Summary
Exercise testing for cardiac disease in children differs in many aspects from the tests performed in adults; their cardiovascular response to exercise presents different characteristics, which are essential for the interpretation of hemodynamic data. Moreover, diseases that are associated with myocardial ischemia are very rare in young patients, and the main indications for exercise testing are evaluation of exercise capacity and identification of exercise-induced arrhythmias. This article describes the specificity of exercise testing in pediatric cardiology, in terms of techniques, indications and interpretation of data.
Résumé
Le test d’effort chez les enfants cardiaques diffère par de nombreux aspects de celui réalisé chez l’adulte ; leur réponse cardiovasculaire à l’effort présente des caractéristiques dont la connaissance est essentielle à l’interprétation des données hémodynamiques. De plus, les maladies ischémiques sont exceptionnelles chez les jeunes patients et les principales indications du test d’effort sont une évaluation de la capacité physique et l’identification d’arythmies induites par l’exercice physique. Cet article décrit les spécificités techniques, les indications et l’interprétation des données en cardiologie pédiatrique.
Background
Standardized exercise testing has almost become a routine procedure in the care of children with cardiac disease . Impairment of functional capacity is usual in this population and may be the result of the primary cardiac problem, its treatment or hypoactivity. The test is often conducted to provide objective information about exercise capacity, to identify abnormal responses to exercise, to make management decisions, to assess the efficacy of medical and surgical interventions, to evaluate exercise-related adverse events, to define individual safety limits, to instill confidence in child and family, and to motivate patients to engage in physical activity, resulting in improved patient outcomes.
Procedure
An analysis of the possible gain in information expected from the test versus the potential harm induced is mandatory before a test is performed, even if adverse reactions are rare . An exercise test should not be carried out if the patient has an acute infection, acute exacerbation of a chronic disease, or any other unstable health condition that may pose an additional transient risk. Severe obstructive diseases, pulmonary hypertension, severe heart failure or certain arrhythmias warrant special consideration. An experienced physician and emergency equipment should always be present during the test.
According to international recommendations , the test should be terminated when diagnostic findings have been established and further testing will not yield any additional information, when monitoring equipment fails, when signs or symptoms indicate that further testing may compromise the patient’s well-being, and when extreme fatigue or other symptoms of insufficient cardiac output are associated with decrease or failure of heart rate to increase with increasing workload. Other criteria are progressive fall in systolic blood pressure with increasing workload, systolic hypertension > 250 mmHg, diastolic hypertension > 125 mmHg, intolerable dyspnoea or tachycardia, progressive fall in oxygen saturation < 90% or a 10-point drop from resting saturation in a symptomatic patient, 3 mm flat or downward sloping ST-segment depression, triggering of atrioventricular block or QTc lengthening > 500 ms and increasing ventricular ectopy with increasing workload, including a triplet.
Defining that an effort was maximal may sometimes be difficult, but is very important to determine maximal exercise data. A plateau of oxygen uptake (i.e. an increase during the final completed stage of an incremental exercise test of < 2 mL/kg/min for a 5–10% increase in exercise intensity or of < 2 standard deviations of the average increase in oxygen uptake during the preceding stages) occurs in half of the children . In other cases, the investigators have to rely on their impression that a maximal effort has occurred and on indicators such as heart rate > 200/min on a treadmill or > 195/min on a bicycle, breathing reserve < 40% or a respiratory exchange ratio of ≥ 1 on a treadmill or 1.05 on a bicycle .
Exercise testing in children, especially sick young children, is more challenging than in adolescents and adults. Treadmill exercise testing is possible in children from the age of 3 years, but a harness with a rope and one extra-staff member may be necessary to safeguard the child during the test. Nevertheless, owing to the strong resemblance to daily activities, the larger muscle mass involved and the lower probability of leg muscle fatigue, a treadmill test may be especially advantageous in very small or very sick children, as well as increasing the likelihood of the cardiorespiratory system being the limiting factor. Because cycle ergometry requires that the subject maintains a cycle cadence with increasing workloads (especially with a mechanically braked cycle ergometer that cannot vary resistance to keep workload constant over a range of pedal speeds) and because the patient must be big enough to reach pedals, exercise testing on a bicycle is hardly possible before the age of 6 years. Bicycle testing is portable and less expensive than treadmill testing, and accurate determination of the workload is easy. Moreover, the upper body is more stable on a cycle ergometer than on a treadmill, so a bicycle is preferred when the underlying condition requires accurate blood pressure assessment or ischemia detection on an electrocardiogram (ECG). However, many subjects may not be accustomed to cycling and premature muscular fatigue during bicycle testing may prevent them from reaching maximal effort.
