Fig. 17.1
Kaplan–Meier plots for all-cause mortality (a) and HF hospitalization (b) by RV ejection fraction categories [11]. (with permission Meyer P, Filippatos GS, Ahmed MI, et al. Effects of right ventricular ejection fraction on outcomes in chronic systolic heart failure. Circulation. 2010;121:252–258.)
Early Studies Demonstrating the Importance of RV Function in Left Heart Failure
Left ventricular failure or left-sided overload of systolic or diastolic nature represents the most common cause of RV dysfunction resulting from the passive elevation of pulmonary pressures. The mechanism of hemodynamic compromise results from an increased RV afterload and subsequent decreased stroke volume. However, in contrast to the primary pressure overload of the RV, this situation is accompanied by systolic dysfunction and chamber enlargement of the LV and, therefore, there is no dramatic evidence of underfilling of the LV via the mechanism of leftward shift of the interventricular septum as in the setting of an acute pulmonary embolus or RV myocardial infarction. Nonetheless, ventricular interactions may explain the improvement in hemodynamics after treatment with drugs having a beneficial effect on RV afterload in patients with severe CHF, since there is a well-documented interaction of RV and LV systolic contraction [20–22]. The decision to use univentricular versus biventricular support is one of the key problems in the successful application of mechanical circulatory support for the treatment of patients with heart failure. Figure 17.2 demonstrates a four chamber apical echocardiographic view of a patient with heart failure before and after insertion of a LV assist device demonstrating the increase in RV dimensions and decrease in LV dimensions due to the unloading effect of the LV assist device [22]. The mechanisms of diastolic and systolic ventricular interaction weigh heavily in this decision and it would appear that the decision will depend upon the pulmonary vascular resistance and the status of RV function in each individual case.


Fig. 17.2
Four chamber views of a patient with heart failure before and after insertion of LV assist device demonstrating the increase in RV dimensions and decrease in LV dimensions due to the unloading effect of the LV assist device [22]. (with permission Dell’Italia LJ. Anatomy and physiology of the right ventricle. Cardiol Clin 2012;30:167–187.)
The physiologic effects on RV function may have important consequences because of the very compelling evidence that RV function is an important determinant of the hemodynamic response, and the prognosis and exercise capacity in patients with CHF. As early as 1983, Polak and coworkers emphasized the importance of RV function in the prognosis of 34 patients with LV failure secondary to atherosclerotic coronary disease [6]. Only 16 patients were alive after a 2-year follow-up and these patients had a higher RV ejection fraction and less LV dysfunction. When a RV ejection fraction of less than 35 % was used as a cut off, mortality was significantly greater among the 21 patients with depressed RV ejection (71 vs. 23 %). Although patients with depressed RV ejection fraction had a greater prevalence of chronic obstructive lung disease and previous inferior myocardial infarction, the differences between the groups were not statistically significant. These underlying problems must be taken into consideration because they are a cause of RV dysfunction independent of left heart failure. Nevertheless, RV ejection fraction has also been identified as an important predictor of outcome after mitral valve surgery in patients with isolated mitral regurgitation in the absence of coronary artery disease [23–28].
Hochreiter and coworkers studied 53 patients with chronic severe mitral regurgitation using right heart catheterization, radionuclide angiography, exercise testing, and ambulatory electrocardiography [23]. Stepwise multiple logistic regression analysis demonstrated only resting RV ejection fraction and left atrial size to be independently associated with exercise capacity, and RV ejection fraction was inversely proportional to the pulmonary arterial systolic pressure (r = −0.67, p < 0.001) and pulmonary capillary wedge pressure (r = −0.68, p < 0.001). Of interest, RV ejection fraction was the only variable significantly related to the presence or absence of complex arrhythmias. Finally, all deaths among medically treated patients are clustered among those with RV ejection fraction <30 % (p < 0.0001 vs. patients with RV ejection fraction >30 %) and LV ejection fraction <45 %. Subsequent studies in small numbers of patients have demonstrated that the failure of the RV ejection fraction to rise despite absence of symptoms and well-preserved LV ejection fraction indicates an almost 50 % likelihood of HF during the succeeding 3 years, which is fourfold greater than for patients with preserved exercise RVEF [23–26]. Most recently in 2012 and 2013, the results from a follow-up of large numbers of patients demonstrate the prognostic importance of the pulmonary systolic pressure [27, 28] or RV ejection fraction measured by radionuclide angiography (Fig. 17.3) [29] in patients with degenerative isolated mitral regurgitation. Figure 17.3 demonstrates the dramatic decrease in 10-year cardiovascular survival in patients with RV and LV ejection fraction impairment [29]. These studies demonstrate the critical importance of RV performance in determining the functional severity of mitral regurgitation and in predicting its important effect on survival that is most likely related to the severity of chronic afterload excess. Indeed, an estimated pulmonary systolic pressure of 50 mmHg is an indication for consideration for mitral valve surgery in the absence of symptoms and a LV ejection fraction >60 % [30, 31].


