Introduction
The placenta is one of the most important and least understood organs in the human body. It is a complex, highly organized structure that—amazingly—can be grown, discarded, and fully grown again in a repetitive manner. More than just an interface for fetal oxygenation and nutrition, the placenta is now appreciated to exert important influences that affect health and well-being for the lifespan of the individual. Placental structure and function influence organogenesis during the early vulnerable period of fetal development, a time in which cells and organ systems are highly plastic and sensitive to their biologic environment. The placenta leaves an imprint in organ development that not only alters the substrate presenting at birth but also can leave a mark that influences organ functionality lifelong. Therefore placental structure and function is an important system to understand within the context of congenital heart disease (CHD).
The study of prenatal factors influencing postnatal outcomes in CHD has not been extensively explored but is now gaining interest. David Barker was the first to introduce the concept of “fetal programming,” or the fetal origins of adult disease hypothesis. Barker first described this phenomenon in relation to the findings of an association between diminished fetal growth and low birth weight with increased risk of later adult-onset coronary artery disease, hypertension, and diabetes. Subsequently, a host of other mature adult conditions have now been linked to prenatal deficiencies. The placenta is the lynchpin. Variations in the processes of placental development lead to variations in the supply of nutrients to the fetus and program a number of key systems that are linked to later disease. As we look to optimize and improve outcomes for individuals with CHD, it makes sense to improve our understanding of how our biologic systems are set at the outset before birth and how it may differ in the population of individuals with CHD.
This chapter reviews the development of the human placenta, conditions of placental disease as models for prenatal dysfunction, and what is currently known concerning the placenta in congenital heart malformations.
Normal Placental Development
The embryo’s early growth and development take place in a fairly hypoxic environment and are supported by uterine gland secretions, which are directly absorbed through phagocytosis. It is not until the end of the first trimester that nutrient delivery shifts to blood-based exchange via the placenta. In preparation, however, the placenta begins to form soon after fertilization and develops in tandem with organs in the embryo.
The mature placenta consists of three layers. The chorionic plate is the layer closest to the fetus, where the umbilical cord attaches. From the chorionic plate, a complex network of nutrient-absorbing villi extends into the intervillous space ( Fig. 11.1 ). The villi are organized into lobules, or villous trees, and correspond with cotyledons on the maternal surface of the placenta. Maternal spiral arteries provide flow into the intervillous space of each lobule, allowing for nutrient and gas exchange. The space drains via endometrial veins between arterial pulsations. While many villi float in this nutrient-rich bath, anchoring villi traverse the intervillous space to connect the chorionic plate to the basal plate on the maternal side. The basal plate is a combination of maternal endometrium, which has remodeled into the decidua, and fetal tissues. As pregnancy progresses, fibrin, secretions and apoptotic cells accumulate in various layers in the placenta; the layer that accumulates in the basal plate is where the placenta will detach at birth.

This complex organ begins to form between 6 and 7 days postfertilization, with implantation. On day 5, a blastocyst is created when cells polarize into an inner cell mass and a fluid cavity, surrounded by a single layer of trophoblast cells. Trophoblast cells are the precursors to the placenta and fetal membranes, whereas the inner cell mass gives rise to the embryo, the umbilical cord, and the placental mesenchyme. Implantation begins with the blastocyst adhering to the uterine epithelium, and is complete when the blastocyst is fully embedded in the endometrium, 12 days postfertilization.
Only trophoblast cells that lie over the inner cell mass are able to facilitate successful implantation. Thus the blastocyst must be oriented with the inner cell mass closest to the uterine epithelium for optimal placentation. Two trophoblast cell types emerge: cytotrophoblasts, the stem cells of the placenta, and the multinucleated syncytiotrophoblast, which forms from the fusion of cytotrophoblasts. The syncytiotrophoblast will eventually become a single multinucleated body that covers the entire surface of the intervillous space, thus serving as the barrier between fetal tissues and maternal blood. Once the blastocyst has formed a stable attachment, the syncytiotrophoblast secretes digestive enzymes that allow for the final, invasive stage of implantation. The syncytiotrophoblast forms projections that advance into fluid-filled spaces, called lacunae, created by the enzymes. As these projections or trabeculae grow, the lacunae coalesce and expand, eroding into maternal capillaries. Maternal blood cells are now able to enter the fluid-filled space surrounding the trabeculae. However, exposure to arterial maternal blood flow and the establishment of placental circulation does not occur until the end of the first trimester.
