9.2.2 MicroRNAs in Cardiac Hypertrophy
Numerous studies have assessed the involvement of miRs in cardiac hypertrophy (HYP). For example, van Rooj et al. [25] report the overexpression of miRs 23a and b, 24, 195, 199a and 214, and the underexpression of 150 and 181b in hypertrophic hearts. miR-208 has been shown to regulate β-MHC as a response to stress, but not during normal development. Furthermore, Cheng et al. [26] found that approximately 50 miRs were up- and 52 downregulated following TAC. According to van Roooiij et al. [27] miR-208 knockout mice had blunted HYP and fibrosis in response to transverse aortic constriction (TAC), while αMHC was increased, as well as the thyroid hormone receptor associated protein 1, together with absence of hypertrophy or fibrosis. Yang et al. [28] describe that Angiotensin II- induces hypertrophy (HYP) through miR-98/let-7 upregulation. They also point out that cyclin D2 which enhances postnatal growth is downregulated in this process.
Kalozoumi and Sanoudou (24) consider the following to be pro-hypertophic: -195, -23a, 23b, 24, 214, 211, 129, 212, 199a and b, 27b, 208a, 499, while 1 and 133 are antihypertrophic. It is interesting that 199b and 27b affect both HYP and heart failure, while 208a and 499 both HYP and arrhythmias, (also see Latronixo et al. [16]). Influences are signalled by diverse and multiple pathways enumeration of which lies outside the scope of this review.
Significant miR changes related to cardiac HYP, can also be detected in blood circulation. For example, Roncarati et al. [29] showed that in patients with hypertrophic cardiomyopathy 12 miRs were increased in the plasma; however, only miRs 199a-5p, 27a, and 29a were correlated with left ventricular (LV) hypertrophy, while only the latter was associated with both HYP and fibrosis.
9.2.3 MicroRNAs in Atherosclerosis
Extensive work has unveiled a major role of miRNAs in atherosclerosis (ATH). A brief outline on the findings relating to peripheral artery (PAD) and coronary artery disease (CAD) are presented herein.
As Papageorgiou et al. [12] and Tousoulis et al. [30] point out, miRs influence atherosclerosis through many different molecular mechanisms, including angiogenesis, vascular smooth muscle cell (VSMC) proliferation, inflammation, and apoptosis (APO), which characterize vascular plaque vulnerability.
For example, miR-92a is an endogenous repressor of the endothelial cell (EC) angiogenic program along with miR-21, 17, 221, 222 and 24. Meanwhile many miRs have been reported to affect VSMC growth and proliferation, such as the inhibitor miR-33. Inhibition of miR-26 promotes SMC differentiation miR-21 is induced by shear stress and decreases apoptosis (APO) by upregulation of endothelial nitric oxide synthase (eNOS) [12]. As regards their influence on the phenotype of VSMCs, miRs 143 and 145 are critical for maintaining their contractile phenotype. They are downregulated in “synthetic” VSMCs.
MiRs 143 and 145 can prevent vascular remodeling and restenosis. Overexpression of miR-145 leads to reduced neointima formation in balloon-injured arteries; miR-221, 222 and 21 are upregulated in neointimal lessions. Knockdown of miR-221 and 222 suppresses VSMC proliferation and neointimal lesion formation after carotid angioplasty in rats. The same is seen after downregulation of overexpressed miR-21.
Another aspect by which miRs are involved in ATH is their influence on inflammation. MiR-126 decreases VCAM-1 expression and leukocyte adhesion to EC. Consistently with these reports, miR-126 is considered a master regulator of endothelial function; it is highly enriched and actually expressed only in ECs. Its targeted deletion causes vessel leakage and hemorrhages, while its expression preserves vascular integrity by inhibiting inflammatory mediation such as VCAM-1. It also upgrades modulation of stromal cell-derived factor-1, which promotes the recruitment and mobilization of progenitor cell to the ischemic region. It has long been known that fatty meals cause an exaggerated postprandial increase of triglycerides (TGL) which is atherogenic. Sun et al. [31] describe that increased TGL and expression of the VCAM-1 receptor which promotes monocyte adherence to the endothelium, is mediated through increased miR-126 activity. Furthermore, miR-31 and miR-17-3p exert an anti-inflammatory action by regulating the TNFα induced expression of E-selectin and intercellular adhesion molecule-1 (ICAM-1) in EC [32].
The multi-faced nature of the atherosclerotic vascular involvement is further stressed by Zampetaki and Mayr [33], who point out that the metabolic derangements leading to ATH and coronary artery disease CAD are associated with miRs related to increased cholesterol synthesis but also reduced cellular cholesterol export. For example, miR-122 inhibition reduces total cholesterol (by decreasing its synthesis) and increases hepatic fatty acid oxidation; miR-122 is regulated by miR-370. Chen and Juo [34] note that it is the most abundant miR in the liver (75 % of total miR expression). Its deficiency in various strains of transgenic mice results in a ≈ 30 % reduction of total cholesterol and TGL levels. Its inhibition leads to a reduction of fatty acid liver content and synthesis.
