Fig. 4.1
Scheme of the RAAS and its inhibitors. Enzymes are in green, inhibitors are in red. Abbreviations: ACE angiotensin converting enzyme, ACEi ACE inhibitors, Dri direct renin inhibitors, ARB angiotensin receptor blockers, Aldo aldosterone, AS aldosterone synthase, ASi AS inhibitors, MRA mineralocorticoid receptor antagonists, ARNi angiotensin receptor-neprilysin inhibitors. Values in brackets are mean concentrations in nMol/L
4.1.2 Angiotensin II
4.1.2.1 History (Table 4.1)
Table 4.1
Chronology of discoveries and clinical observations in the RAAS
Authors/year | Discovery |
---|---|
Tigerstedt and Bergman 1898 [11] | Renal extracts contain a pressor substance |
Goldblatt et al. 1934 [12] | Renal artery constriction produces hypertension in dogs |
Renin is a peptidase producing angiotonin/hypertensin | |
Kahn et al. 1952 [15] | Increased “hypertensin” activity in blood of hypertensive humans |
Simpson et al. 1953 [16] | Discovery of aldosterone |
Skeggs et al. 1954 [17] | Identification of Ang I and Ang II |
Skeggs et al. 1956 [18] | Identification of ACE |
Liddle 1957 [19] | SC-5233 increased urinary excretion of Na in a patient with HF |
Gross 1958 [20] | Ang II releases aldosterone |
Kagawa et al. 1959 [21] | Isolation of spironolactone at Searle laboratories |
Pals et al. 1971 [22] | Saralasin, the 1st Ang II peptide antagonist |
Brunner et al. 1972 [23] | High renin levels associated with heart attack and stroke |
Cushman et al. 1977 [24] | Synthesis of the 1st ACE inhibitor, captopril |
Carini and Duncia 1988 [25] | Synthesis of 1st orally active nonpeptide ARB, losartan |
Brilla et al. 1993 [26] | Cardiac fibrosis by aldosterone-salt, prevented by spironolactone |
Pitt et al. 1997 [27] | ELITE I study, captopril: decreased mortality in HF |
Pitt et al. 1999 [28] | RALES study, spironolactone decreases mortality in HF |
Pitt et al. 2000 [29] | ELITE II study, losartan = captopril to decrease mortality in HF |
Pitt et al. 2003 [30] | EPHESUS study, eplerenone decreases mortality in post-MI |
Early Discoveries
The story of the RAAS begins with the discovery that the injection of an extract of renal cortex from ischemic rabbits induced an increase of blood pressure in control rabbits [11]. However, it was not until 1934 that Goldblatt and other groups confirmed the pressor effects of ischemic kidney extracts, identified as a product of renin and called hypertensin or angiotonin [12]. Then, it was realized that the effect of renin was due to a peptide produced by the proteolytic enzyme renin [13, 14]. In 1952, an increased “hypertensin” activity was evidenced in arterial blood samples of hypertensive humans compared to normotensive subjects [15]. The protein substrate, a globulin mainly produced by the liver [31], was later named angiotensinogen and the peptide named angiotensin [32]. Further works have demonstrated that angiotensin existed in two distinct forms: angiotensin I (Ang I) and angiotensin II (Ang II), where Ang I was cleaved by ACE to generate the biologically active Ang II. The relationship between Ang II and ALD hypothesized by Gross [20] was rapidly confirmed [33].
4.1.3 Clinical Observations in Hypertension
In the 1960s, the clinical problem of essential hypertension was identified but there was no consensus on the interest to treat this disorder. It had previously been shown that ganglionic blockade improved cardiac output in dogs with experimentally induced hypertension [34]. In addition, clinical observations had already demonstrated that nitroglycerin provided immediate relief in patients with dyspnea. In the 1960s, several groups demonstrated increased sympathetically mediated vasoconstriction in HF patients [35]. These discoveries paved the way to the observations that nitroprusside or nitroglycerin given in patients within the first 24 h of acute MI improved congestive symptoms, left ventricular pressure, and cardiac output. Further studies demonstrated similar hemodynamic benefits of afterload reduction in patients with chronic HF using phentolamine or nitroprusside. Thus, the role of afterload reduction in the treatment of HF was firmly established in patients and the stage was set for the next series of clinical studies using RAAS blockade in patients with hypertensive HF [36].
Before 1970, it was well-appreciated that oversecretion of ALD and renin-angiotensin were responsible for end-organ damage in adrenal tumors and in malignant hypertension. In 1972, however, Brunner et al. were the first to report that high renin levels were associated with heart attack, stroke, and LV hypertrophy in patients with essential hypertension [23]. At the same time, striking reductions in blood pressure were observed in patients with renovascular hypertension using the angiotensin peptide analog, saralasin. Saralasin had to be administered intravenously and had a 2-min half-life. It bound to the Ang II receptor and prevented Ang-II binding but, in being structurally similar to Ang II, had agonist activity. The discovery of the conversion of Ang I to the active form Ang II by angiotensin-converting enzyme (ACE), and the full characterization of the kininase II ACE were determinant for the development of the first oral ACE inhibitor, captopril.