Numerous exercise protocols have been used in children. Most laboratories use continuous graded protocols requiring a maximal effort, even if important data can be obtained during submaximal exercise . The choice of protocol depends on the age and body size of the child to be tested, the measurements planned and the equipment available. Hemodynamic and gas exchange responses are better, and determination of the ventilatory threshold is easier in protocols with shorter stage durations, the so-called ramp protocols, whereas a steady state for more physiological functions (heart rate and oxygen uptake) requires exercise stages of ≥ 3 minutes. In general, the total exercise duration should be kept to 6–12 minutes in children, to avoid premature muscle fatigue and lack of attention and motivation. For treadmill exercise testing, most laboratories use the Bruce treadmill protocol , in which increase in work rate is accomplished by increasing speed and grade every 3 minutes. Disadvantages of the Bruce protocol are large interstage increments in work that can make estimation of maximal values less accurate, and intermediate stages than can be either run or walked, resulting in different oxygen costs. Alternatives, with a slower increase in workload, are more appropriate for unfit patients; an example is the Balke protocol, in which the speed of the belt is held constant and the increase in work rate is accomplished only by increasing the slope. Different protocols are also used for cycle ergometry . When an electronically braked cycle ergometer is available, a continuous ramp protocol is usually preferred. Increments in workload can be increased by 5 to 20 W/min, depending on height, weight or body surface area , or can be standardized by 0.25 W/kg/min . Additional procedures are used to answer specific questions, such as the Wingate test protocol to determine anaerobic power, step-like increases of workload to assess oxygen uptake kinetics, or a 4–10-minute intense exercise bout to induce bronchoconstriction.
Exercise testing with ECG monitoring, but without gas analysis, is sufficient when only abnormal blood pressure response, electrocardiographic changes or arterial oxygen desaturation must be detected. Exercise testing is also performed without gas analysis when the patient cannot tolerate the mouthpiece or the facemask (e.g. very young or very stressed children). Endurance time for treadmill protocols and peak work rate for bicycle protocols are then used as an index of exercise capacity. However, their usefulness is limited: the normal range for these variables is quite broad in children, they are influenced by factors unrelated to the cardiopulmonary system, optimal effort cannot be ascertained, and little information is provided regarding the cause of exercise intolerance. Therefore, exercise testing usually includes an expiratory gas analysis and variables relevant to the assessment of cardiopulmonary adaptation to exercise are then calculated from breath-by-breath estimates of oxygen consumption (VO 2 ), carbon dioxide production (VCO 2 ), minute ventilation (VE) and end-tidal partial pressures of oxygen (PO 2 ) and carbon dioxide (PCO 2 ).
Outcome variables
Peak oxygen uptake (peak VO 2 ) is generally seen as the gold-standard variable for assessing aerobic capacity and cardiac function ( Table 1 ). Peak VO 2 , calculated as the average VO 2 over the last 30 seconds of peak exercise, should be used instead of maximal oxygen uptake, as half of untrained children and most heart failure patients do not achieve a plateau phase . Owing to recruitment of more muscle groups, children usually achieve ± 10% higher peak VO 2 values on a treadmill than on a bicycle , and ± 20% higher peak values during upright cycling than during supine cycling , but no difference is noted between similar protocols . Peak VO 2 is usually expressed in mL/min/kg body mass . This expression underestimates the aerobic capacity of overweight and taller subjects.