Chen et al. [32] describe the miR-33 involvement in atherosclerosis; its inhibition increases HDL levels in African green monkeys; miR-33 also reduces fatty acid oxidation, increases intracellular TGL levels and promotes hepatic TGL synthesis. Its overexpression increases the level of hepatic bile acids. It also reduces the production of IL-1β and TNF-α, serving as an anti-inflammatory agent in macrophages. It also inhibits the activation of downstream insulin-signaling pathways. Torella et al. [35] point out that while miR-1 vascular levels are negligible, miR-133 is robustly expressed in VSMCs and has protective actions. Actually, miR-133 reduces but anti miR 133 exacerbates VSCM proliferation and migration.
Norata et al. [36] reviewed the relationship of miRs and lipoproteins. They describe that miR-33, and 122, 106, 758, 26, 370, 378, let-7, 27, 103, 107, 34α, 143, and 335 finely regulate lipid metabolism and progression and regression of ATH.
They further note that miR-33b is not conserved in rodents, which may explain some of the differences between rodent models of ATH and humans. They repeat that its inhibition leads to an increase of plasma HDL and regression of ATH, while it also increases the degradation of VLDL. Overexpression of miR-33 significantly inhibits insulin signaling. Thus, anti-miR-33 therapies may be an attractive therapeutic approach.
Importantly, circulating miRs may not act only as markers but also as effectors: LDL and HDL have been shown to transport miRs [37]. Affecting their bioavailability, and probably their contribution to immunity. In this context Wang et al. [38] have shown that repression of miR-10b promotes reverse macrophage cholesterol transport in mice.
Ma et al. [39] studied miR-155, a multifunctional miRNA that is expressed in the ECs, where it suppresses the expression of Angiotensin II type 1 receptor, a modulator of expression of inflammation. Interestingly, miR-155 is also expressed in the macrophages and atherosclerotic plaques, where it is induced by oxidized LDL. Furthermore, it seems to negatively modulate inflammation, and confer protective effects in VSMCs.
One can only marvel at this huge array of molecular players and realize the complexity of attempting atherosclerotic treatment through miRNA manipulation.
9.2.4 Action of microRNAs on Platelets
Stakos et al. [37] have written a pertinent review on this role of miRs, pointing out that human platelets express high levels of megakaryocyte-derived miRs. They mention that in 2008 it was first reported that the platelets of patients with polycythemia vera and essential thrombocythemia had a higher expression of miR26-b in their platelets. The authors further describe in detail how miRs regulate platelet function: The P2Y12 receptor plays a central role in platelet activation; miR-223 represses its gene expression; miR-200b may keep platelets in a hyporeactive state. MiR-107 targets and reduces the mRNA of the circadian locomotor output cycles Kaput (CLOCK) which regulate circadian rhythm in many species. It may be associated together with increased plasma von Willebrand factor levels with a pro-thrombotic state.
Further associations include: Platelet coagulation and wound healing are impeded by miR-17 overexpression due to fibronectin inhibition; miR-29 is associated with a decrease in fibrogen production; miR-96 decreases platelet reactivity; while miR-495 is associated with platelet hyporeactivity to epinephrine. The angio-miRs-126, -21 and -210 appear to have a very interesting role as well. MiR-126 which is endothelial specific is also proangiogenic miRNA, which is also abundant in platelets, suggesting that platelets may communicate with ECs. miR-200b prevents platelet activation by suppressing CAMP-dependent protein kinase II-β regulation subunit gene expression in the megakaryocytes.
Zampetaki and Mayr [33] stress that platelets contain abundant quantities of small miRs, resulting into a higher miR/mRNA ratio as compared to nucleated cells. They cite miRs 223, 123, 103, let-7, 21, 107, 24 and 222.
Tousoulis et al. [30] provide a detailed list of miRs involved in the atherosclerotic process which in many ways affect various processes:
Platelet activation (17, 24, 191, 197, 223)
Nitric oxide (21, 155, 222/222, 214, 217), apoptosis (21)
Angiogenesis (28b, 130, 217, 222/222, 378)
VSCM proliferation (21, 143/145, 222/222)
Inflammation (125a/b, 221/222) adhesion molecules (17–30, 31), and
Collagen formation (133), among others.
9.3 MicroRNAs in Diabetes Mellitus
MiRNAs have been implicated in diabetes through multiple mechanisms: they contribute to insulin resistance, impaired angiogenesis, and diabetic cardiomyopathy. Shantikumar et al. [40] studied the role of miRs in diabetes and its cardiovascular complications through a variety of diabetic animal models i.e. rats, mice, rabbits, as well as humans, and in various tissues, such as skeletal and cardiac muscles, liver, adipose tissue, omentum, subcutaneous fat, ECs and retina. The following miRs were found to be upregulated: 29 family (a/b/c), 103, 125a,b, 195, 27a, 221, 222, 181a, 147, 197, 320, 503, 1, 206, 206b, 146, 155, 131, 21, 133. In contrast the following were found to be downregulated: 24,10b, 17-5p, 132, 134, 27a, 30e, 140, 155, 210, 133a, 200b.