4.1.4 The Development of ACE Inhibitors
The story of the design and synthesis of the first inhibitors of ACE is one of the great success stories of modern medicinal chemistry. It has been described as one of the first examples of true ‘rational drug design’. Several detailed reviews of this story have been published [37, 38]. In the 1960s, Sergio Ferreira prepared an extract of the venom of the Brazilian viper Bothrops jararaca and established that it potentiated the actions of bradykinin. The extract was tested on ACE and found to be a potent inhibitor thereof. A nonapeptide named teprotide, was isolated from the venom extract and tested intravenously in hypertensive patients by Dr John Laragh, a strong supporter of the ACE concept. The result was positive and this led to a top priority effort at Squibb pharmaceutical company to develop an orally active form of the drug, which company scientists (namely David Cushman and Miguel Ondetti) accomplished. Captopril (trade name Capoten) was approved by the FDA in the early 1980s and it opened a new approach for the treatment of this serious disease. When the adverse effects of Captopril (skin rash and loss of taste) became apparent new derivates were designed, first Enalaprilat and Lisinopril, then many others. Indeed, several major clinical trials using ACE inhibitors were initiated in the late 1970s, first in hypertension then in HF.
Most of the ACE inhibitors on the market today are non-selective towards the two active sites of ACE because their binding to the enzyme is based mostly on the strong interaction between the zinc atom in the enzyme and the chelating group on the inhibitor. Although N- and C-domain have comparable rates in vitro of ACE hydrolyzing, it seems like that in vivo the C-domain is mainly responsible for regulating blood pressure. Ang I is mainly hydrolyzed by the C-domain in vivo but bradykinin is hydrolyzed by both active sites. Apparently, the N-domain does not play a big role in controlling blood pressure but it seems to be the principal metabolizing enzyme for Acetyl-SDKP, a natural haemoregulatory hormone. Thus, C-domain selective inhibition could possibly result in specialized control of blood pressure with less vasodilator-related adverse effects.
4.1.5 The Development of AT1 Antagonists
The dipeptidyl carboxypeptidase ACE cleaves not only Ang I but also bradykinin, which generates enkephalins and substance P. These substrates are involved in bronchoconstriction and inflammation and their accumulation may contribute to cough or asthma-like symptoms or both. In addition, before the development of captopril, there was evidence for alternative pathways to ACE for formation of Ang II through chymase, trypsin, kallikrein, tissue plasminogen activator and cathepsin-G. The potential pathophysiological significance of alternative Ang II-forming pathways in human CVD remained obscure until Urata and Husain reported that a chymotrypsin-like serine protease accounted for 90 % of the Ang II-forming capacity in tissue extracts from human myocardium [39], suggesting that ACE was not the major Ang II-forming enzyme in the human left ventricle. To address the problems of ACE inhibitor-induced cough and alternative pathways for Ang II production, Saralasin was developed in the early 1970s. Saralasin was an octapeptide analogue of Ang II, in which three amino acids were different. Saralasin was not orally bioavailable, had a short duration of action and showed partial agonist activity and therefore it was not clinically suitable as a drug. Research investigators at Takeda company discovered in 1982 the weak nonpeptide Ang II antagonists S-8307 and S-8308 which had moderate potency and short duration of action, however they were selective AT1 receptor antagonists without partial agonist activity. Then, the pharmaceutical company DuPont modified the Takeda molecule and produced the first AT1 receptor antagonist, losartan (DuP-753) in 1989 [40]. A series of AT1 antagonists, called angiotensin receptor blockers (ARB), have been rapidly synthesized by several companies. The modifications on the molecule aimed to increase the bioavailability, elimination half-life, affinity for the AT1 receptor and activity, namely on blood pressure reduction. Telmisartan is the only ARB that can cross the blood–brain barrier and can therefore inhibit centrally mediated effects of Ang II, contributing to even better blood pressure control (Table 4.1).
4.2 Cellular Mechanisms
The ACE cleaves Ang I to give the octapeptide Ang II. In addition, several peptidases (chymase, chymotrypsin, ACE2 (a homolog of ACE) and aminopeptidase A) generate a number of bioactive peptides from angiotensin I and/or Ang II, such as Ang III, angiotensin IV and angiotensin (1–7). In the heart the majority of Ang I is converted by chymase [41]. The carboxypeptidase ACE2 cleaves one amino acid from either Ang I or Ang II, decreasing Ang II levels and increasing the metabolite Ang 1–7, which has vasodilator properties. Thus the balance between ACE and ACE2 is an important factor controlling Ang II levels. The main pathway of Ang II synthesis occurs in plasma, but so-called local RAASs have also been evidenced in the kidneys, heart, brain, adrenal glands, and possibly other tissues [42, 43]. The local RAASs operate in a paracrine or autocrine manner and it is generally considered that they work with the classical circulating RAAS in a complementary manner.