Fig. 17.3
(a) Ten-year cardiovascular survival rate after mitral valve surgery in patients with preserved (>35 %) versus depressed (≤35 %) RV EF (p = 0.037). (b) Ten-year cardiovascular survival rate after mitral valve surgery in patients with various combinations of RV and LV ejection fraction measured by radionuclide angiography. Importantly, there is a dramatic decrease in 10-year cardiovascular survival in patients with RV and LV ejection fraction impairment compared to the remaining patients (p < 0.0001) [29]. (with permission Le Tourneau T, Deswarte G, Lamblin N, Foucher-Hossein C, Fayad G, Richardson M, Polge AS, Vannesson C, Topilsky Y, Juthier F, Trochu JN, Enriquez-Sarano M, Bauters C. Right Ventricular Systolic Function in Organic Mitral Regurgitation: Impact of Biventricular Impairment. Circulation. 2013;127(15):1597–608.)
Also starting in the early 1980s, several studies demonstrated that the exercise capacity of heart failure patients is also dependent on RV function. Baker and coworkers studied 25 patients with chronic LV failure due to coronary artery disease (n = 12) and idiopathic dilated cardiomyopathy (n = 13) [32]. Maximum upright bicycle exercise testing was performed to determine maximum oxygen consumption (MVO2) which averaged only 13 + 4 mL/min/kg in this group of patients. Resting LV ejection fraction did not correlate with MVO2 (r = 0.08). However, resting RV ejection fraction did correlate with MVO2 (r = 0.70, p < 0.001) in the 25 patients, even when these patients were separated into ischemic (r = 0.88, p < 0.001) and nonischemic (r = 0.60, p < 0.01) causes of cardiomyopathy. Leier and coworkers demonstrated significantly improved clinical status and exercise capacity during treatment with isosorbide dinitrate in 30 patients with moderate to severe CHF [33]. This improvement in exercise capacity may have been attributed to afterload reduction of the RV resulting in an augmented RV stroke output and improved LV filling; in addition, Franciosa and Baker demonstrated that indices of pulmonary vascular resistance afterload are better indicators of exercise capacity than previously studied markers of LV systolic dysfunction [34]. In 41 patients with chronic LV failure, exercise MVO2 correlated with the resting pulmonary artery wedge pressure (r = −0.54, y = 32.8−0.8x, p < 0.001) and mean pulmonary artery pressure (r = −0.54, y = 44.9−1.0x, p < 0.001) but not systemic vascular resistance (r = −0.20). It is therefore tempting to speculate that in the pre-captopril era of heart failure therapy, improved survival in the VA Cooperative Study achieved with combined hydralazine and nitrate therapy may have been to a large part due to a drug related decrease of the pulmonary vascular resistance [35].
To determine the relative efficacy of right versus left-sided afterload reduction on the hemodynamic response to nitroprusside infusion, Yin and coworkers measured both pulmonary and systemic vascular impedance in seven patients with severe CHF [36]. Left ventricular unloading was manifested by a fall in systemic vascular resistance, whereas significant unloading of the RV was manifested by a similar decrease in pulmonary vascular resistance but also by a direct decrease in pulmonary artery compliance by simultaneously measuring pressure and flow in the main pulmonary artery. Thus measuring pulmonary vascular impedance (see also Chap. 2) reveals hemodynamic alterations in the compliance of the central pulmonary vessels that are not reflected in the standard pulmonary vascular resistance and suggest a direct, active effect of nitroprusside on the pulmonary vasculature rather than a passive decrease in pulmonary venous pressures. These data suggest that both LV hemodynamics and cardiac output benefit in an additive fashion from a primary afterload reduction of the RV during nitroprusside infusion. The value of pulmonary vascular impedance measurement in the determination of RV afterload will be further discussed in this chapter.
In 1985 Packer and coworkers compared the effects of captopril and isosorbide dinitrate, drugs with known effects on pulmonary arteriolar resistance, in patients with severe left ventricular failure [37]. Left ventricular filling pressures declined similarly with both captopril and isosorbide dinitrate; however, the mean right atrial pressure decreased to a greater extent with nitrates than with captopril. Although systemic resistance declined similarly with both drugs, the cardiac index increased to a greater extent with nitrate therapy than during converting-enzyme inhibition (+0.47 vs. +0.23 L/min/m2, p < 0.01). The authors speculated that an inappropriately high pulmonary afterload limits the degree to which the RV output can increase during converting-enzyme inhibition; this may explain why patients with poor RV function not respond to converting-enzyme inhibitors. Furthermore, preliminary data from early work by Packer and coworkers suggested that RV function appears to be the principle determinant of long-term survival in patients treated with captopril [38]. In summary, these and other early studies [39–42] demonstrate the importance of both pulmonary and systemic arterial afterload reduction not only in the treatment of patients with CHF but also in patients with mitral valvular heart disease.