Mortuza and Chakrabarti [41] recently also investigated the role of miRs in diabetes. They found that miR133a downregulation in chronically diabetic rat hearts was associated with SGK1 and IGF1 upregulation which induce HYP. MiR-133 however, has been described as either increased or decreased across different studies, which could be only partly explained by the diversity of conditions examined. Some points should be stressed: miR-133 is downregulated in the myocardium according to some authors but upregulated according to others. This upregulation caused a reduction of the G protein levels with resultant QT prolongation. It can also be the cause of hypertrophy in diabetic cardiomyopathy [42].
Baseler et al. [43] studied the myocardium of mice rendered diabetic after streptozotocin treatment and identified 26 upregulated miRs, notably miR-205, -141, 208b, and 200c. They comment on the role of miR-141 as a regulator of the production of ATP for the inner mitochondrial membrane.
According to Zampetaki and Mayr [33], multiple miRs are also involved in glucose homeostasis. For example, miR-375 suppresses insulin secretion, while miRs -9, -96 and -124a fine-tune insulin release. Importantly, while miR 124 promotes insulin secretion under basal conditions, it attenuates it in response to glucose stimulation. MiRs-34a and -146 induce b-cell APO, while miRs-103 and -107 are negative regulators of insulin sensitivity. The let-7 family of miRs, when blocked, promotes an insulin-sensitized state.
These authors also describe the role of miRs in glucose homeostasis. They point out that knockdown of miRNAs-24, 26, 182 and 148 in cultured b-cells or in isolated primary islets suppresses insulin promoter activity and insulin mRNA levels. Furthermore, miR-37 suppresses insulin secretion, while miRNAs-103 and -107 are negative regulators of insulin sensitivity, while miR-34α and -146 induce b-cell APO. According to the authors miR-9 is mandatory for optional secretory capacity of b-cells, while miR-96 increases granuphilin which enhances basal but inhibits K1- induced insulin secretion miRs in the metabolic syndrome (MS):
Pulakat et al. [44] focused on cardiac insulin resistance, and described how miR-143 inhibits insulin-stimulated Akt activation, while miR-122 dampens insulin signaling, consistently with aforementioned studies.
Feng et al. [45] found that let-79 and miR-221 were higher in the MS prominently in females.
9.3.1 Obesity
Obesity is a condition closely associated with diabetes. Jordan et al. [46] have shown that in this state, overexpression of miR-143 inhibits insulin-mediated Akt activation and impairs glucose metabolism. Of note, Wang et al. [47] found that let-7 and miR-221 were increased in patients with the metabolic syndrome and particularly in women.
Blumensalt et al. [48] found that Activin A which is released from epicardial adipose tissue inhibits insulin action on cardiomyocytes through the induction of miR-143 which inhibits the Akt pathway.
9.3.2 Senescence
Another aspect of ATH is endothelial senescence (SENSC). Menghini et al. [49] mention the following miRs involved in SENSC: mIR-34, -217, and -146: miR-34 is abundantly expressed in EC and increases with SENSC.
MiR-217 is preferentially expressed in aged but not in young cells.
These 2 miRs directly inhibit Sir T1 which plays a protective role in the endothelium, with eNOS as one of its main targets. SirT1 activity is also essential for maintenance of TIMP3 expression, which antagonizes MMPs. As already stated, miR-126 inhibits expression of inflammatory mediators such as VCAM1; miR-92α is an endogenous repressor of the angiogenic program in EC; its inhibition improves functional recovery in hindlimb ischemia and acute myocardial infarction. MR-21 is a negative regulator of angiogenesis miR-146α decreases with SENSC. It results into an increase of NOX4 protein, which induces the reduction of molecular oxygen to ROS in the vessel walls, further promoting SENSC.
The authors describe that on the whole miRs34a, 217 and 92a promote while miRs126 and 146a inhibit SENSC and ATH.
Dimmeler and Nicotera [50] note an age induced increase in vascular miR-29, 34α, 146, 217. They also note that in cardiac tissue miR-21 is increased, together with 22 and 24, and 18 and 19 are decreased in age-induced fibrosis.
Finally, Olivieri et al. [51] describe that circulating inflammatory miRs in age-related diseases are related to diabetes mellitus, cardiovascular disease and Alzheimer’s disease.
9.4 Coronary Artery Disease
Tousoulis et al. [30] and Papageorgiou et al. [52] give an account of miRs involved in stable coronary artery disease (CAD). They point out that miR-126, -17, 92a and 155 (which is associated with inflammation) are downregulated in CAD. MiR-126 was found to be intensely related to LDL cholesterol by Sun et al. [53] who however did not find differences in its levels between patients with CAD and controls.