The receptor subtypes AT1 and AT2 are polypeptides containing seven trans-membrane domains [44]. Despite their similar affinities for Ang II, AT1 and AT2 receptors are functionally distinct, with a sequence homology of only 30 %. Composed of 359 amino acids, the AT1 receptor (40 kDa) belongs to the superfamily of G protein-coupled receptors. AT1 receptors bind Ang II with an affinity similar to its circulating concentration (0.1 nM) and they are regulated by their rates of biosynthesis and recycling (up- and down-regulation). Most known physiological effects of Ang II are mediated by AT1 receptors, which are widely distributed in all organs, including liver, adrenals, brain, lung, kidney, heart, and vasculature. AT2 receptors exert anti-proliferative and pro-apoptotic changes in vascular smooth muscle cells, mainly by antagonizing AT1 receptors via activation of tyrosine or serine/threonine phosphatases. AT2 receptor expression declines after birth, suggesting that it may play an important role in fetal development, and it is induced again in adult heart under pathological conditions.
4.2.1 Cardiovascular Effects of Ang II
Ang II, within seconds to minutes of binding to AT1 receptors, activates signaling pathways leading to vascular smooth muscle cell contraction, maintaining vascular tone. In addition to stimulating the synthesis and release of ALD and increasing renal Na+ absorption, Ang II’s actions on the central nervous system are critical in maintaining sympathetic outflow to the vasculature and in autoregulating cerebral blood flow. Ang II is extremely important in modulating minute to minute changes that occur in spatial adaptation. For example, when we stand up from a supine position, Ang II increases myocardial inotropy and chronotropy via an increase of inward Ca2+ current through L-type channels.
The binding of Ang II to AT1 receptor leads to the dissociation of subunits of a guanine-nucleotide-binding protein (Gq/11), which activates phospholipase C to generate diacylglycerol, and inositol trisphosphate which releases calcium from intracellular stores. Ang II also increases the entry of calcium into cells. Calcium and diacylglycerol activate various enzymes, such as protein kinase C and calcium-calmodulin kinases that catalyze the phosphorylation of proteins, and this ultimately regulates the cell functions affected by Ang II. These signal-transduction events are completed within seconds or minutes. However, they also initiate slower responses to Ang II including vascular growth and ventricular hypertrophy. The stimulation of growth by Ang II also involves other processes common to growth factors in general, such as the phosphorylation of tyrosine, and the activation of mitogen-activated protein kinase and Stat 91, proteins that affect the cell nucleus and activate DNA transcription. In vivo experiments in rats reported that AT1 receptor antagonists prevent Ang II-induced cardiac hypertrophy. The cellular responses depend on receptor biosynthesis, insertion into the membrane, internalization and degradation. The internalization of receptors is initiated by binding to hormone and is one mechanism by which target cells can become desensitized during prolonged exposure to agonists. RAAS blockade causes an increase in renin levels as a result of interference with a negative feedback loop between Ang II and renin release. This effect explains, at least in part, why Ang II and ALD breakthroughs occur. In addition to renin, the levels of its precursor, prorenin, might also increase during breakthroughs. Since the discovery of the prorenin receptor [45], which induces profibrotic effects in vitro in response to renin or prorenin binding, it has been proposed that increases in renin and prorenin levels will not only result in diminished RAAS suppression, but also in unwanted effects via the prorenin receptor stimulation.
4.2.2 Pathophysiological Role of Ang II in Cardiovascular Disorders
Experimental studies have demonstrated that abnormal elevation of Ang II and ALD levels in plasma induce structural and functional alterations in the heart, kidneys, and vessels with effects such as vascular inflammation and REM, cardiac fibrosis, nephrosclerosis, and disturbed fibrinolysis. This large spectrum of actions explains that dysregulation of the RAAS is a major factor in the development of cardiovascular pathologies including hypertension, MI, congestive HF and stroke, as well as renal disorders especially diabetic nephropathy. In atherosclerotic plaques, the local RAAS system is activated, with high levels of ACE, Ang II, and AT1 receptor. Ang II’s cytokine-like effects usually occur with longer exposure, and promote cell growth and migration, extracellular matrix deposition, and vascular and electrical remodeling. It is important to underscore that the dramatic increase of salt (and fat) in the modern food will amplify the effects of the RAAS in cardiovascular pathologies. In other words, the primitive function of the RAAS is less and less adapted to the conditions of high salt intake, and this is a problem of increasing importance [46].