Between 11 and 13 days after fertilization, cytotrophoblast cells create invaginations in the trabeculae of syncytiotrophoblast and become primary villi. The fluid-filled space around the villi has now become the intervillous space. Around day 16, mesenchymal cells follow the invaginations made by the cytotrophoblast cells and transform the villi into secondary villi. Finally, tertiary villi form, which contain mesenchymal connective tissue, fetal vessels, and macrophages, encased in the single multinucleated syncytiotrophoblast.
Around this time, some cytotrophoblast cells also begin migrating beyond the syncytiotrophoblast into maternal tissues, becoming extravillous or interstitial trophoblasts. Trophoblast invasion will continue through the second trimester, eventually extending into the inner third of the myometrium. This invasion is facilitated by migration-inducing chemokines in the decidua, while simultaneously inhibited by cytokines promoting attachment over further invasion. Extravillous trophoblasts travel down within the lumen of the endometrial spiral arteries, replacing endothelial cells, while interstitial trophoblasts enter the vessel walls directly. The spiral arteries of the endometrium lose smooth muscle and dilate in response to hormonal signaling and this trophoblast invasion. Initially, the density of extravillous trophoblast invasion is such that the spiral arteries are effectively plugged, preventing arterial maternal blood flow into the intravillous space. By 10 to 12 weeks after implantation, the plugs loosen and the unobstructed spiral arteries, now continuous with the intervillous space, bathe the villi with maternal blood. The uteroplacental circulation is thus established, with spiral arteries feeding into a large, low-impedance lake surrounding the villi. Nutrition and oxygen exchange occur as the blood flows over the villi. From 15 weeks onward, as the uteroplacental circulation introduces oxygen to a previously hypoxemic environment, further invasion of the spiral arteries takes place. Remodeling of maternal arteries normally extends beyond the spiral arteries and the decidual border into the radial arteries of the myometrium. Arteriovenous shunts also form in the maternal myometrium underneath the placenta, which support placental circulation by decreasing maternal systemic vascular resistance.
Thus the human placenta forms from the interweaving of maternal and fetal tissues, guided by a complex interplay of hormones, cytokines, and growth factors. By the end of the first trimester the placenta has developed into its definitive state. Although trophoblast invasion continues through 19 to 20 weeks, the fetus has become dependent on the uteroplacental circulation.
Mechanisms and Conditions of Placental Dysfunction
Placental dysfunction is the direct consequence of impaired placental development. It can trigger a myriad of conditions affecting both mother and fetus, including miscarriage, premature rupture of membranes, fetal growth restriction, and the hypertensive disorders of pregnancy, including preeclampsia. For definition purposes, preeclampsia is a disorder of pregnancy believed to develop due to placental dysfunction and is characterized by the onset of maternal hypertension and proteinuria. As severity progresses it can lead to thrombocytopenia, hemolysis, hepatic dysfunction, renal dysfunction, peripheral edema, and visual disturbances. In this advanced form, some refer to the condition as HELLP syndrome (hemolysis, elevated liver enzymes, and low platelet count). If untreated, preeclampsia can lead to seizures, at which point it is then known as eclampsia. Many of these clinical symptoms are believed to result from a maternal inflammatory reaction to placental dysfunction with maternal endothelial dysfunction and increased vascular reactivity.
Many pathologic findings are associated with placental dysfunction, which can be loosely grouped into three categories: defective trophoblast invasion and failure of spiral artery dilation, vascular lesions termed “atherosis,” and lesions of the mature placenta. However, how these findings are interrelated, and the roles they play in the mechanisms of the various clinical manifestations, is still somewhat unclear.