Fichtlscherer et al. [54] in a small group of eight patients with stable CAD versus 8 controls found 46 significantly downregulated and 20 upregulated miRs. They further analyze their findings in a larger numbers of patients. The circulating levels of endothelial-expressed miRs 126, 92a and 17 were significantly reduced, as well as the vascular smooth muscle enriched miR-145 and the inflammatory miR-155. A trend towards an increase of cardiac-muscle enriched miR-208a and -133a was seen.
Van Aelst and Heymans [55] cite the following miRs as biomarkers for asymptomatic CAD, as regards the serum levels:
Increased: 133a, 135a. 134. 370, 140, 140-3p, 182, 92a,b.
Decreased: 126, 17. 92a, 199a, 155, 145, 24, 21, 206, 15a, 191, 197, 223, 320, 486.
They remark that miR1 and 133a are abundantly expressed cardiac miRs as well as miR208a/b which are derived from the introns of a- and β-MHC. Further associations are beyond the scope of this review.
Zhu and Fan [56] point out that the reduced concentration of circulating vascular miRs detected in patients with CAD may seem paradoximal but it could be due to an increased uptake of circulating miRs into the widely disturbed atherosclerotic lessons.
Concerning the prognostic role of microRNAs we cannot conclude as the prospective studies are limited. Zampetaki et al. [57] based on the Brunech cohort of 820 participants followed up for 10 years concluded that 3 miRNAs were consistently and significantly related to incident myocardial infarction: miR-126 showed a positive association, whereas miR-223 and miR-197 were inversely associated with disease risk [pmid 22813605].
9.4.1 Acute Myocardial Infarction (AMI)
The main and most dramatic manifestation of CAD is the emergence of a severe acute myocardial infarction (AMI). A large number of miRs have been associated with AMI during the last year.
Fiedler and Thum [58] in a very recent review point out the following in rat models:
miR-1 is overexpressed in ischemic myocardium. Its role in heart failure (HF) will be discussed later.
miR-15 is upregulated in the infarct and border zone in rat and porcine models of AMI.
miR-24 is increased in EC at hypoxia and cardiac ischemia; this miR is also pro-apoptotic.
miR-29 is highly abundant in fibroblasts. Its role in post-AMI cardiac remodelling (REM) will be discussed later.
miR-29a is increased in hindlimb ischemic and AMI, and is antiangiogenic.
miR-101 is chronically downregulated in this peri-infarct zone,
miR-126 is involved in endothelial function and integrity.
miR-214 is upregulated in HF.
As the aforementioned authors imply, most of these miRs can constitute therapeutic targets in AMI.
9.4.1.1 Circulating microRNAs in AMI
An important role has also been described for circulating miRs, which could serve as novel biomarker in acute coronary syndromes (ACS). For example, Pleister et al. in a detailed review [59] site the increased miRs: 1,133, 133a, 208a and b, 499-5p, 499, 122, 126, as well as the decreased: 122, 375, 663b, and 1291. It is postulated that these miRs originate mainly from the injured myocardium.
In the already mentioned review of Van Aelst and Heymans [55], the following miRs are cited in acute coronary syndromes (ACS):
Increased: miR1, 133a and b, 499-5p, 208b, 328, 21.
Decreased: miR122, 375, 126.
Nabialek et al. [60] studied three miRs (425-5p, 208a and 1) in AMI and stable CAD. They found an early (<6 h) increase of miR-423-p, normalizing at 6 h.
In stable CAD pts there were no differences.
Several studies have also aimed at identifying circulating miRs that could serve as biomarkers of AMI. Among the different miRs that have been proposed as promising in that respect are miR-1, -133a, -208a, -208b, -499 and -499-5p, which appear significantly changed predominantly during the first few hours post AMI [61]. Serum levels of miR-1 have been associated with infarct size.
Also, Olivieri et al. [62] found that miR-499-5p increased 80-fold in non-ST elevation myocardial infarction; its diagnostic value was higher than hs and classical cTnT.
Importantly miR-208a which is encoded by an intron of the aMHC gene is the only heart-specific miR, while miR-499,1 and 133 are also expressed in skeletal muscle.
Presumably the same miR profile could emerge in myocardial injury after a precutaneous coronary intervention (PCI). However, few studies on this subject exist. Recently Yu et al. [63] found that miR-126 after PCI was associated with a worse prognosis in patients under dual antiplatelet treatment, up to 1 year. D’ Alessandra et al. [64] gave a detailed review. In patients with coronary reperfusion after PCI they found increases in miR1, 133a and b, 499-5p, while miR-122 and 375 were decreased. Their patients with thrombolysis were too few for meaningful comments.
Jaguszewski et al. [65] very recently found that a unique signature comprising miR-1, -16, -26a and 133a differentiated Takotsubo cardiomyopathy from healthy subjects and from patients with AMI.