Variable | Interpretation | Indications |
---|---|---|
Peak VO 2 | ↓ if cardiopulmonary dysfunction or deconditioning | CHD, CM, PAH, potential transplant recipients, complete atrioventricular block |
Maximal heart rate | ↓ if chronotropic insufficiency | Operated CHD, LQTS, transplanted heart |
↓ if efficacy of beta-blockade | CHD/CM with heart failure, arrhythmia | |
ECG | Exercise-induced arrhythmia | CHD, primary arrhythmia |
Ischemic changes | Kawasaki disease, coronary arterial anomalies (congenital or post-repair in Ross or arterial switch operation) | |
Other exercise-induced changes | LQTS, Brugada, WPW, pacing system | |
Oxygen pulse | ↓ if limited stroke volume adaptation | CHD, TCPC, CM |
Oxygen saturation | ↓ if pulmonary disease, intracardiac or intrapulmonary shunts | CHD |
Blood pressure | ↓ if cardiac dysfunction | CHD, CM (hypertrophic CM++), PAH |
↑ if arterial hypertension | Aortic coarctation | |
VAT | ↓ if cardiopulmonary dysfunction or deconditioning | CHD, CM, rehabilitation programs |
VE/VO 2 , VE/VCO 2 slopes | ↑ if ventilatory inefficiency (ventilation/perfusion mismatch) | CHD with heart failure or right-to-left shunt, operated Fallot, PAH, potential transplant recipients |
Pulmonary function tests | ↓ if co-existent pulmonary problems | CHD with multiple thoracotomies, right-to-left shunt or ventilation/perfusion mismatch |
Maximal workload (in watts) can easily be determined on a bicycle, but for a treadmill test it may be only estimated from the maximum speed and incline. It is important to use the same protocol to evaluate the trend in achievement of a given patient because the maximal power depends on the exercise protocol chosen, as long stages lead to a muscle fatigue at lower workloads compared with a protocol with shorter stages. Maximal power increases with age and, after puberty, the increase is greater in males than in females. Maximal power is commonly around 3.0–3.5 W/kg in healthy boys and 2.5–3.5 W/kg in healthy girls.
A 12-lead ECG should be used during exercise testing. The limb electrodes must be placed supraclavicularly, near the shoulders, and suprailiac. Resting heart rate and heart rate at a given workload decrease with growth, whereas peak heart rate is independent of age and sex in children, but depends on the mode of exercise, testing on a treadmill leading to higher maximal heart rates than on a bicycle. An increase in heart rate during exercise is delayed in athletes, but is more rapid than normal in deconditioned children or in those with heart failure. The decline in heart rate during recovery is much faster in the youngest children . Heart rate response in cardiac patients must be interpreted very carefully because many have chronotropic incompetence . An ECG can also detect exercise-induced arrhythmias and ischemic or QTc changes.
The oxygen pulse, which is the VO 2 per heartbeat, represents the product of stroke volume and arteriovenous oxygen extraction. This last variable changes predictably during exercise because, at peak exercise, oxygen extraction is maximized and mixed venous oxygen saturation varies little across a wide spectrum of cardiac function, so that oxygen pulse can be used as a surrogate marker for stroke volume. As it is difficult to measure cardiac output accurately by non-invasive methods in children, oxygen pulse is often used to assess ventricular function during exercise in children. Interpretation is difficult, however, when oxygen extraction is abnormal (e.g. in case of anaemia, polycythaemia, hypoxemia and metabolic or muscular disorders). Stroke volume has a higher relative contribution to cardiac output during the initial stages of exercise and the O 2 pulse kinetics profile is hyperbolic. A reduced rise in O 2 pulse is noted during exercise when stroke volume adaptation is limited (e.g. in case of ventricular dysfunction, obstructive cardiac or vascular disease or valvular regurgitation). The oxygen pulse is also decreased in Fontan and total cavopulmonary connection patients , probably because of the limited ability of the passively perfused pulmonary vascular bed to accommodate the high rate of blood flow during severe exercise. By comparison, oxygen pulse is usually normal at peak exercise in case of chronic aortic regurgitation because the fall in systemic vascular resistance during exercise lessens the severity of the regurgitation, and the dilated left ventricle helps to maintain forward stroke volume. Other ratios have been proposed, such as the VO 2 efficiency slope , which analyses the relationship of VO 2 versus the logarithm of VE and indicates the status of systemic and pulmonary perfusion, or work efficiency, which analyses the relationship of VO 2 versus work rate or, during graded treadmill exercise, versus estimated exercise intensity .
Oxygen saturation is measured by pulse oximetry using a finger or forehead sensor. Oximeters may have difficulty tracking the signal at peak exercise because they are motion sensitive. Exercise-induced arterial desaturation usually occurs in patients with pulmonary diseases or relevant intrapulmonary or intracardiac shunts.