Defective trophoblast invasion and subsequent failure of spiral artery dilation have been shown to be associated in particular with early-onset preeclampsia, fetal growth restriction, and premature rupture of membranes. Beginning even before fertilization, if the uterine decidua is insufficiently prepared, trophoblast invasion may be suboptimal. Abnormal trophoblast invasion prevents adequate spiral artery remodeling and dilation, not only leading to decreased flow into the placenta but loss of the potentially oxygen-driven deep trophoblastic invasion and arterial remodeling. In severe preeclampsia, deep placentation is severely affected, with few spiral arteries demonstrating full transformation, compared to 90% transformation in normal pregnancies. Premature loss of the spiral artery trophoblast plugs can result in miscarriage or preeclampsia, depending on the location and timing. Normally, blood flow from spiral arteries begins at the center of the placenta first, and spreads to the margins; flow that begins at the margins instead may result in high oxidative stress and villous regression. Conversely, if intervillous flow does not reach the placental margins, there can be chorionic regression and subsequently a small placenta.
Atherosis is another common finding in states of placental dysfunction. It can be seen in preeclampsia, hypertensive disease without preeclampsia, fetal growth restriction without maternal hypertension, and systemic lupus erythematosus. Lipophage plaques and fibrin accumulate in spiral arteries, and fibrinoid necrosis occurs. In addition to physically obstructing flow, the resulting vasculopathy leads to endothelial disruption and a prothrombotic state, as well as aneurysm formation in the weakened arterial walls.
Over time, macroscopic lesions develop in the placenta. Without adequate dilation of the spiral arteries, maternal arterial blood flow enters the intravillous space with greater velocity, creating intervillous lakes. These abnormal pockets often become lined with thrombus due to altered flow dynamics. When severe, anchoring villi can be ruptured, pushing the chorionic plate away from the basal plate. This perpetuates further placental disorder as fewer extravillous trophoblasts are able to reach the maternal surface to remodel the spiral arteries.
As the structure of the placenta is disrupted, the delicate balance of oxygen and nutrient exchange is also affected. In the classic state of fetal growth restriction, uterine venous blood has higher oxygen content than normal, which may be due to the higher velocity of arterial blood entering a structurally disrupted intervillous space resulting in suboptimal gas exchange. Moreover, without spiral artery remodeling, the vasocontractility of the vessels remains intact. These arteries are less able to provide adequate flow to keep up with increasing fetal demand as pregnancy progresses. Their vasoreactivity can also cause episodes of placental hypoxia and reperfusion, leading to oxidative stress, apoptosis, and necrosis. This, in turn, can trigger or promote a maternal hyperinflammatory response.
Not every pregnancy with imperfect placentation results in clinically significant disease, and the clinical manifestations of diseases are not consistent. Although many of these findings can be seen in intrauterine growth restriction and preeclampsia, not all fetuses of preeclamptic pregnancies are growth restricted.
Less dramatic aberrancies in placental development nevertheless have an impact. In situations where the inner cell mass of the blastocyst is imperfectly aligned at implantation, a smaller region of syncytiotrophoblast invasion takes place, resulting in a smaller placenta and a degree of fetal growth restriction. Smaller placentas are also seen when the umbilical cord inserts at the margin or into fetal membranes instead of the central placental mass. Infants with such placentas have been found to be proportionally smaller. Even when the umbilical cord insertion is eccentric but not marginal, the efficiency of the placental circulation is reduced and birth weight is smaller than would be expected for the placental weight.
Placental insufficiency is a multifactorial failure of the maternal and fetal tissues to form the placenta. Suboptimal uterine preparation, impaired decidualization, atypical maternal immune response to trophoblast invasion, and a host of potential aberrancies in the complex signaling involved in placental development contribute to the spectrum of clinical disease.