4.2.2.1 Experimental Models
The experimental models of hypertension or HF are detailed in another chapter of this book. To summarize, hypertension is experimentally obtained in rodents by either strain selection (spontaneously hypertensive rats, SHR), lower aorta or renal artery ligation, or genetic intervention (REN-2 rats, Ren-TgKC mice, etc.). Severe experimental hypertension evolves generally towards with time (this is also the case in humans, but their hypertensin is cured). Heart failure is produced in rats or mice by MI, aortic stenosis, aortic insufficiency, infusion of angiotensin, ALD-salt, isoproterenol or other hormones or drugs, generally infused by osmotic minipumps, and by numerous genetic models of gene over-expression or invalidation. Each of the RAAS genes has been eliminated by gene targeting in embryonic stem cells and blood pressure phenotypes have been established for each, see review in [47]. For example, the complete absence of angiotensinogen, ACE or AT-1a receptor cause a significant decrease in blood pressure. Specific knockout of the tissue-bound ACE that does not alter circulating ACE also causes a decrease in blood pressure, thus supporting the hypothesis that tissue-bound ACE is critical in the physiologic generation of Ang II. These studies are also in agreement with those showing a lack of correlation between the hypertensive effect of ACE inhibitors and circulating levels of ACE. The decrease in arterial pressure in the AT-1a (an AT1 receptor subtype only present in rodents) knockout is similar to the blood pressure observed in angiotensinogen and ACE knockout mice, in agreement with previous observations that most of the effects of Ang II on blood pressure are mediated by AT-1a receptors. Many transgenic models have used the insertion of human renin (hRen) and/or angiotensinogen (hAGT) genes in the mouse or rat genome in order to develop models emulating human hypertension. For example, overexpression of the renin gene in liver induces hypertension and renal alterations, with a relation between the severity of the symptoms and the number of gene copies inserted [48].
4.2.2.2 Ang II, Inflammation and Remodeling
In rats, Ang II infusion for 2-weeks leads to hypertension and vascular smooth muscle cell (VSMC) hypertrophy [49]. Shear stress from elevated blood pressure has been shown to upregulate Ang II receptors [50], linking hypertension to vascular REM. Independently from the mechanical effect of hypertension that stimulates cell growth, several in vitro and in vivo experiments have shown that Ang II is an important growth factor, causing cell proliferation, VSMC hypertrophy, cell differentiation, and apoptosis [51]. The main targets of Ang II are VSMC [52]. However, it has multiple effects on endothelial cells, such as producing reactive oxygen species, activating apoptotic signaling pathways, and promoting thrombosis. The increase in oxidative stress caused by Ang II leads to impaired endothelial relaxation and endothelial dysfunction. Ang II has been shown to stimulate the production of TNF-α, an important contributor to vascular inflammation in adult mammalian heart. Ang II also stimulates the production of matrix metalloproteinases, which are necessary for vascular REM. Ang II-induced EGFR- and MAPK-dependent pathways participate in the matrix formation and regulation. Ang II-mediated EGFR transactivation regulates fibronectin and TGF-β synthesis. Additionally, production of matrix metalloproteinases like MMP-2 and breakdown of collagen IV is also modulated by Ang II. Cardiac repair occurs in the infarcted myocardium and structural REM is developed in noninfarcted myocardium, which are accompanied by activated cardiac RAAS. The spatial and temporal sequence of cardiac expression of key-components of RAAS has been nicely described using autoradiography in sections of rat infarcted myocardium [53]. In these experiments, ACE and AT1 receptor expressions are enhanced in the infarcted myocardium within a few days post-MI and cells expressing ACE and AngII receptors are primarily macrophages in the early stage of repair. These observations suggest that locally produced AngII may regulate the function of macrophages in an autocrine manner.
4.2.3 Clinical Studies
Besides the successful classes of ACE inhibitors and AT1 blockers, other RAAS inhibitors have been developed, namely direct renin inhibitor (DRI), ALD antagonists or better termed mineralocorticoid receptor antagonists (MRA), and ALD synthesis inhibitors. All these classes of drugs have proven good efficiency in animal models of hypertension, HF and MI. But the end-point being treatment of human diseases, it is necessary to verify that the promising results are confirmed in the “real life” of clinical practice. This is necessary for at least two reasons: first, patients with chronic, and often severe, CVD have treatments involving several drugs, and possible deleterious interactions between all these drugs (which are never tested in the laboratory for reasons of time and money) may only be evidenced in clinical studies enrolling hundreds to thousands of patients; secondly, approval for sale may be obtained only if the new drug brings a significant benefit over the standard of care. In this highly competitive field, several drugs have been eliminated on one of these criteria after large clinical studies.
It would be tedious here to analyze in detail the results of all clinical studies involving ACE inhibitors and ARBs. Numerous extensive and comprehensive reviews on this important topic have be published [5, 6, 8, 9].
