Background
Although the ratio of early mitral inflow velocity (E) to global diastolic strain rate (E′sr) has been correlated with left ventricular filling pressure, its relationship with cardiac outcomes in patients with atrial fibrillation (AF) has never been evaluated. The aim of this study was to examine the ability of E/E′sr ratio in predicting cardiac outcomes in patients with AF.
Methods
In 190 patients with persistent AF, comprehensive echocardiography was performed, with assessment of E′sr from three standard apical views using the index beat method. Cardiac events were defined as death and hospitalization for heart failure.
Results
There were 50 cardiac events, including 22 deaths and 28 hospitalizations for heart failure, during an average follow-up period of 20 months (interquartile range, 14–32 months). Multivariate analysis showed old age, chronic heart failure, and increased E/E′sr ratio (per 10-cm E/E′sr increase; hazard ratio, 1.258; 95% confidence interval, 1.132–1.398, P < .001) were associated with increased cardiac events. In direct comparison, E/E′sr ratio outperformed the ratio of E to early diastolic mitral annular velocity (E′) in predicting adverse cardiac events in both univariate and multivariate models ( P ≤ .046). Additionally, the addition of E/E′sr ratio to a clinical model including age, chronic heart failure, diabetes, hypertension, left atrial volume index, left ventricular ejection fraction, and E/E′ still provided an extra benefit in the prediction of adverse cardiac events ( P = .010).
Conclusions
E/E′sr ratio is a useful parameter and is stronger than E/E′ ratio in predicting adverse cardiac events, and it may offer additional prognostic benefit over conventional clinical and echocardiographic parameters in patients with AF.
Atrial fibrillation (AF) is the most common form of cardiac arrhythmia, and its prevalence increases with age, reaching 8% in those aged >80 years. AF is associated with an increased risk for stroke, heart failure, and all-cause mortality. Thus, the early detection of left ventricular (LV) dysfunction in patients with AF is important in the prediction of which patients are at high risk for developing cardiac events and in providing therapeutic intervention to reduce the future incidence of cardiac events and its accompanying medical and social costs. AF is frequently associated with LV diastolic dysfunction, resulting in a considerable risk for developing heart failure with preserved LV ejection fraction (LVEF). Hence, consideration of only LV systolic function may be imperfect in the prediction of adverse cardiac events in patients with AF. Additional consideration of LV diastolic function was shown to be helpful in improving outcome prediction in these patients.
Two-dimensional echocardiographic speckle-tracking can accurately measure myocardial strain and strain rate. This imaging method discriminates between active and passive myocardial motion, allows the angle-independent quantification of myocardial deformation in two dimensions, and more accurately reflects the systolic and diastolic performance of all myocardial segments. LV early global diastolic strain rate (E′sr) was reported to be a useful parameter of LV diastolic function. Furthermore, several studies have demonstrated a close correlation between invasively measured LV filling pressure and the ratio of early mitral inflow velocity (E) to E′sr. Recently, Ersbøll et al . showed that E/E′sr is independently associated with outcomes in patients with acute myocardial infarctions.
Because of beat-to-beat variation, it has traditionally been difficult to estimate LV function in patients with AF. To overcome the tedious method of averaging multiple cardiac cycles of LV function, an index beat, the beat after the nearly equal preceding and pre-preceding intervals, has been used as a substitute for the averaging technique in the evaluation of LV function in patients with AF. Kusunose et al . recently demonstrated that E′sr measured from the index beat in patients with AF is an accurate estimate of E′sr measured from averaging multiple cardiac cycles and is significantly correlated with the time constant of isovolumic LV pressure decay. In addition, they also found that E/E′sr was highly associated with LV filling pressure in patients with AF. Therefore, using the index beat method, we can easily obtain reliable values of E′sr in patients with AF. Although E/E′sr ratio is useful in prediction of outcomes in patients without AF, its relationship with adverse cardiac events in those with AF has never been evaluated. Hence, this study was designed to investigate whether E/E′sr ratio is a useful parameter in the prediction of adverse cardiac events in patients with AF. Additionally, we also evaluated the major correlates of E/E′sr ratio in these patients.