Most recently, it was reported that in patients presenting with acute decompensated left heart failure, improvement in RV mechanics in response to intensive medical therapy was associated with a lower rate of long-term adverse events when compared to patients not showing improvement of RV function [43]. It is important to note that in this study the presence of tricuspid regurgitation was significantly worse in patients with adverse events. This study utilized two-dimensional echocardiographic strain imaging to quantify regional myocardial function. This fairly simple technique can provide a more direct assessment of RV mechanics and RV strain imaging (see also Chap. 10). These results suggest that medical therapy targeted to improve RV function in heart failure will improve functional capacity and outcomes. At this point it is important to address the assessment of RV function, which will be more completely addressed in Chaps. 2, 11, and 12.
The RV forms a crescent-shaped structure with a very flat main chamber having a heavily trabeculated but thin free wall. It is bounded by the thicker interventricular septum medially and the cylindrical outflow tract superiorly, and in contrast to the ellipsoidal LV, the RV chamber defies measurements based on an appropriate geometric model. To further complicate matters, the ejection of blood from the RV chamber emanates from an interaction of three different sources: free wall, interventricular septum, and conus. The conus, with its circumferentially arranged muscle fibers [44], contracts 30–50 ms after the base and apex of the free wall; [45–47] this can be exaggerated by vagal stimulation or abolished by sympathetic stimulation [48, 49]. The free wall contracts in a peristalsis wave-like fashion starting at the base and progressing to the apex (see also Chap. 11). In the normal RV, because of the highly compliant lung circulation, RV free wall and outflow tract segments cease shortening, and even lengthen, as the ejection continues and RV pressure rapidly fall during relaxation [50]. Thus, it has been proposed that RV systolic ejection dynamics are dependent on early active shortening of the free wall surface area and septal to free wall distance and later, when the column of blood located in the outflow tract continues to flow into the pulmonary artery, due to the momentum of the expelled blood and the normally highly compliant pulmonary vascular bed [51]. Figure 17.4 demonstrates these important features of RV contraction using magnetic resonance imaging in a patient with a previous left anterior descending coronary artery occlusion with LV apical thinning but well preserved RV systolic function. As described above, there is a very impressive RV free wall shortening with marked translation of the tricuspid annulus and decrease in RV area from end-diastole to end-systole, which is evident in the normal hearts, in hearts of marathon runners, and in patients with well-preserved RV and LV function with isolated mitral regurgitation (Fig. 17.5). However, this RV free wall thickening and tricuspid annular excursion is markedly reduced in a diffuse ischemic cardiomyopathy (Fig. 17.6). These anatomic and functional features of RV ejection dynamics demonstrate the utility of the echo/Doppler equivalents of RV function measured by RV free wall area, tricuspid annular plane systolic excursion, and RV free wall strain imaging which have been used in large scale studies to determine the prognostic importance of RV function in left heart failure.




Fig. 17.4
Magnetic resonance imaging in a patient with a previous left anterior descending artery occlusion with LV apical thinning but well preserve RV systolic function. There is very impressive RV free wall thickening with marked translation of the tricuspid annulus that is greater than that of the LV

Fig. 17.5
End diastolic imaged from the short axis and end-diastolic and end-systolic images from four chamber below of normal, marathon runner, and mitral regurgitation subjects demonstrating the differences in LV and RV remodeling in the marathon runner and mitral regurgitation subjects. Note the elongation of the heart in the marathon runner and the sphericalization of the LV in the mitral regurgitation LV. The RV function is well preserved with increased end-diastolic volume in the marathon runner and RV free wall thickening and marked translation of the tricuspid annular plane [159]. (with permission Schiros CG, Ahmed MI, Sanagala T, et al. Ventricular structural remodeling in endurance athletes compared to patients with mitral regurgitation using magnetic resonance imaging with three-dimensional analysis. Am J Cardiol. 2013;111(7):1067–1072.)