4.2.3.1 Hypertension and Heart Failure
To summarize, ACE inhibitors have an established role as the first-line treatment for a number of cardiovascular and renal diseases. Their role in the management of hypertension is proven, and they have been shown to reduce mortality associated with both HF with reduced ejection fraction and left-ventricular dysfunction after MI. Furthermore, ACE inhibitors have been shown to reduce the rate of stroke, MI, and death in high-risk individuals without known HF. ACE inhibitors are indicated by the European Society of Cardiology in potentially all patients with symptomatic (NYHA functional class II– IV) systolic HF, with a Class of recommandation I and a Level of evidence A (together with beta blockers and mineralocorticoid receptor antagonists) [54]. In fact, there is consensus that a beta-blocker and an ACE inhibitor should both be started as soon as possible after diagnosis of HF with reduced left ventricular (LV) ejection fraction (EF). This is in part because ACE inhibitors have a modest effect on LV whereas beta-blockers often lead to a substantial improvement in EF. Furthermore, beta-blockers are anti-ischaemic and probably more effective in reducing the risk of sudden cardiac death, and lead to a striking and early reduction in overall mortality.
Angiotensin receptor blockers (ARBs) have been compared with other classes of drugs and namely ACE inhibitors in large clinical trials. The results show that ARBs remain an appropriate alternative in patients who are intolerant to ACEI.However, according to the Guidelines of the European Society of Cardiology, ARBs are no longer the first choice recommendation in patients with HF and an ejection ≤40 % who remain symptomatic despite optimal treatment with an ACE inhibitor and beta-blocker. This is because in the EMPHASIS-HF trial (discussed in the Aldosterone paragraph), eplerenone led to a larger reduction in morbidity-mortality than seen in the ARB ‘add-on’ trials, and because in both the RALES and EMPHASIS-HF studies, MR antagonists treatment reduced all-cause mortality, whereas ARB ‘add-on’ treatment did not [54]. Useful meta-analyses are often published, which help to keep a clear mind on the subtle conclusions of the large clinical trials. For example, a recent pooled analysis of 20 cardiovascular morbidity–mortality trials in hypertension, involving a total of around 160,000 patients, showed that use of ACE inhibitors was associated with a significant 10 % reduction in all-cause mortality, whereas no mortality reduction could be demonstrated with ARB treatment [55].
4.2.3.2 Combination Therapy of ACEI and ARB
The rationale of giving ACE inhibitors in combination with ARB is based on the evidence that standard doses of ACE inhibitors only offer a partial blockade of ACE. One proposed explanation was that Ang II may be generated from angiotensinogen and other peptide substrates, in a mode independent of ACE, by enzymes such as chymase or cathepsin G. Moreover, one would expect more specific Ang II blockade at AT1 receptors and theoretically unopposed AT2 receptor agonism. However, the results of several trials (CHARM-Added, VALIANT, ONTARGET) were somewhat conflicting and failed to find benefit for a combination treatment over a single drug use.
4.2.3.3 Other Pathologies
The pharmacological treatment of HF with preserved LV ejection fraction remains challenging, and effective treatment is urgently needed for this disease, which may be as common and lethal as HF with reduced LV ejection fraction. Activation of RAAS and other neurohormonal pathways occurs in HF with preserved LV ejection fraction; however, several studies of ACE inhibitors and ARBs in this disease have failed to show convincing benefit. On the other hand, several clinical trials have shown that the frequency of new onset of type 2 diabetes can be reduced by ACE inhibitors and ARBs (as opposed to beta-blockers and diuretics), thus supporting a role of RAAS in diabetes mellitus. However, the level of renin activity or Ang II in plasma of diabetic patients is generally normal and the mechanistic links remain to be determined.
4.2.3.4 Direct Renin Inhibitors (DRI)
Development of DRIs dates back to the 1980s, but the early DRIs had poor bioavailability (<2 %), a short half-life, lack of specificity, and low potency. Aliskiren is the first of a new class of nonpeptide-orally active DRIs, and further DRIs are in development. Aliskiren has limited bioavailability (2.7 %), but its half-life is 45 h, and it is therefore suitable as a once-daily medication. Aliskiren blocks the active site of renin and therefore can block the action of both renin and prorenin. It has the potential to block both circulating and tissue RAAS and has been shown to reduce renin activity. In a study of spontaneously hypertensive rats, aliskiren blocked RAAS more effectively than ACE inhibitors or ARBs. Aliskiren is effective at reducing blood pressure and is approved for the treatment of hypertension. The role of DRIs in HF has not yet been well defined, with early trials suggesting that they may have a role in HF but subsequent studies failing to show a significant impact on mortality and hospital admissions, raising clear concerns about safety. We await the outcome of the ATMOSPHERE trial, designed to test the effect of aliskiren in systolic HF, for further clarification [5].