Methods
Study Patients
This observational cohort study prospectively and consecutively included patients with persistent AF referred for echocardiographic examinations at Kaohsiung Municipal Hsiao-Kang Hospital from April 2010 to June 2012. Persistent AF was defined as AF lasting for ≥7 days, which was confirmed by 12-lead electrocardiography, 24-hour Holter electrocardiography, or electrocardiographic recording during echocardiographic examination. Patients with moderate and severe mitral stenosis (n = 5), moderate and severe aortic stenosis or regurgitation (n = 4), severe mitral regurgitation (n = 5), or inadequate echocardiographic visualization (n = 11) were excluded. Additionally, four patients who had no beats fulfilling the requirements of the index beat in their stored cardiac cycles were also excluded. Finally, 190 patients with AF were included in this study. The study protocol was approved by our institutional review board, and all enrolled patients gave written informed consent.
Echocardiographic Evaluation
The echocardiographic examination was performed by one experienced cardiologist using a Vivid 7 (GE Vingmed Ultrasound AS, Horten, Norway), with the participant respiring quietly in the left decubitus position. The cardiologist was blinded to the clinical data, such as history of hypertension, diabetes mellitus, coronary artery disease, and so on. Two-dimensional and anatomic M-mode images were recorded from the standardized views. The Doppler sample volume was placed at the tips of the mitral leaflets to obtain the LV inflow waveforms from the apical four-chamber view. Pulsed Doppler tissue imaging was performed with the sample volume placed at the lateral and septal corners of the mitral annulus from the apical four-chamber view. Early diastolic mitral annular velocity (E′) was averaged from septal and lateral ones. The wall filter settings were adjusted to exclude high-frequency signals, and the gain was minimized. LVEF was measured using the modified Simpson’s method. LV mass was calculated using the modified Devereux method. LV mass index (LVMI) was calculated by dividing LV mass by body surface area. Left atrial volume was measured using the biplane area-length method. Left atrial volume index (LAVI) was calculated by dividing left atrial volume by body surface area.
LV apical four-chamber, two-chamber, and long-axis views were acquired using a high frame rate (50–90 frames/sec). The endocardial border was manually defined using a point-and-click technique. An epicardial surface tracing was automatically generated by the system, creating a region of interest, which was manually adjusted to cover the full thickness of the myocardium in the systolic frame. The ventricular chamber was divided into six segments, and six segmental strain and strain rate curves were analyzed in each apical view. Peak segmental longitudinal systolic strain and early diastolic strain rate measurements were determined from these curves. E′sr and global LV longitudinal systolic strain (GLS) were assessed in the 18 LV segments from the three standard apical views. The values in the 18 LV segments were averaged to give the mean value for later analysis. The minimum number of LV segments for acceptable E′sr and GLS measurements was 15. We used cine loops to determine which beat would be calculated. The raw ultrasonic data, including 15 consecutive beats from the three standard apical views, were recorded and analyzed offline using EchoPAC version 08 (GE Vingmed Ultrasound AS).
LV dimensions, LVEF, LAVI, LVMI, GLS, and E′sr were measured from the index beat. Because their measurements were easy and rapid, E, E-wave deceleration time, and E′ were obtained from five beats and then averaged for later analysis. If the cardiac cycle length was too short to complete the diastolic process, this beat was skipped. Thus, the selection of E, E-wave deceleration time, and E′ was not always consecutive. In addition, heart rate was determined from five consecutive beats.
Index Beat Selection
The index beat taken after the nearly equal preceding and pre-preceding intervals was selected from the 15 stored cardiac cycles. The preceding and pre-preceding intervals of the index beat must be >500 msec, and the difference between them must be <60 msec. The cardiac cycle of the index beat was also >500 msec ( Figure 1 ). If patients had no beats fulfilling the requirements of the index beat in the 15 stored cardiac cycles in any apical view, they were excluded. If several beats fulfilled the requirements of the index beat in the 15 stored cardiac cycles, we chose the index beat of first appearance to calculate the echocardiographic data.