Fig. 17.6
Ischemic cardiomyopathy demonstrating inferior wall thinning and akinesis in the two chamber image (far left). In the four chamber image (middle) there is lateral wall akinesis with RV dilatation and markedly reduced RV free wall shortening and minimal tricuspid annular movement from end-diastole to end-systole. In the short axis images there is RV dilatation with reduced free wall thickening and severe LV systolic dysfunction. The LV inferior wall akinesis is also present in the inferior portion of the interventricular septum
Even with the most advanced imaging techniques, the complex anatomy of the RV still poses challenges that are not encountered when imaging the more symmetrical LV. It must be emphasized that multiple techniques have been utilized to assess the impact of RV function on prognosis in left heart failure including echocardiographic RV fractional area [19], tricuspid annular displacement [9, 17], and RV free wall strain [43] and radionuclide RV ejection fraction [5, 11]. The nuclear imaging technique is the method that has been widely utilized in many of the published studies demonstrating the importance of RV function in left heart failure [5, 11]. The advantage of radionuclide angiographic techniques used to measure RV ejection fraction is its geometry independence, but the weakness of this technique is the right atrial overlap. However, it is important to appreciate the differences in RV ejection fraction that can occur with various radionuclide imaging techniques. Figure 17.7 demonstrates the inherent problems associated with the gated equilibrium and the first pass techniques with regard to the problem of anatomical right atrial overlap with the RV chamber in the right anterior oblique and left anterior oblique projections (Fig. 17.7) [52]. Marving and coworkers compared RV ejection fractions calculated from three approaches: (1) gated first pass fixed region of interest, (2) gated first pass variable region of interest, and (3) gated equilibrium method [52]. Methods 1 and 3 had a tendency to underestimate the RV ejection fraction, probably resulting from inclusion of right atrial activity into the RV region of interest at end-systole. However, LV ejection fraction is unaltered by any of the techniques. Using cine-magnetic resonance imaging as the gold standard for RV ejection fraction, we showed that a first pass 2-region of interest method or the first pass right atrial subtracted single region of interest method are the most accurate methods for assessing RV ejection fraction [53].


Fig. 17.7
First pass end-diastolic (a) and end-systolic (b) frames and gated equilibrium radionuclide angiographic images from end-diastole to end-systole (1–8) demonstrating the problem of right atrial overlap with the RV chamber in each of the techniques. The fixed region technique with first pass (white dots) and overlap with the equilibrium technique (arrows) demonstrate the contribution of right atrial counts to end-systolic RV counts [52]. (with permission Marving J, Hoilund-Carlsen PF, Chræmmer-jorgensen B, Gadsboll N. Are right and left ventricular ejection fractions equal? Ejection fractions in normal subjects and in patients with first acute myocardial infarction. Circulation. 1985;72(3):502–514.)
Right Ventricular Anatomy and Physiology
The reason for the important predictive capacity of RV function in the setting of left heart failure may be obvious because the failure of both ventricles not surprisingly results in a greater overall loss of function and decreased survival. However, at this point it is important to review the great capability of the RV to maintain function in the setting of ischemic heart disease. Protection from ischemic damage may also explain the importance of the RV in predicting not only morbidity and mortality, but also exercise capacity and response to biventricular pacing in LV systolic heart failure.
A description of the anatomy of the RV emphasizes the importance of the conus in support of RV function. The muscle mass of the low pressure RV is approximately one-sixth that of the high pressure LV. The thin-walled RV forms a crescent-shaped chamber comprised of a sinus (body) and outflow tract which stand in stark contrast to the ellipsoidal, concentric left ventricle. Both ventricles are bound together by spiraling muscle bundles encircling both ventricles in a complex interlacing fashion forming a functionally single unit. Of particular interest is the RV conus or outflow tract, because of its mechanical advantage of circumferentially organized fibers and its protection from ischemic injury due to the unique anatomy of the right coronary circulation.
Already in 1924 the RV conus was recognized as being anatomically distinct from the main portion of the RV [54]. In all developing vertebrate embryos, the bulbus cordis is present as a separate chamber distal to the common ventricle but disappears in the LV as development proceeds, whereas in the RV it remains to form the infundibulum or outflow tract (see also Chap. 1). The muscle fibers of the conus run in a parallel alignment from epicardium to endocardium in nine different mammalian species including man; while muscle fibers of the sinus or body of the RV undergo a slow right angular directional change from epicardium to endocardium and this fiber orientation is similar to that of the LV [44]. Both sinus and conus have a similar wall thickness; however, the conus has a mechanical advantage over the sinus because it has a smaller radius of curvature. Armour and coworkers have also demonstrated that there exists an appreciable pressure drop from the sinus to the conus portion during stellate stimulation in the anesthetized dog and that the lower pressure in the conus section is transmitted to the pulmonary artery [44]. The ability of the conus to function as a resistive element preventing the high sinus pressure from reaching the pulmonary artery is easily appreciated given its anatomy. This cylindrical structure is composed of parallel circumoral fibers resulting in a much smaller radius of curvature and a greater wall thickness to radius when compared to the thin-walled sinus. Consequently, the conus region has a lower wall stress for any given pressure and is capably suited for absorbing and dissipating the high pressure generated by the sinus. Therefore, there exists an architectural and functional separation of the inflow and outflow tracts of the RV.