They also found a correlation with pressure and duration of balloon inflation and consider it as a new target of presentation and treatment of endothelial dysfunction. Polimeni et al. [66] give a recent review in this subject. They describe how miRs regulate VSMCs and ECs after vascular injury. The former are responsible for in-stent restenosis. They describe many miRs affecting VSMC regulating either the pro-contractile (1, 133a, 143, 145), and the pro-synthetic phenotype (26a, 31, 146a, 221, 24 222). MiR21 can be either pro-contractile or prosynthetic. They also describe miRs implicated in various ways in EC function, including 126, 217, 17-as, 17–50, 18a, 19a, 20a, 92a, 221/222 and 663. All these can be the target of future therapeutic interventions.
Lu et al. [67] comment on another aspect: they observed that miR-1 overexpression in ischemic myocardium was reversed by propranolol which also had beneficial cardiac effects. This was likely achieved through the inhibition of the β-adrenoreceptor-CANP-protein, kinase A signaling pathway by propranolol, which could regulate miR-1 expression.
9.4.1.2 Prognostic Value of microRNAs After an Acute Myocardial Infarction
Eitel et al. [68] assessed the relation between miR-133a myocardial damage by CMR and prognosis at 6 months, in 216 consecutive patients. They found a significant correlation of infarct size, microvascular obstruction, and myocardial salvage index with miR133a, however its concentrations could not independently predict clinical events.
Matsumoto et al. [69] attempted to identify circulating miRs as predictors of CHF in post-MI patients. They collected sera ~18 days after MI onset, in 21 patients followed for 1 year. The levels of 3 miRs which are p53 responsive miRs, 192, 194 and 349 were upregulated.
In a similar context, miR146a was up-regulated and miR-150 and -155 were down-regulated in post-MI ventricular rupture; these miRs are involved in innate immunity [70].
Further considerations on these associations will be given when heart failure is discussed.
9.4.2 MicroRNAs in Cell Death
The work of Matsumoto et al. [69] has been already mentioned.
The tumor suppressor p53 is an inducer of the APO pathways, partly through the co-ordinated induction of multiple miRs expression.
According to Hullinger et al. [71], the miR-15 family is increased in the heart within 24 h after I/R. Its inhibition reduced cardiomyocyte cell death and improved mitochondrial function, since this family targets Bcl-2 and the mitochondrial protective protein ADP-ribosylation factor like 2.
Inhibition of the pro-apoptotic miR-34 family, which targets SIRT1, had the same effects.
According to Aurora et al. [72] miR-214 protects the mouse heart from I/R injury by controlling Ca2+ overload by repression of the mRNA encoding the sodium/calcium exchanger 1, a key regulator of Ca2+ influx.
Orogo and Gustafsson [73] describe the following associations between miRs and cell death (CD): miR-21, 199α, 210 and 494 promote cardiomyocyte survival during ischemia. miR-1 reduces the anti-apoptotic Bcl-2, while miR-214 deletion increases apoptosis after I/R.
In cell loss, miR-1 and miR-133 have opposing roles, the former promoting it through increased APO through HSP60 and 70 suppression, which the latter decreased APO by decreasing caspase-9 protein levels and activity.
Ruan et al. [74] suggest that miR-23α may be involved in TNF-α induced EC APO through the caspase -7 and 3 pathway activation.
Li et al. [75] have found that miR-150 aggravates H2O2 induced apoptosis in cultured rat cardiomyopathies.
Fu et al. [76] found that let-7 is involved doxorubicin induced myocardial injury.
Also acute doxorubicin apoptosis was associated with miR-146α induced inhibition of the neuregulin-ErbB pathway [77].
9.4.3 Heart Failure and microRNAs
Many miRs have been investigated in CHF. One of the main causes of heart failure is post-MI remodelling (REM) which has already been discussed.
van Rooij et al. [78] stress that miR-92α increases early after ΑΜΙ; its inhibition increases capillary density and improves cardiac function. miR-24 has intriguing actions; it inhibits angiogenesis but it also attenuates infarct size. miRr-29 which is repressed after aortic banding and reduced after AMI since it is antifibrotic, its downregulation in the border zone would promote fibrosis. miR-21 blocking by antagonists prevents cardiac fibrosis. However, it also has an antiapoptotic action and decreases infarct size. miR-101 is profibrotic; It is downregulated after MI. Its forced overexpression inhibited proliferation of cardiac fibroblast and reduced collagen production [79].
Zile et al. [80] first reported that miR29a early post-MI was associated with left ventricular end-diastolic volume at 3 months.
Many additional studies have been devoted to the subject. Devaux et al. [81] noted that miR-150 is a marker for REM.
Divakaran and Mann [82] also describe in depth the role of miRs in REM. They point out an important fact which will explain many of the data to be referred to, that miR-1, let7, miR26a, 30c, 126-3p and 133 are the more highly expressed in the human heart.
They further describe the following associations as regards.
9.4.3.1 Myocyte Hypertrophy
The subject has already been described in Sect. 9.2.2.