Collection of Demographic, Medical, and Laboratory Data
Demographic and medical data including age, gender, and history of diabetes mellitus, hypertension, coronary artery disease, stroke, and chronic heart failure were obtained from medical records or interviews with patients. Body mass index was calculated as the ratio of weight in kilograms to the square of height in meters. Systolic and diastolic blood pressures were measured using a mercury sphygmomanometer before echocardiographic examination. Study subjects were defined as having diabetes mellitus if the fasting blood glucose level was >126 mg/dL or hypoglycemic agents were used to control blood glucose levels. Similarly, study patients were considered to have hypertension if systolic blood pressure was ≥140 mm Hg, diastolic blood pressure was ≥90 mm Hg, or antihypertensive drugs were prescribed. Stroke was defined as a history of cerebrovascular accident, including cerebral bleeding and infarction. Coronary artery disease was defined as a history of typical angina with positive stress test results, angiographically documented coronary artery disease, old myocardial infarction, or having undergone coronary artery bypass surgery or angioplasty. Heart failure was defined according to Framingham criteria. Laboratory data including total cholesterol and triglyceride levels were also collected. In addition, information regarding patient medications, including angiotensin-converting enzyme inhibitors, angiotensin II receptor blockers, β-blockers, calcium channel blockers, diuretics, and antiplatelet and anticoagulant drugs, during the study period was obtained from medical records.
Definition of Cardiac Events
Cardiac events were defined as all-cause mortality and hospitalization for heart failure. Hospitalization for heart failure was defined as admission for dyspnea with chest radiographic evidence of pulmonary congestion and treatment with intravenous diuretics. Cardiac events were ascertained and adjudicated by two cardiologists, with disagreements resolved by adjudication from a third cardiologist from the hospital course and medical record. If patients had multiple cardiac events, only the first event was coded. However, if patients died after episodes of heart failure during the same admission, they were coded as deaths. Patients reaching the study end points were followed until the first episodes of adverse events. The other patients were followed until May 2013.
Reproducibility
Thirty patients were randomly selected for evaluation of the interobserver variability of E′sr measurement by two independent observers. To obtain intraobserver variability, the same measurement was repeated 1 week apart. Mean percentage error was calculated as the absolute difference divided by the average of the two observations.
Statistical Analysis
SPSS version 18.0 (SPSS, Inc, Chicago, IL) was used for statistical analysis. Data are expressed as mean ± SD, percentages, or median (interquartile range) for follow-up period. Continuous and categorical variables among groups were compared using one-way analysis of variance, followed by a post hoc test adjusted with Bonferroni’s correction and a χ 2 test, respectively. The relationship between two continuous variables was assessed using a bivariate correlation method (Pearson’s correlation). The significant variables in the univariate analysis were selected for multivariate analysis. Time to the adverse events and covariates of risk factors were modeled using a Cox proportional-hazards model with forward selection. Direct comparison between E/E′ and E/E′sr was performed in both univariate and multivariate models. Incremental model performance was assessed by change in the χ 2 value. A Kaplan-Meier survival plot was calculated from baseline to the time of adverse events and compared using the log-rank test. Stepwise multiple linear regression analysis was used to identify the determinants of E′sr and E/E′sr ratio. All tests were two sided, and the level of significance was established as P < .05.
Results
Table 1 shows the comparison of clinical and echocardiographic characteristics according to the tertile of E/E′sr ratio. The mean value of E/E′sr was 64.7 ± 25.2 cm, and patients in tertiles 1, 2, and 3 had E/E′sr ratios of <51.6, 51.6 to 66.3, and >66.3 cm, respectively. The mean age of all patients was 70 ± 10 years. There were significant differences in the prevalence of coronary artery disease and chronic heart failure, anticoagulant use, LV end-systolic and end-diastolic dimensions, LVMI, LVEF, E, E-wave deceleration time, E′, E/E′ ratio, GLS, E′sr, and E/E′sr ratio among patients in different tertiles.