It is of interest that Schlesinger and coworkers reported a lower incidence of occlusion of the conus artery than any other artery directly connected to the aorta [55]. This feature, coupled with its location between the main left anterior descending and right coronary arteries, explains its functional importance in supplying collateral blood flow to these vessels with a much higher incidence of occlusion [56, 57]. In addition, the unique anatomic position of the conus artery arising close to the ostium or as a separate ostium artery generally results in preservation of systolic function of the RV outflow tract in patients with acute RV myocardial infarction, because ostial occlusions of the right coronary artery are an infrequent occurrence. In the setting of left heart failure it is tempting to speculate that the conus shortening is important for the preservation of RV systolic function independent of the body of the RV. In support of this hypothesis, RV outflow tract shortening by M-mode echocardiography from the parasternal short axis view was shown to accurately separate patients with normal and reduced RV function [58].
One of the more remarkable capacities of the RV is the well-documented recovery of RV ejection fraction after RV myocardial infarction [59–61]. This unique feature of the RV is even more impressive when taking into consideration that these studies were performed before the widespread use of coronary reperfusion with either thrombolytic therapy and percutaneous transluminal coronary angioplasty. In the pig, the RV has a lower mitochondrial density and ratio of mitochondria:myofibrils than that of the LV, which has a higher workload and greater myocardial oxygen consumption (MVO2) [62]. RV oxygen extraction is difficult to measure in vivo because the RV venous drainage is accomplished through a complex network of Thebesian veins and five to seven anterior cardiac veins [63]; this complicates the accurate assessment of the venous efflux from the RV. Nevertheless, cannulation of the anterior cardiac veins and the coronary sinus in open-chest dogs showed that RV myocardial oxygen consumption (MVO2) was approximately one-half that of the LV and right coronary artery blood flow was lower than coronary blood flow in the left anterior descending artery under baseline conditions [64]. Pacing, isoproterenol, and methoxamine stress, resulted in an increase in the left anterior descending artery flow with no increase in MVO2 in the LV; while in the RV these stresses resulted in an increase in both right coronary artery blood flow as well as oxygen extraction. Cardiac volume loading induced by creation of an arterio-venous shunt increased RV MVO2 and augmented oxygen extraction in addition to a rise in right coronary artery blood flow; while the increment in LV oxygen extraction was less than that in the RV (31 vs. 54 %, p < 0.01) [65]. Other studies also demonstrate that RV oxygen demand, blood flow rate and oxygen extraction are approximately one-half that of the LV [66, 67]. These properties, combined with a higher coronary vasomotor tone at baseline, explain the RV’s unique ability to increase oxygen extraction in response to afterload and inotropic stress. These properties, however, are abolished in dogs with RV hypertrophy caused by pulmonary artery banding (PAB) resulting in responses which were indistinguishable from those of the LV [68].
The RV with its thinner wall and lower operating pressures offers other interesting contrasts regarding coronary flow dynamics throughout the cardiac cycle, resulting in a systolic-diastolic flow ratio that is much greater in the coronary vessels that perfuse the RV when compared to those that perfuse the LV [69–75]. Normal phasic flow in the right and left coronary arteries has been extensively studied in the dog using electromagnetic flow probes. This is a unique preparation because the canine right coronary artery is a nondominant vessel which only supplies the RV free wall. Systolic coronary artery flow commences rapidly in both arteries after opening of the aortic valve producing an early peak which declines immediately to a higher plateau throughout systole in the right coronary artery compared to the left coronary artery. Subsequently, the level of flow in the right coronary artery during diastole remains relatively constant in contrast to the rapidly declining diastolic flow in the left coronary circulation. However, systolic flow was markedly reduced and was inversely related to peak RV systolic pressure in dogs with congenital pulmonic stenosis.
In summary, low oxygen demands, the ability to extract more oxygen during times of stress, and both systolic and diastolic coronary flow provide a greater reserve of nutrients and oxygen during ischemia. These mechanisms may work in concert to prevent irreversible ischemic damage to the RV in the setting of heart failure due to ischemic heart disease. However, RV necrosis was markedly increased in pigs after right coronary occlusion with a preexisting RV hypertrophy induced by PAB [76]. This inherent protection from ischemic RV damage may be offset in patients by any condition that causes RV hypertrophy due to chronic pulmonary hypertension or results in a greater extent of coronary artery disease. It is of interest that in a recent report, a reduction in RV ejection fraction to less than 40 % beyond the first 30 days after an acute myocardial infarct predicted a three times higher long-term mortality risk independent of other variables [77]. In another recent study cMRI evidence of RV infarction after primary percutaneous coronary intervention indicates a 16-fold higher risk of major adverse cardiac events and even more so in patients with an anterior myocardial infarct [78]. Thus, the presence of more extensive coronary artery disease may explain why there are some studies where RV function is not predictive of exercise capacity in heart failure patients [79, 80]. It is of further interest that in a large cohort of subjects in the Multi-Ethnic Study of Atherosclerosis (MESA) study, RV hypertrophy measured by cMRI was associated with the risk of heart failure or death in a multi-ethnic population free of clinical cardiovascular disease at baseline [81]. It is tempting to speculate that RV hypertrophy may be an early indicator of increased LV end-diastolic pressure resulting from LV systolic or diastolic dysfunction, which in turn increases RV pressure and wall stress. This study underscores the importance of RV morphology as well as RV ejection fraction in the complete assessment of the patient with heart failure.