4.2.3.5 Angiotensin Receptor-Neprilysin Inhibitors (ARNi)
Neprilysin, like ACE, is a zinc metalloproteinase enzyme that is expressed, in the kidney, lung, endothelial cells, vascular smooth muscle cells, cardiac myocytes, fibroblasts, and neutrophils, with the highest concentrations being present in the renal proximal tubule. Neprilysin degrades biologically active natriuretic peptides, including atrial natriuretic peptide, BNP, cardiac natriuretic peptide, angiotensin I, bradykinin, and endothelin-1. By augmenting the active natriuretic peptides, neprilysin inhibition increases generation of myocardial cyclic guanosine 3′,5′-monophosphate, which improves myocardial relaxation and reduces hypertrophy. However, neprilysin also contributes to the breakdown of angiotensin, which is the rationale for dual-acting compounds that both inhibit this enzyme and block the action of angiotensin. Hence, drugs that inhibit both neprilysin and ACE were developed and were referred to as vasopeptidase inhibitors. These drugs decrease peripheral vascular resistance and improve both local blood flow and the sodium/water balance. However, in the OCTAVE trial, the frequency of angioedema was three to four times higher with the first vasopeptidase inhibitor to be developed, omapatrilat, than with enalapril. Novel approaches have been explored to avoid angioedema. One proposed concept was to combine neprilysin inhibition with ARBs, i.e., a dual-acting ARB-neprilysin inhibitor (ARNI), which would not directly affect ACE or aminopeptidase and may therefore be a safer approach to inhibiting the RAAS and increasing natriuretic peptide levels. LCZ696 is a first-in-class angiotensin receptor-neprilysin inhibitor that comprises the molecular moieties of the ARB valsartan and AHU377 (the prodrug of the neprilysin inhibitor) in a 1:1 molar ratio. AHU377 is metabolized by enzymatic cleavage to LBQ657, the active inhibitor of neprilysin. Preliminary data in hypertension and in HF are promising [5].
4.3 Aldosterone
The classic role of ALD is to adjust the hydro-mineral balance in the body, and thus to participate to the regulation of blood pressure. ALD has several modes of action. The best known involves activation of the cytoplasmic mineralocorticoid receptor (MR) by binding of ALD; the MR-aldosterone complex enters the nucleus and binds to a specific DNA sequence which initiates transcription of target genes (as subunits of the amiloride-sensitive sodium channel, Na+K+ -ATPase and others) which activates sodium reabsorption in the kidney and epithelial tissues. The MRAs prevent the binding of ALD to its receptor and thus prevent its action. As discussed below, these antagonists have been first marketed as diuretics, which is probably too restrictive in regard to their recently recognized efficacy in CVD. Like other steroid hormones, ALD may also cause ionic effects (activation of sodium currents) in a few minutes at the membrane level, without activation of the MR. A third mode of action has been evidenced more recently by which ALD can quickly activate kinases, through binding to the MR but without gene transcription [56]. The physiological role of this latter intermediate mode is still poorly defined.
4.3.1 History of Aldosterone Discovery (Table 4.1)
Aldosterone, the last A of RAAS, was isolated 60 years ago. A substance secreted by the cortex of adrenal glands and having the ability to retain salt has been evidenced in the 30s by the teams of Kendall and Reichstein (for a more detailed review on the discovery of aldosterone, see [57]). From the crystallization of glucocorticoids and mineralocorticoids, researchers have discovered that a fraction extracted from adrenals was not crystallized. This so-called “amorphous” fraction had an important mineralocorticoid activity, albeit different from that of deoxycorticosterone or other steroids. A potentially confounding issue was the fact that cortisol possessed effects both on carbohydrate metabolism and electrolyte secretion, leading to a widely held opinion that cortisol was the physiologically important mineralocorticoid hormone. Nevertheless, some researchers were convinced of the existence of a mineralocorticoid different from deoxycorticosterone. The isolation of electrocortin (subsequently termed aldosterone) relied on two important technical developments: the use of partition chromatography and the development of a specific radiolabeled bioassay (Na24/K42). Sylvia and James Tait, working in London, purified a very small quantity of ALD, carried out pilot studies and identified some chemical groupings. In 1952, they began a collaboration with Pr Tadeus Reichstein in Basel. The initial isolation of adequate quantities of ALD was carried out at Ciba AG, Basel, where material from 1,500 kg of pig adrenals yield 60 mg of amorphous pure ALD, which Reichstein crystallized in August 1953. The hormone was then better characterized under the name of ALD [57]. That this hormone was secreted by the adrenal gland was then evidenced by Tait’s team, showing that the hormones extracted from beef or dog adrenal perfusate were identical.