Characteristic | Tertile 1 (E/E′sr < 51.6 cm) (n = 63) | Tertile 2 (E/E′sr 51.6–66.3 cm) (n = 63) | Tertile 3 (E/E′sr > 66.3 cm) (n = 64) | P | All patients (n = 190) |
---|---|---|---|---|---|
Age (y) | 71 ± 10 | 69 ± 8 | 71 ± 11 | .432 | 70 ± 10 |
Men | 41 (65.1%) | 40 (63.5%) | 47 (73.4%) | .438 | 128 (67.4%) |
Diabetes mellitus | 16 (25.4%) | 15 (23.8%) | 21 (32.8%) | .477 | 52 (27.4%) |
Hypertension | 39 (61.9%) | 40 (63.5%) | 46 (71.9%) | .444 | 125 (65.8%) |
CAD | 4 (6.3%) | 3 (4.8%) | 13 (20.3%) ∗ † | .007 | 20 (10.5%) |
Stroke | 6 (9.5%) | 13 (20.6%) | 14 (21.9%) | .130 | 33 (17.4%) |
CHF | 13 (20.6%) | 12 (19.0%) | 30 (46.9%) ∗ † | .001 | 55 (28.9%) |
SBP (mm Hg) | 132 ± 21 | 133 ± 19 | 132 ± 19 | .957 | 133 ± 20 |
DBP (mm Hg) | 77 ± 12 | 77 ± 11 | 77 ± 13 | .952 | 77 ± 12 |
Heart rate (beats/min) | 81 ± 20 | 84 ± 18 | 84 ± 22 | .542 | 83 ± 20 |
Body mass index (kg/m 2 ) | 25.5 ± 3.5 | 26.9 ± 4.0 | 26.4 ± 4.6 | .195 | 26.3 ± 4.1 |
Triglyceride (mg/dL) | 124 ± 89 | 123 ± 71 | 120 ± 79 | .972 | 123 ± 79 |
Total cholesterol (mg/dL) | 177 ± 42 | 175 ± 33 | 167 ± 32 | .340 | 173 ± 36 |
Medications | |||||
ACE inhibitors and/or ARBs | 40 (63.5%) | 30 (47.6%) | 34 (53.1%) | .192 | 104 (54.7%) |
β-blockers | 28 (42.9%) | 31 (49.2%) | 25 (39.1%) | .508 | 83 (43.7%) |
CCBs | 22 (34.9%) | 19 (30.2%) | 24 (37.5%) | .677 | 65 (34.2%) |
Diuretics | 28 (44.4%) | 21 (33.3%) | 31 (48.4%) | .204 | 80 (42.1%) |
Antiplatelet agents | 39 (61.9%) | 34 (54.0%) | 39 (60.9%) | .613 | 112 (58.9%) |
Anticoagulants | 11 (17.5%) | 22 (34.9%) ∗ | 22 (34.4%) ∗ | .049 | 55 (28.9%) |
Echocardiographic data | |||||
LAVI (mL/m 2 ) | 46 ± 21 | 46 ± 13 | 52 ± 23 | .165 | 48 ± 20 |
LVEDD (mm) | 49 ± 6 | 51 ± 6 | 55 ± 10 ∗ † | <.001 | 52 ± 8 |
LVESD (mm) | 33 ± 7 | 35 ± 7 | 39 ± 12 ∗ † | <.001 | 36 ± 9 |
LVMI (g/m 2 ) | 126 ± 36 | 133 ± 34 | 151 ± 45 ∗ † | .001 | 137 ± 40 |
LVEF (%) | 61 ± 10 | 55 ± 13 ∗ | 48 ± 17 ∗ † | <.001 | 55 ± 14 |
E (cm/sec) | 84 ± 16 | 96 ± 17 ∗ | 110 ± 26 ∗ † | <.001 | 97 ± 23 |
EDT (msec) | 140 ± 27 | 143 ± 26 | 165 ± 67 ∗ † | .004 | 149 ± 46 |
E′ (cm/sec) | 9.7 ± 2.3 | 9.3 ± 2.2 | 7.5 ± 1.8 ∗ † | <.001 | 8.8 ± 2.4 |
E/E′ ratio | 9.0 ± 2.3 | 10.6 ± 2.4 ∗ | 15.5 ± 5.2 ∗ † | <.001 | 11.8 ± 4.5 |
GLS (%) | −14.8 ± 2.8 | −13.6 ± 3.0 | −11.1 ± 3.4 ∗ † | <.001 | −13.1 ± 3.4 |
E′sr (sec −1 ) | 1.91 ± 0.38 | 1.64 ± 0.28 ∗ | 1.26 ± 0.33 ∗ † | <.001 | 1.60 ± 0.42 |
E/E′sr ratio (cm) | 44.3 ± 4.8 | 58.4 ± 4.6 ∗ | 90.9 ± 26.5 ∗ † | <.001 | 64.7 ± 25.2 |
∗ P < .05 compared with tertile 1.