Blood pressure measurement is essential if patients with cardiac diseases or suspected exercise-induced cardiovascular symptoms are tested. During exercise, systolic blood pressure increases whereas diastolic blood pressure remains relatively constant . A lack of increase or even a decrease in blood pressure may be related to cardiac dysfunction, although a drop can also occur in healthy children . An increase in diastolic blood pressure during exercise may indicate hypertension.
The ventilatory anaerobic threshold (VAT) is an indirect indicator of the onset of metabolic acidosis during graded exercise; it is usually defined as the VO 2 immediately below the exercise intensity at which pulmonary ventilation increases disproportionally relative to VO 2 . VAT is determined by a sudden increase in the ventilatory equivalent for O 2 (VE/VO 2 ) without an increase in the ventilatory equivalent for CO 2 (VE/VCO 2 ), a sudden change in the slope of the regression line between VO 2 and VCO 2 (V-slope method) or by changes in the respiratory exchange ratio. In any case, it is recommended to use 10–15-second data averages instead of breath-by-breath data, and to plot gas exchange data over VO 2 rather than over time . VAT is expressed as a percentage of peak VO 2 , decreases with age , suggesting an increase in lactic acid anaerobic capacity during growth, and is lower than normal in children with cardiac disease . VAT cannot be determined in all individuals from a single exercise test, and analysis of the same data set may reveal some interobserver variability. The anaerobic threshold is determined from a series of blood lactate measurements during an incremental exercise test. The work rate at which the lactate-over-work rate curve steepens considerably defines the anaerobic threshold. However, it is rarely performed in children because it usually does not add relevant information and because blood samplings are not well tolerated by young patients.
Ventilatory equivalents are often used to detect ventilatory inefficiency. The ventilatory equivalent for oxygen (VE/VO 2 ) and the ventilatory slope (VE/VCO 2 ) have a higher slope in cardiac patients. Pulmonary blood flow maldistribution and consequent ventilation/perfusion mismatch are probably the main factors that underlie this elevation; they result from pulmonary vascular obstructive disease in pulmonary hypertension, residual peripheral pulmonary stenoses in operated tetralogy of Fallot and elevated pulmonary capillary wedge pressure in heart failure. The ventilatory slope is also elevated in case of right-to-left shunt because the respiratory drive is increased by the CO 2 -rich systemic venous blood entering the systemic arterial circulation.
Many cardiac patients also have co-existent pulmonary problems, which can contribute to exercise intolerance; they tend to have mild-to-moderate restrictive changes resulting in smaller lung volumes and flow rates, leading to a ± 25% reduction in ventilatory capacity on average . A restrictive lung disease due to intrathoracic fibrosis or restriction of chest wall motion must be especially suspected in patients who have had multiple thoracotomies. Therefore, in cardiac patients with exercise intolerance, pulmonary function must be assessed by baseline spirometry, measurement of maximal voluntary ventilation (forced expiratory volume in 1 second multiplied by 40) and breathing reserve (percentage of maximal voluntary ventilation that is not used at peak exercise and is normally ± 30%), and exercise tidal flow-volume loops . The analysis of exercise end-tidal PCO 2 is also useful; lower values will be observed in case of ventilation/perfusion mismatch (the air from alveoli with high ventilation/perfusion ratio dilutes air from other alveoli) and right-to-left shunt (PCO 2 of the blood returning from the lung is reduced to compensate for the shunted hypercapnic blood to systemic circulation), whereas higher values will be noted in case of airway obstruction or hypoventilation due to obesity. When exercise-induced bronchospasm is suspected in patients with chest pain, exercise testing with pre- and postexercise pulmonary function before and after giving an inhaled beta-agonist, can be useful.
