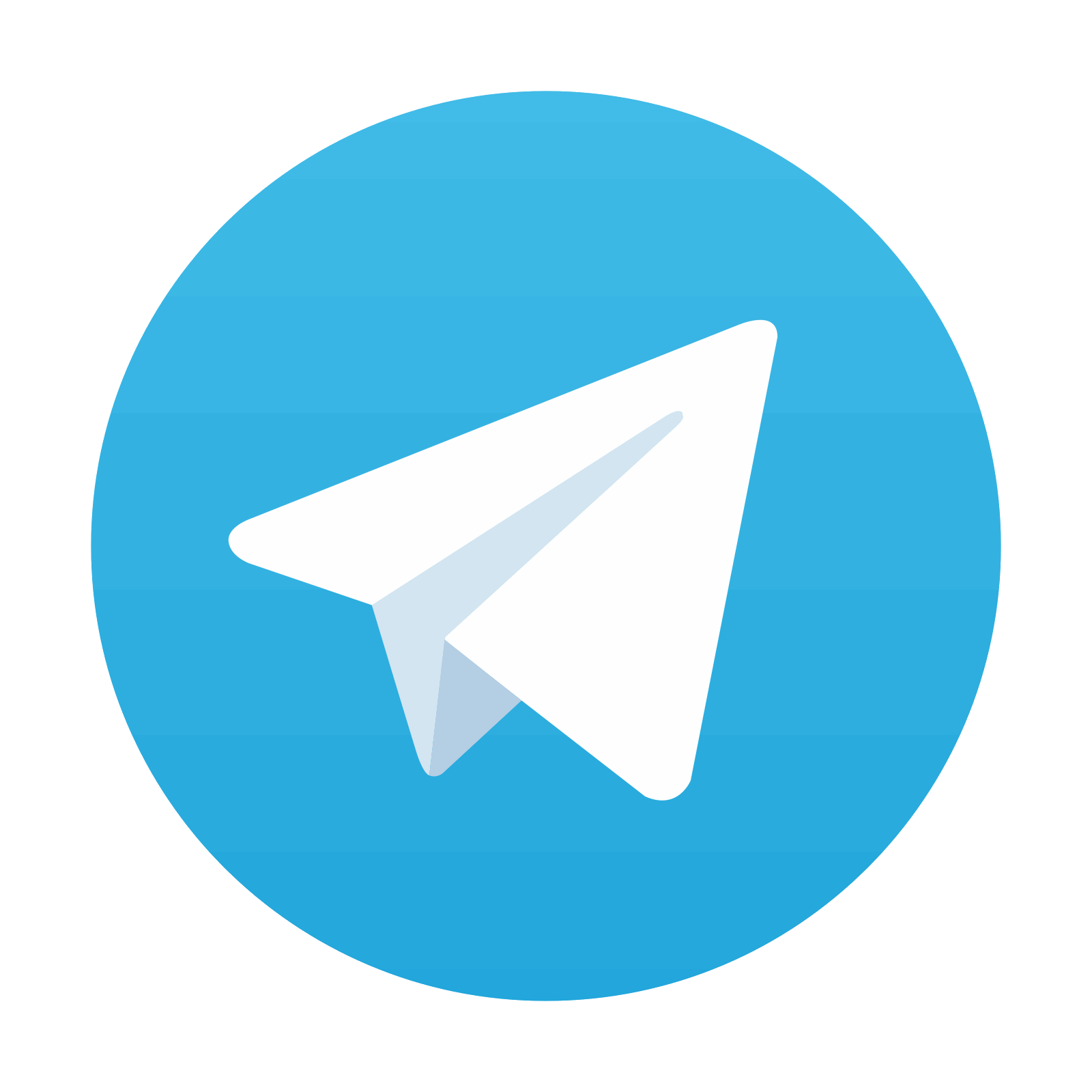
Stay updated, free articles. Join our Telegram channel

Full access? Get Clinical Tree
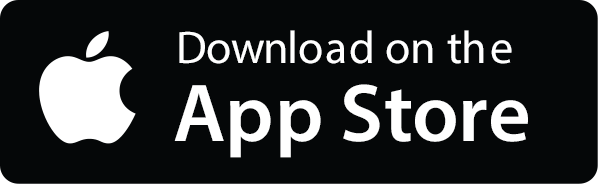
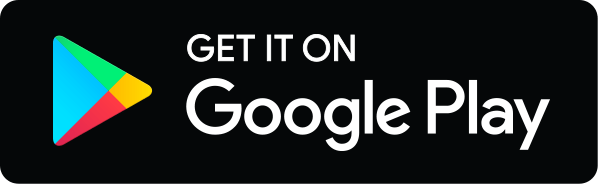