The follow-up period to cardiac events was 20 months (interquartile range, 14–32 months) in all patients. Fifty cardiac events were documented during the follow-up period, including death (n = 22) and hospitalization for heart failure (n = 28). A Cox proportional-hazards regression analysis for cardiac events is shown in Table 2 . In the univariate analysis, E/E′sr ratio was significantly related to adverse cardiac events (per 10-cm E/E′sr increase; hazard ratio, 1.295; 95% confidence interval, 1.190–1.410; P < .001) and outperformed the model with E/E′ ratio in direct comparison (χ 2 = 39.71 vs 35.73, P = .046). In the multivariate analysis, old age, the presence of chronic heart failure, and increased E/E′sr ratio (per 10-cm E/E′sr increase; hazard ratio, 1.258; 95% confidence interval, 1.132–1.398; P < .001) were independently associated with increased cardiac events. In direct comparison, the multivariate model without E/E′ and E/E′sr (global χ 2 = 83.14) was nonsignificantly improved by adding E/E′ (global χ 2 = 85.56, P = .120), whereas the addition of E/E′sr instead caused a significant improvement (global χ 2 = 91.46, P = .004). Additionally, in the univariate analysis, E/E′sr still outperformed the model with the ratio of E to E′ measured using the index beat method in direct comparison (χ 2 = 39.71 vs 31.64, P = .005).
Parameter | Univariate | Multivariate (forward) | ||
---|---|---|---|---|
HR (95% CI) | P | HR (95% CI) | P | |
Age (per 5 y) | 1.254 (1.078–1.458) | .003 | 1.247 (1.076–1.447) | .003 |
Male vs female | 1.087 (0.605–1.951) | .781 | ||
Diabetes mellitus | 1.453 (0.801–2.637) | .217 | ||
Hypertension | 0.716 (0.408–1.256) | .242 | ||
CAD | 1.795 (0.842–3.826) | .124 | ||