There is mounting evidence for the importance of LV diastolic dysfunction in the development of pulmonary hypertension in patients with heart failure and preserved LV ejection fraction [12, 82, 83], which not surprisingly is exacerbated by the coexistence of mitral regurgitation [84]. Recent studies reinforce the concept that a combined noninvasive echo-derived assessment of RV systolic function using tricuspid annular plane systolic excursion and Doppler pulmonary systolic pressure is the most sensitive and predictive index for prognosis of both patients presenting with either reduced or preserved LV systolic function [85–87]. Figure 17.8 demonstrates that the tricuspid annular plane systolic excursion vs. pulmonary artery systolic pressure relationship shows a downshift of the regression line for non-survivors vs. survivors. Further, the tricuspid annular plane systolic excursion vs. pulmonary artery systolic pressure relationship quartiles distribution shows that this relationship is shifted downward in non-survivors with a similar distribution in heart failure patients with reduced or preserved LV systolic function (Fig. 17.8). Taken together, these recent studies emphasize that reduced RV function alone is not the crucial factor for the patient’s prognosis, but rather the coexistence with elevated pulmonary systolic pressure is crucial for prognosis and survival in left heart failure. This information now adds to the previous data demonstrating the independent prognostic importance of both pulmonary systolic pressure and RV systolic function in left heart failure and opens the question of how the low pressure RV pump responds to increasing afterload at the level of the myocardium.


Fig. 17.8
TAPSE vs. PASP relationship and shows a downshift of the regression line for non-survivors vs. survivors (a). Non-survivors exhibited a more unfavorable relationship having higher PASP and lower TAPSE values, respectively. HFrEF and HFpEF (b) were significantly different (p = 0.009) despite a similar distribution across the respective TAPSE and PASP. When patients were separated according to TAPSE </≥16 mm, a downward shift occurred for TAPSE <16 mm 184 group with slope of the regression significantly different (p = 0.048) (c). No correlation was found between TAPSE and LV ejection fraction [87]. (with permission Guazzi M, Bandera F, Pelissero G, Castelvecchio S, Menicanti L, Ghio S, Temporelli P, Arena R. Tricuspid Annular Systolic Excursion and Pulmonary Systolic Pressure Relationship in Heart Failure: an Index of Right Ventricular Contractility and Prognosis. Am J Physiol. 2013 Aug 30.)
Characteristics of RV as a Pump
The thinner RV free wall and conus comprise a markedly different chamber geometry that has one-sixth the mass and operates at volumes slightly greater than the LV. At the chamber level, pressure–volume relationships from isolated hearts demonstrate that the RV has greater chamber distensibility than the LV (see also Chap. 2) [88, 89]. Since the right and left ventricles are comprised of the same interlacing muscle fibers that encircle the heart, the greater distensibility reflects a necessary functional difference of two pumps in series having equal stroke outputs but coupled to markedly different vascular loads. Diastolic ventricular interaction is present on a moment to moment and beat to beat basis especially during respiration; however, ventricular interaction is most apparent with acute changes in ventricular volume, in particular, in the setting of an acute pulmonary embolus and after implantation of an LV assist device, as demonstrated in Fig. 17.2 (see also Chap. 9).
The force opposing the shortening of muscle fibers, or afterload, is a major determinant of myocardial performance in isolated muscle preparations and in the intact heart. Pulmonary Impedance is a measure of the opposition to pulmonary artery flow. Pulmonary input impedance measurements in normal animals [90–93] and man [94–97] have consistently demonstrated a pulse wave velocity that was approximately one-half that of the normal systemic circulation. This causes reflected pressure waves to return later, after the pulmonic valve closure, and appears to offset the shorter distances to reflecting sites in the pulmonary arteries so that the right and left ventricles are optimally matched at physiologic heart rates. Figure 17.9 demonstrates simultaneous tracings of the electrocardiogram, the first derivative of RV pressure development (dP/dt), pulmonary artery flow velocity, and high-fidelity right ventricular and pulmonary artery pressures in a normal subject [98]. The normal RV pressure wave form has a low peak pressure which occurs early in systole and subsequently drops off rapidly. The pre-ejection period (PEP) and RV ejection time (RVET) aid in demonstrating minimal isovolumic contraction due to the low pulmonary artery diastolic pressure and ejection of blood as the RV pressure is rapidly declining. The observation of continued forward flow in the presence of, not only a declining pressure, but also a negative pressure gradient, emphasizes the inability of pressure measurements alone to define the mechanisms of RV-pulmonary artery coupling because total RV afterload is comprised of resistive, capacitative, inertial, and pulse wave reflection properties of the pulmonary vasculature (see also Chap. 2). In contrast, Fig. 17.10 demonstrates the response to acute pressure loading with phenylephrine in humans without cardiovascular disease; RV pressure and flow dynamics generate a longer period of isovolumic pressure which in part accounts for a higher dP/dt max. In addition, the earlier reflection of pressure waves produces a more rounded RV pressure wave form with cessation of forward flow soon after the peak pressure. Indeed, chronic [99, 100] RV hypertension in humans demonstrates pressure and flow characteristics similar to those of the LV and aorta. In normal subjects and in patients with chronic pulmonary hypertension, there is a linear relationship between pulmonary artery pressure and peak + dP/dt max [99]. Furthermore, high + RV dP/dt max values have been noted in patients with pulmonary hypertension despite clinical evidence of RV failure manifested by markedly elevated right heart filling pressures, ascites, and peripheral edema [100]. Therefore, values in the normal RV are low (100–250 mmHg/s) and do not provide a reliable index of contractility because of the load dependency of peak + dP/dt max.