Reduced expression of miR-1 and 133 has been found in hypertrophic hearts [24]. The authors comment on the controversial findings as regards miR-21: this miR is upregulated post transverse aortic banding, and increased by the well-known hypertrophy induce, Angiotensin II and phenylephrine; however they also refer to studies in which antisense knock down of miR-21 actually provoked hypertrophy and increased fetal gene expression.
As regards the occurrence of CHF which is the eventual outcome of REM, it is –as already stated in Chaps. 16, 17 of this book, characterized by cardiomyocyte loss mostly through Apoptosis (APO) and Necrosis (N), and changes in the composition of the extracellular matrix (ECM).
An important step in the process towards REM is the inability of angiogenesis to cope with the ensuing hypertrophy. A number of pro- and anti-angiogenic miRs have been found. As already stated, miR-126 knockdown resulted in loss of vascular integrity; this miR also enhances the pro-angiogenic actions of VEGF and FGF [56]. Importantly however, the authors point out that miR126 is increased in the failing human hearts.
As regards to the increase in fibrotic tissue through ECM proliferation, van Rooij et al. pointed out that miR-208 is required for cardiac fibrosis [78].
Topkara and Mann [83], analyzing the role of miRs in cardiac REM and CHF also point out the role of miR-1 as a negative regulator of hypertrophy; miR-133 is down-regulated in many hypertrophy models, while its adenoviral overexpression attenuates hypertrophic growth. However, they point out that transient versus long-term suppression of its expression may have different effects. They also try to elucidate the role of miR-21, repeating that it is up-regulated in the failing myocardium, while its role in cardiac hypertrophy is not convincingly proven, while its antiapoptotic and pro-survival activity is discussed. The authors also describe the role of miR-199a in inducing hypertrophy, while its downregulation leads to hypoxic cell death through up-regulation of the hypoxia inducible factor 1-a (HIF-1a) which stabilizes the pro-apoptotic factor p53.
miR-320 leads to APO-induced CD, while its knockdown by an antagonist leads to reduction of I/R injury. As regards the upregulation of fibrosis, the authors stress the role of miR-21, which increases fibroblast survival and fibrosis; it is upregulated in the border zone of post-Mi hearts, which is characterized by increased fibrosis compared to the remote region.
miR-29 is also downregulated in the border zone, thus promoting collagen deposition. They also point out the already discussed anti fibrotic effect of miR-133a.
miR-208a is also necessary for the development of fibrosis. A detailed review was given 2 years ago by Zhu and Fan [56].
They connect to a large extent the protection against I/R injury to the size of the infarct, which as stressed elsewhere in this book, determines to a large extent the course towards cardiac REM.
They stress the results of many investigations, mainly in rodent I/R models, and the increase of miR-133a at I/R in the rat, while in the human they are both down-regulated.
According to these authors miR-21 has controversial effects on REM; the same holds true for miR-29 and -24, while miR-126, -210, -494 and -499 have protective effects.
They additionally describe the protective effects of the following miRs:
miR-126 as a pro-angiogenic factor; It also upregulates the homing ability of endothelial progenitor cells and to promote myocardial regeneration. Its upregulation decreases REM.
miR-210 is also pro-angiogenic, and anti-apoptotic; as will be discussed further, it mediates ischemic pre-conditioning.
Hu et al. [84] showed that it directly increases myocyte survival and decreases REM.
The most recent detailed review on cardiac REM and HF is given by Kumarswamy and Thum [85].
They underline that the following miRs contribute to ≈ 90 % of total cardiac miRs in order of abundance: 1/206, let 7/98, 30, 29, 26, 378, 143, 24, 133 etc.
These authors also provide a list of “good” and “bad” cardiac-influencing miRs, as follows:
Protective miRs: 1, 101, 133, 142, 144/41, 145, 17, 18, 19, 210, 214, 30, 494, 9.
Detrimental miRs: 132/212, 141. 199b, 208, 21, 22, 221, 23, 24. 34, 350, 98/let-7.
They Provide the Following Data on Cardiac Hypertrophy, HF and REM
miR-208 is important for cardiac hypertrophy; specifically miR-208a is sufficient to induce cardiac REM and β-MHC expression. They cite the work of Sayed et al. [86], who found miR-1 downregulation in pressure-overload cardiac hypertrophy. This miR probably plays a protective role against HF emergence; It is also restored after adenoviral SERCA-2 gene therapy [87].
miR-1 also targets the Na+ –Ca2+ exchanger. miR-214 protects against I/R injury through the repression of this exchanger.
The action of miR-499 are somewhat contradictory and at this stage beyond the scope of this review.
The authors also describe the important action of non-cardiomyocyte specific miRs. As already noted in the Chap. 17 on cardiac REM in this book, fibrosis is a hallmark of REM. In this context, miR-21 promotes, and its antagonism inhibits, fibrosis.