Stroke | 0.729 (0.310–1.7124) | .466 | ||
CHF | 4.378 (2.493–7.688) | <.001 | 3.397 (1.864–6.188) | <.001 |
SBP (per 1 mm Hg) | 1.002 (0.987–1.018) | .754 | ||
DBP (per 1 mm Hg) | 0.996 (0.971–1.021) | .749 | ||
Heart rate (per 1 beat/min) | 1.006 (0.992–1.020) | .393 | ||
Body mass index (per 1 kg/m 2 ) | 0.898 (0.826–0.975) | .012 | ||
Triglyceride (per 1 mg/dL) | 0.998 (0.994–1.003) | .502 | ||
Total cholesterol (per 1 mg/dL) | 0.997 (0.988–1.006) | .515 | ||
Medications | ||||
ACE inhibitors and/or ARBs | 1.124 (0.641–1.972) | .683 | ||
β-blockers | 0.861 (0.491–1.511) | .602 | ||
CCBs | 1.246 (0.698–2.224) | .456 | ||
Diuretics | 2.690 (1.509–4.972) | <.001 | ||
Antiplatelet agents | 0.822 (0.471–1.434) | .489 | ||
Anticoagulants | 1.111 (0.590–2.091) | .744 | ||
Echocardiographic data | ||||
LAVI (per 1 mL/m 2 ) | 1.012 (0.999–1.012) | .071 | ||
LVEDD (per 1 mm) | 1.058 (1.021–1.096) | .002 | ||
LVESD (per 1 mm) | 1.064 (1.034–1.095) | <.001 | ||
LVMI (per 10 g/m 2 ) | 1.153 (1.080–1.230) | <.001 | 1.074 (1.001–1.153) | .047 |
LVEF (per 1%) | 0.962 (0.944–0.981) | .001 | ||
E (per 1 cm/sec) | 1.014 (1.002–1.026) | .017 | ||
EDT (per 1 msec) | 1.005 (1.000–1.011) | .039 | ||
E′ (per 1 cm/sec) | 0.721 (0.631–0.823) | <.001 | ||
E/E′ ratio (per 1) | 1.125 (1.081–1.171) | <.001 | ||
GLS (per 1%) | 1.163 (1.070–1.264) | <.001 | ||
E′sr (per 1 sec −1 ) | 0.203 (0.098–0.423) | <.001 | ||
E/E′sr ratio (per 10 cm) | 1.295 (1.190–1.410) | <.001 | 1.258 (1.132–1.398) | <.001 |
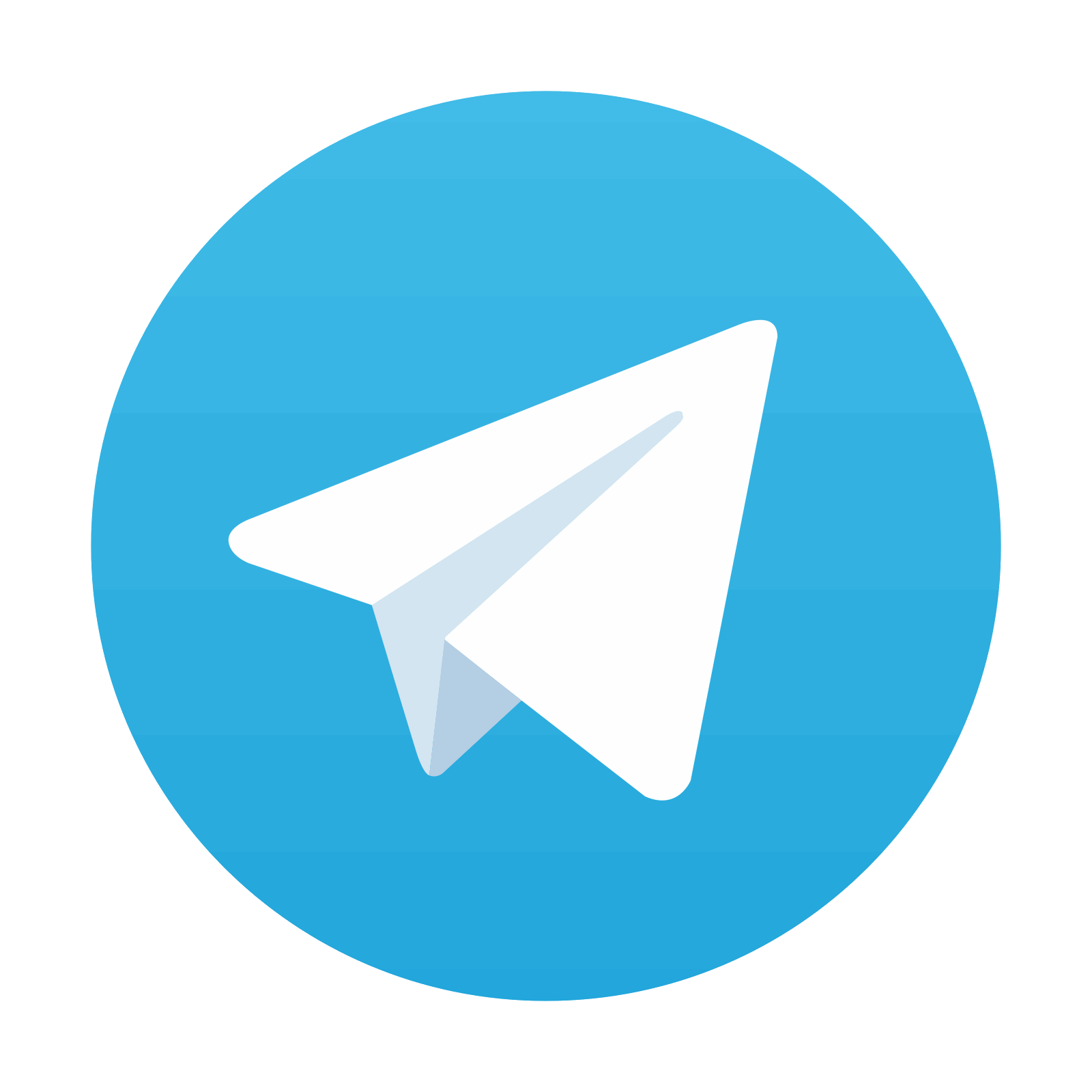
Stay updated, free articles. Join our Telegram channel

Full access? Get Clinical Tree
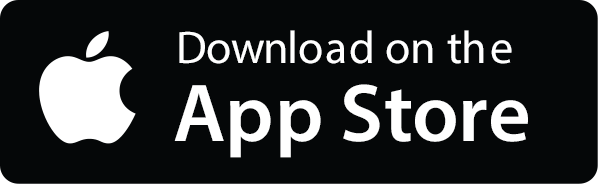
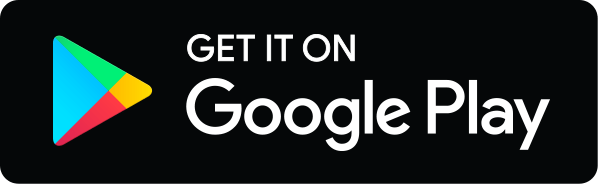