Fig. 17.9
Simultaneously recorded electrocardiogram (ECG), RV analog signal of pressure development (dP/dt), phasic pulmonary artery flow, pulmonary artery pressure (PAP), and RV pressure (RVP) in a human. Vertical lines demonstrate the PEP and RVET. The hangout interval (HOI) represents the time interval between the pulmonary incisura and the RV pressure wave form, due to ejection into the very compliant pulmonary vasculature (see also Chap. 2) [22]. (with permission Dell’Italia LJ. Anatomy and physiology of the right ventricle. Cardiol Clin 2012;30:167–187.)

Fig. 17.10
Simultaneous electrocardiogram (ECG), RV dP/dt, phasic pulmonary artery (PA) flow velocity, and PA and RV pressure at low, medium, and high loading conditions produced by nitroprusside and phenylephrine infusion. The increase in RV pressure is accompanied by a higher dP/dt, greater wave reflection, and increased isovolumic contraction time [22]. (with permission Dell’Italia LJ. Anatomy and physiology of the right ventricle. Cardiol Clin 2012;30:167–187.)
As demonstrated above, the continuation of forward blood flow during a phase of a rapidly declining pressure characterizes RV ejection dynamics in the presence of a normal pulmonary circulation. The role of RV unloading in CHF remains largely unexplored, despite the recognition that RV performance has both great functional and prognostic importance in heart failure. As mentioned, early studies demonstrated that the cardiac index increased to a greater extent with nitrate therapy than during converting-enzyme inhibition, raising the question whether renin-angiotensin system blockade has any direct effect on pulmonary vascular resistance other than its unloading of the LV [37]. In contrast nitroprusside produced a balanced unloading effect on the systemic circulation and the RV, manifested by a decrease in pulmonary vascular resistance and a decrease in pulmonary artery compliance in heart failure patients [36]. In addition, the amplitude of impedance oscillations was less at higher nitrate doses, suggesting less wave reflection [101]. A recent study of dogs with pacing-induced heart failure produced a profound increase of the RV afterload (measured by pulmonary impedance) and RV systolic dysfunction that was out of proportion to the estimated pulmonary vascular resistance or pulmonary artery pressure [102]. This study underscores the potential effects of a subclinical afterload on the RV that may not be apparent by standard hemodynamic monitoring of pressures.
RV Diastolic Function in Heart Failure
All of the foregoing discussion supports the notion that the prognosis in heart failure patients is heavily dependent on the adaptation of RV function to an increased afterload. There are now numerous reports from clinical registries documenting the importance of an increased right atrial pressure/pulmonary capillary wedge pressure ratio for predicting an increased risk of adverse outcomes in patients with advanced heart failure [103–105]. Some of the studies demonstrate further associations between higher pulmonary vascular resistance and reduced RV function manifested by an enlarged right atrium and ventricle and a lower RV stroke work index [105]. In the more extreme example of RV dysfunction in acute RV myocardial infarction, an increase of the right atrial pressure/pulmonary capillary wedge pressure ratio >0.8 is coincident with low cardiac output and RV systolic and diastolic dysfunction (see also Chap. 9) [60, 106–109]. In a systematic and prospective study of 53 patients with inferior myocardial infarction, the findings of both an elevated jugular venous pressure (>8 cm H2O) and Kussmaul’s sign on physical examination were the most sensitive (88 %) and specific (100 %) markers for hemodynamically important RVMI by invasive hemodynamic recordings [110]. Taken together, a simple physical examination that demonstrates an unequivocally normal or only slightly elevated jugular venous pressure should suggest well-preserved RV systolic function and a better prognosis in patients with CHF. Indeed, of the 7,788 participants in the Digitalis Investigation Group trial, 1,020 (13 %) had an elevated JVP at baseline and in these patients the JVP was a marker of a higher burden of sickness and poor outcomes [111].