Importantly, transforming growth factor-β which is profibrotic, upregulates miR-21.
miR-12b is highly abundant in EC already described. Its loss may be construed to limit neovascularization in HF. Conversely, it suppresses angiogenesis. Also, miR-24 induces EC apoptosis and inhibits capillary network formation. MiR-23α is prohypertrophic and is actually transactivated by the prohypertrophic NFATC 3; this also transactivates miR-199b which is upregulated in HF. Moreover, deletion of miR-22 accelerates the progression to cardiac dysfunction through a reduction of SERCA2a transporting activity.
A very interesting finding was recently reported in an uncommon but serious cause of HF, peripartum cardiomyopathy. Haghikia et al. [88] found an increase of serum levels of cathepsin-D, an enzyme induced by oxidative stress with proapoptotic properties which generates 16 k Da Prolactin whose target, miR-146α, is also increased in the serum. This is an antiangiogenic miR located in EC; after its release it is absorbed by cardiomyocytes, where it changes their metabolic profile.
Its increase in the serum as a result of an increase of endothelial microparticles. The upregulation of 146a has been discussed.
Papageorgiou et al. [12] describe the utility of miRs in the diagnosis and classification of the HF. Thus, Schipper et al. [89] studied 17 consecutive patients with end-stage heart failure. They found a decrease in miRs-1, 133α 133β, 208; LVAD support led to partial restoration of this expression.
In another paradigm of HF, doxorubicin cardiotoxicity, Vacchi-Suzzi et al. [90] found that it upregulates mirs 208b, 216b, 215, 34c and 367 in rat hearts in their course towards REM.
9.4.4 MicroRNAs in Arrhythmias-Conduction Defects
The more frequent arrhythmia is Atrial Fibrillation (AF).
Wang et al. [91] discuss in a detailed review that miR-133a/b/c, -26a/b -let 7a/b/c/f/g, -24, -125 a/b, -27a/b, -126, -23a/b, -29 a/b, -12, -21, -92, and -100, are the more abundantly expressed in the myocardium. They then describe that AF has a multifunctional causative etiology: Ionic remodelling, structural remodelling, abnormalities of intracellular Ca2+ homeostasis, and enhanced spontaneous activity – which implicate multiple miRNAs at multiple levels.
miR-1 has arrhythmogenic potential.
miR-26 upregulates IK1; it is downregulated in AF. An increase in IK1(inward rectifier K+ current) leads to a shortening of atrial action potential (APD) duration creating the substrate for AF.
miR-328 is upregulated in AF. It enhances vulnerability to AF, diminishes I Cal (L-type Ca2+ currents) in the left atrium and this shortens atrial APD.
miR-133 is upregulated in diabetic hearts and is associated with QT prolongation. It depresses Ikr. It prolongs APD. Its downregulation in AF would be expected to shorten APD.
9.4.4.1 Influence on Apoptosis and Fibrosis in the Genesis of Atrial Fibrillation
Down-regulation of the antifibrotic miR-133 and miR-590 also favors AF through production of atrial structural remodeling. Interestingly they are also downregulated by nicotine [91]. These authors also postulated that other miRs may have a potential role, through their action on fibrogenesis. Thus, miR-208 and -21 are characterized as pro- and miR-29, -30 (and-133, -590, as already mentioned) as anti-fibrotic. In this sense they may affect vulnerability to AF.
Another mechanism is induction of apoptotic cell death, the pro-apoptotic miR-1 and the anti-apoptotic miR-133 could be direct contributors to this pathogenetic phase.
Also, miR-29, -320 are considered to be pro- and -21 and -199α anti-apoptotic. However, Wang et al. [91] stress that the role of these miRs in AF is not proven.
Liu et al. [92] have examined the levels of various miRs in the plasma in AF. They found reduced levels or miR150, which interestingly had an inverse relationship with CRP.
Kim [93] gives a review on the role of miRs in arrhythmias and cardiac conduction;
He shows the action of various miRs on the APD. He cites the work of Zhao et al. [94] who showed that deletion of miR-1-2 caused conduction blocks in mice. Also they note that an increase in miR-1 in ischemic hearts was accompanied by arrhythmogenesis.
Overexpression of this miR resulted in QRS prolongation. QT prolongation is associated with increased miR-133 and miR-1.
Atrioventricular conduction slowing is seen in miR-208α knockdown mice; this miR is required for Connexin 40 expression; miR-1 reduces Connexin 43 protein levels.
miR-1 enhances cardiac excitation-contraction coupling by selectively increasing the L-type Ry R2 channels and is associated with development of arrhythmia [95].
9.4.5 MicroRNAs in the Blood Circulation
A constantly expanding subject is the number of miRs detected in the periphery with new miRs being added every month. Many authors have provided lists of miRs to be found either in plasma, serum, peripheral mononuclear cells, and whole blood. Their content in the platelets has already been described [37]. Four recent reviews are very inclusive [56, 96–98]
According to Kinet et al. [96] miRs are unexpectedly stable, since naked RNA is readily targeted by exonucleases expressed in various body fluids. To achieve this protection, miRs are packaged in microparticles, such as exosomes microvesicles, and apoptotic bodies. They are also protected through their association with RNA binding proteins such as Argonaute 2 or nucleophosmin and also are bound by HDL. Microvesicles are produced from many cells including epithelial endothelial and tumour cells. They are also called microparticles or ectosomes.