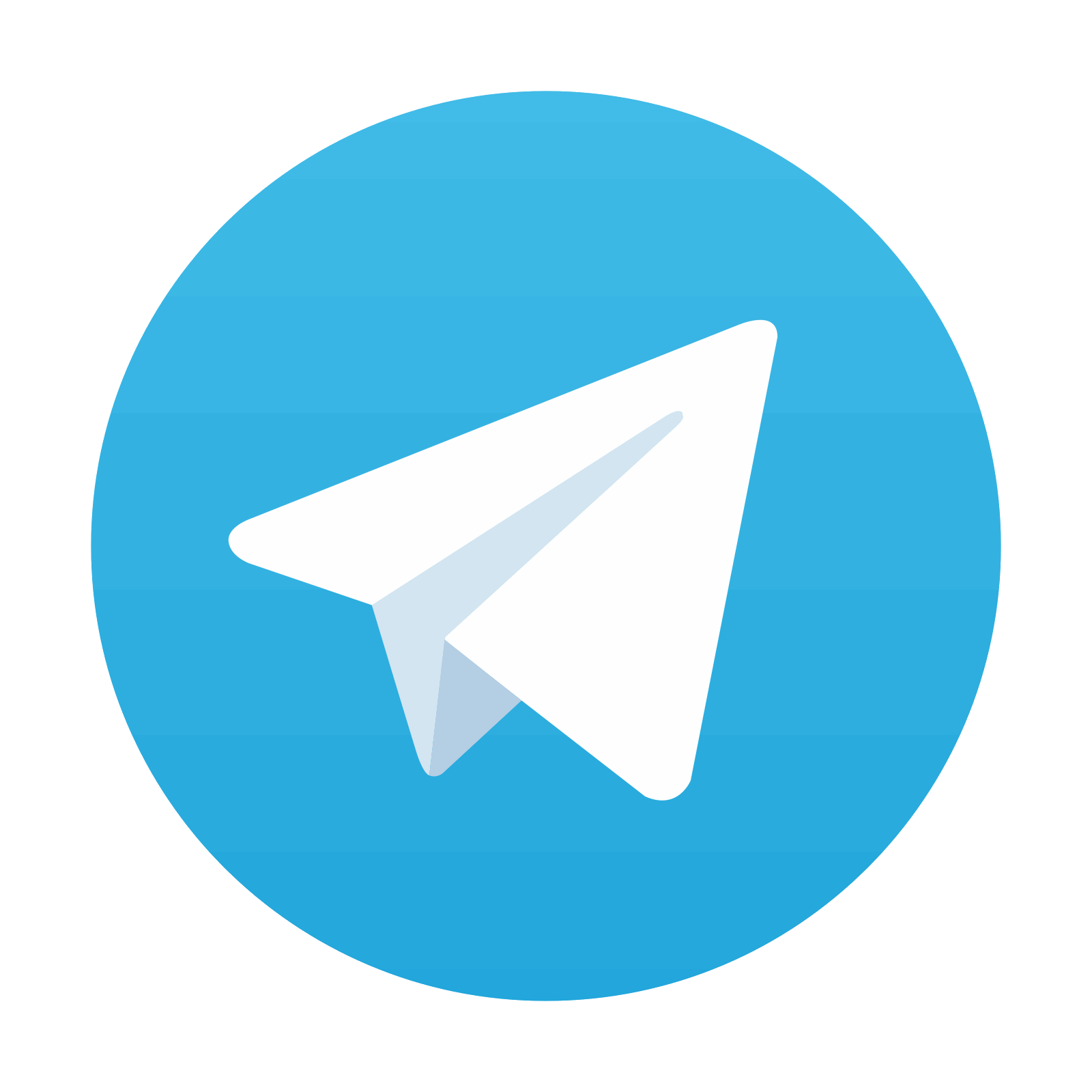
Stay updated, free articles. Join our Telegram channel

Full access? Get Clinical Tree
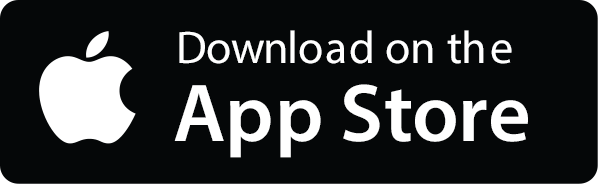
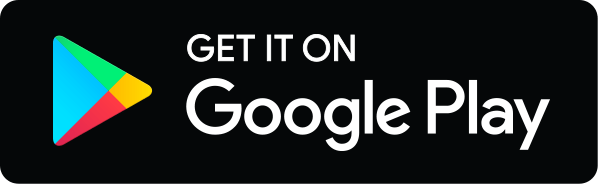