4.3.2 Development of MR Antagonists
The development of spironolactone by the Searle laboratories in the 1950s was intended to provide a diuretic drug with a complementary mode of action to that of the diuretics currently used. When coadministered with a thiazide, it would cause an additional Na+/water loss, but also reverse the hypokalemic effects of chronic thiazide therapy. Frank Sturtevant was working on experimental mineralocorticoid and renal hypertension while in the nearby labs Charlie Kagawa was examining the effects of a spirolactones series in blocking the activities of the sodium-retaining actions of DOCA and ALD. At lunchtime, they discussed their exploratory findings with the spirolactones and suggested that the compound SC-5233 be taken into clinical trials. Thus the first ALD antagonist was discovered serendipitously using compounds synthesized for very different reasons. ln 1957, Grant Liddle at Vanderbilt University showed that SC-5233 increased the urinary excretion of sodium in a patient with congestive HF, as well as the sodium-retaining actions of DOCA in patients with Addison’s disease [19]. Then, Liddle’s group published the clinical effects of five analogs of SC-5233 in edematous patients. They concluded that a number of steroid 17-spirolactones have proved to be effective diuretic agents in man by antagonizing the renal tubular actions of ALD. One of the spirolactone analogs was SC-9420, which was subsequently named spironolactone. Spironolactone was marketed as a novel diuretic with additional antihypertensive effects [21]. While spironolactone has useful clinical effects, it has significant tolerability problems due to painful gynecomastia and menstrual disturbances in premenopausal women. These side effects are attributed to its androgenic and progesteronergic properties. The introduction of epoxy groups into the spironolactone molecule resulted in more potent and highly selective MR antagonists, of which epoxymexrenone (CGP30083) proved to be the optimal compound. This compound, now called eplerenone, was patented in 1984 and later taken into clinical trials [58].
4.3.3 Cellular Mechanisms
4.3.3.1 Biosynthesis of Aldosterone
Aldosterone is a steroid hormone synthesized from cholesterol in the zona glomerulosa of adrenal cortex. The first rate-limiting step involves a transport of cholesterol into mitochondria controlled by the steroidogenic acute regulatory protein (StAR). The last steps of its biosynthesis involve the mitochondrial P-450 aldosterone-synthase which catalyses the 11beta-hydroxylation of deoxycorticosterone to form corticosterone, then a 18-hydroxylation and 18-methyloxidation to yield ALD. This enzyme is encoded by the CYP11B2 gene and its activity is stimulated mainly by Ang II and potassium, and more weakly by ACTH and sodium. Inhibitors of aldosterone-synthase have been developed but, despite promising results in experimental HF [59], side-effects compromise their use in humans. Extra-adrenal sites of production of ALD have been also described, such as the brain, vessels and heart. The level of ALD synthesis in these organs is generally low in resting conditions, suggesting that the secreted hormone could have local autocrine or paracrine actions, if any. The relevance of ALD synthesis in heart is a matter of debate, but several observations have been made in pathological conditions where the well described stimulation of the intracardiac renin-angiotensin system could lead to an increased level of ALD within the cardiac tissue, with likely deleterious consequences. This has been observed in post-MI in rat, where the increased AngII and ALD cardiac production played a key role in the development of cardiac fibrosis [60]. Similarly, ALD production is activated in proportion to severity in failing ventricle in humans [61], and myocardial ALD and aldosterone synthase mRNA levels are elevated by 4- to 6-fold in humans with hypertrophic cardiomyopathy, and provoke expression of hypertrophic markers in rat cardiac myocytes and expression of collagens and transforming growth factor-beta1 in rat cardiac fibroblasts [62].
The Mineralocorticoid Receptor (MR)
The MR and glucocorticoid receptor (GR) belong to the nuclear hormone receptors superfamily, and they have a high sequence homology. Sequence evolution studies suggest that MR is the first receptor to have diverged within this subfamily [63]. Interestingly, primitive fishes living in salt water express the MR but not ALD, suggesting that this « orphan » MR is activated by glucocorticoids. Glucocorticoids (cortisol in humans and corticosterone in rats and mice) bind to GR and MR with a strong affinity. ALD binds to MR with strong affinity, while its affinity for GR is much lower. Since plasma ALD levels are three orders of magnitude lower than those of GR, the latter should occupy most of the MRs. However, this theoretical excess is around ten-fold decreased by the 97 % binding of GR to plasma transcortin, while the binding of ALD to albumin is lower (30 % of plasma ALD is free). In addition, transfection studies have shown that cortisol has a transactivation activity of MR ten-fold lower than that of ALD despite identical binding affinities, and the cortisol-MR complex is less stable than the ALD-MR complex due to differences in the conformation changes of MR induced by hormone binding. A first conclusion is that ALD seems disadvantaged compared to cortisol to bind to MR, but probably not as much as the ratio of plasma concentrations suggests. But in these conditions, how can ALD have a specific action?