< div class='tao-gold-member'>
Only gold members can continue reading. Log In or Register a > to continue
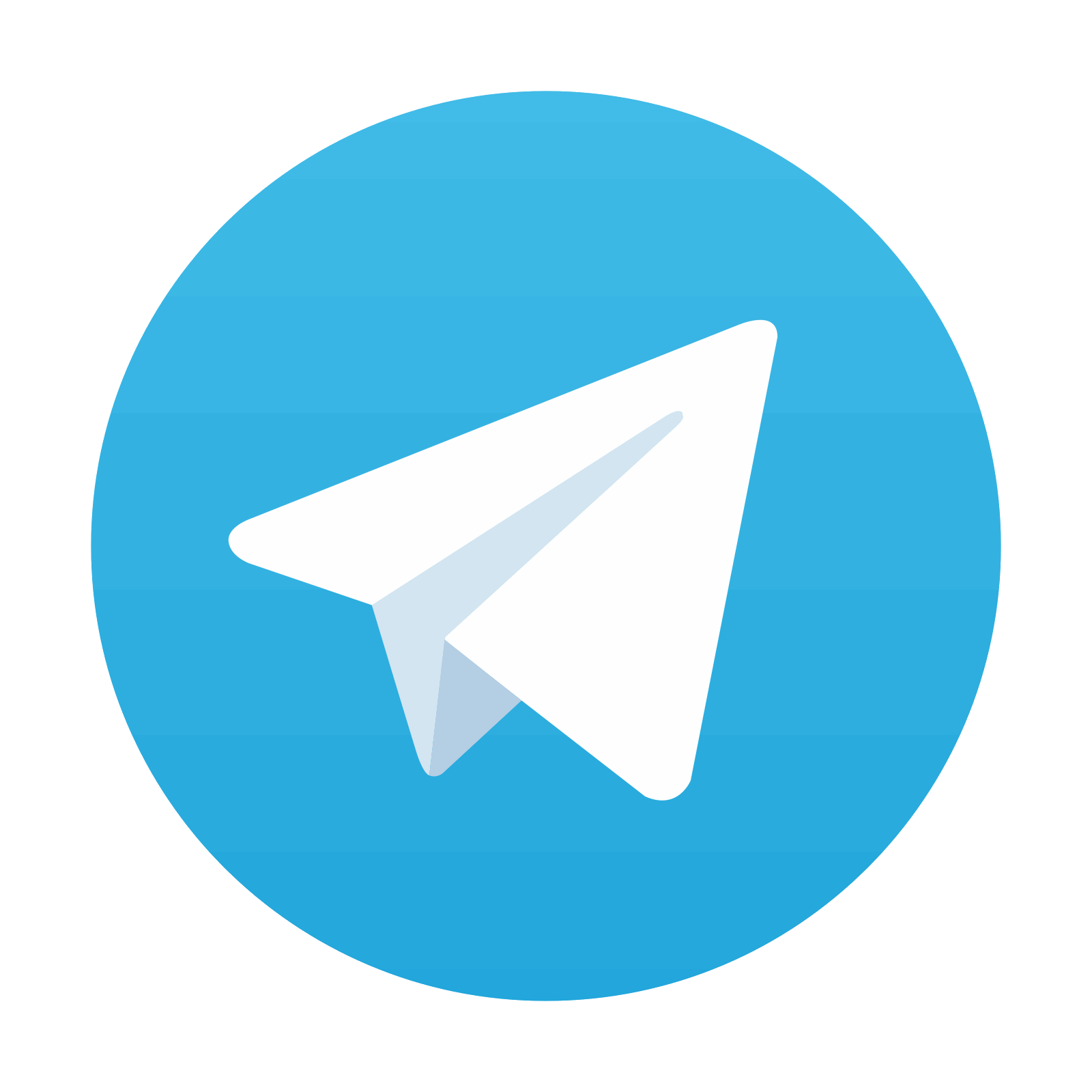
Stay updated, free articles. Join our Telegram channel

Full access? Get Clinical Tree
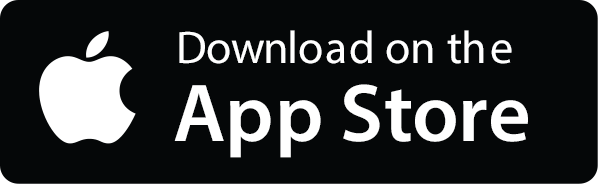
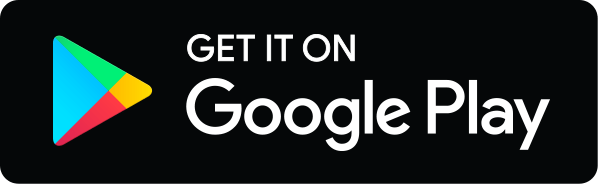