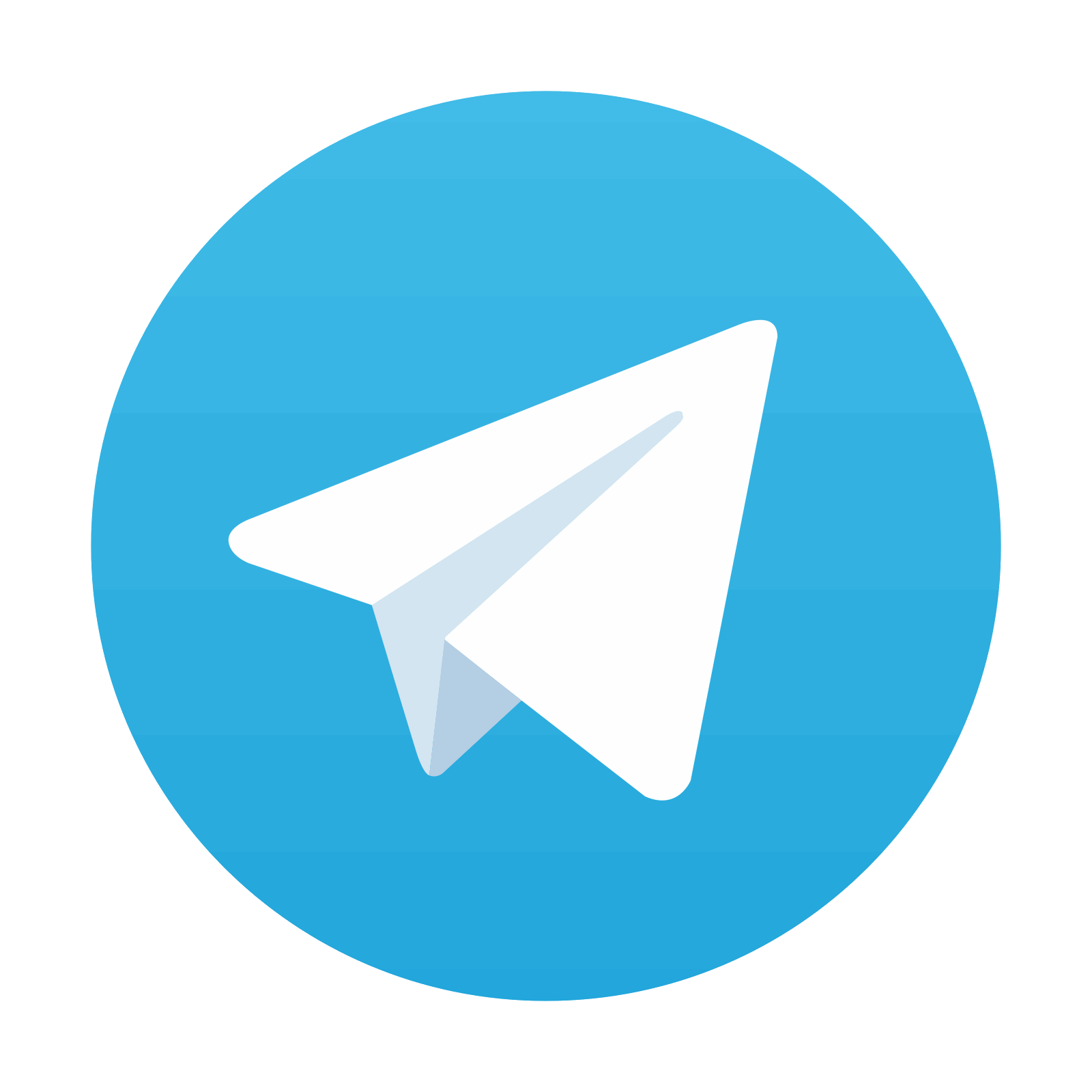
Stay updated, free articles. Join our Telegram channel

Full access? Get Clinical Tree
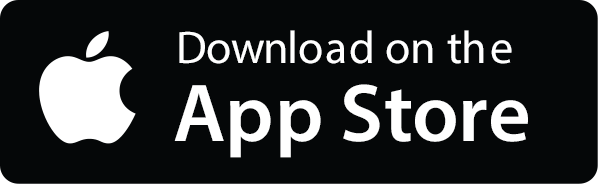
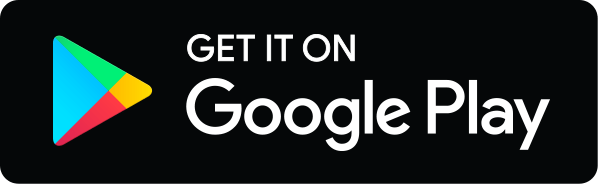