< div class='tao-gold-member'>
Only gold members can continue reading. Log In or Register a > to continue
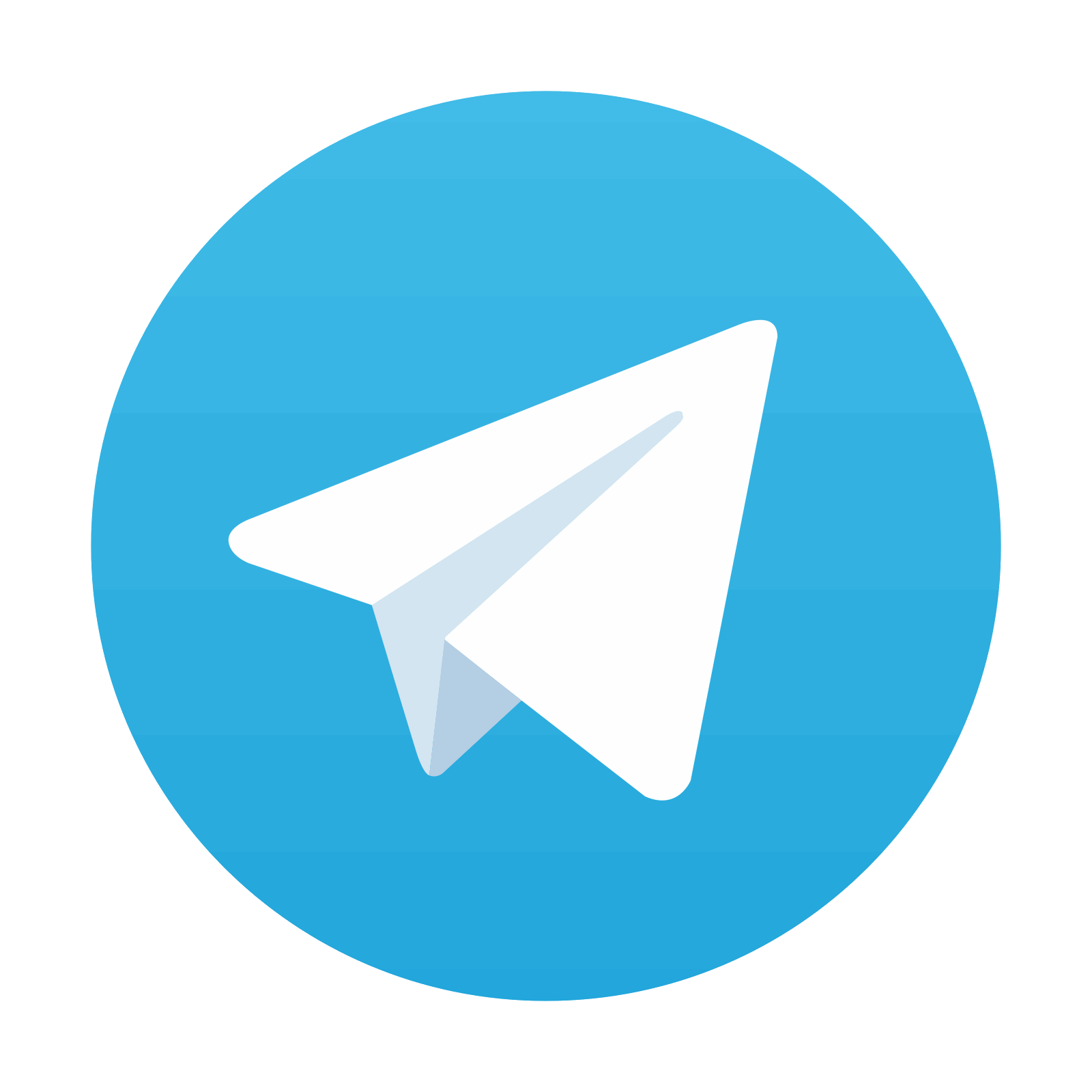
Stay updated, free articles. Join our Telegram channel

Full access? Get Clinical Tree
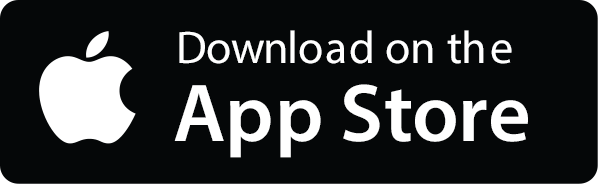
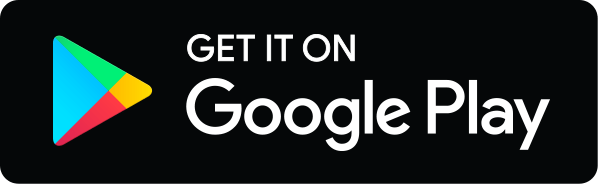