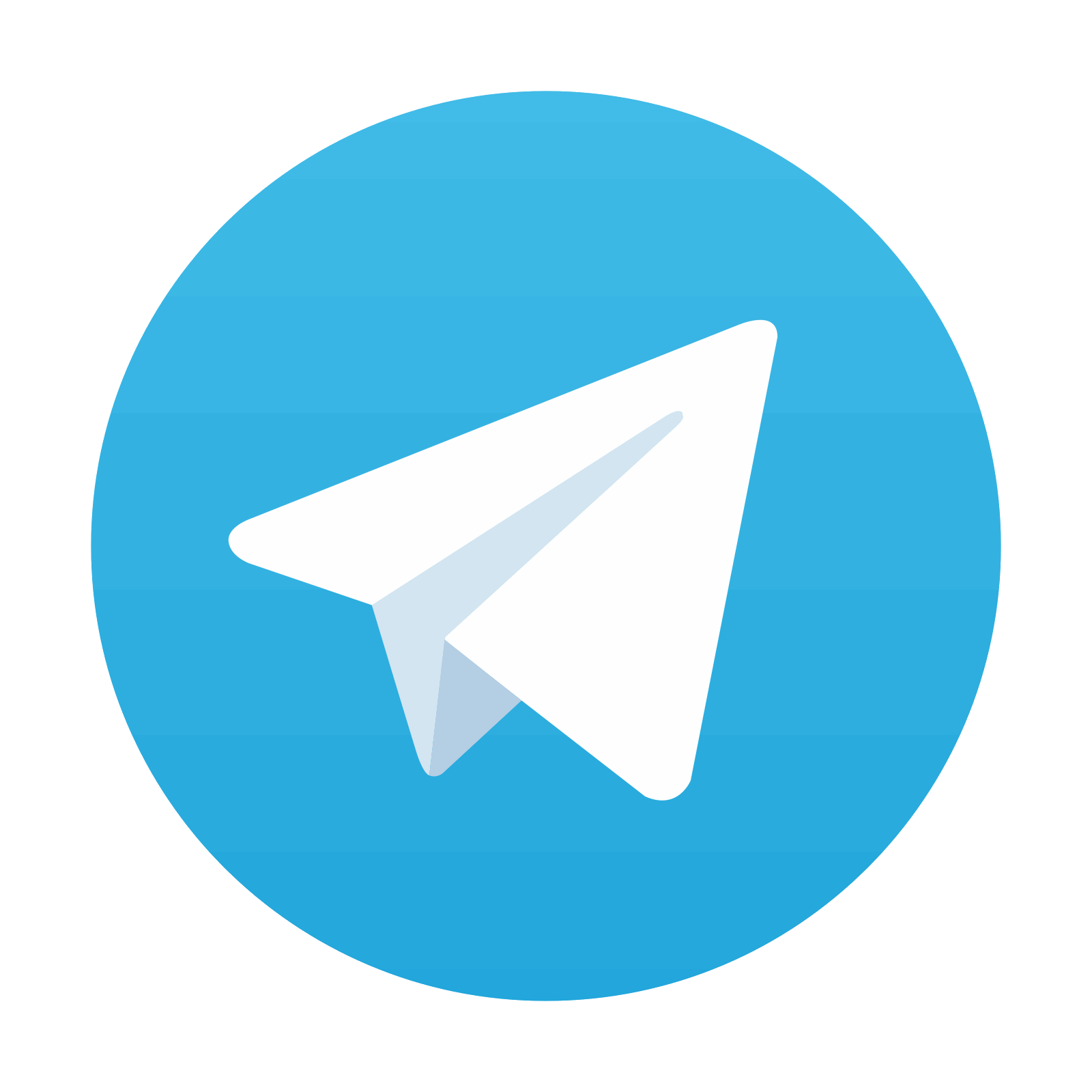
Stay updated, free articles. Join our Telegram channel

Full access? Get Clinical Tree
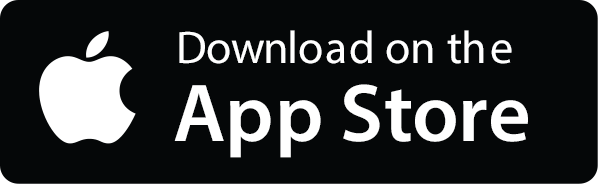
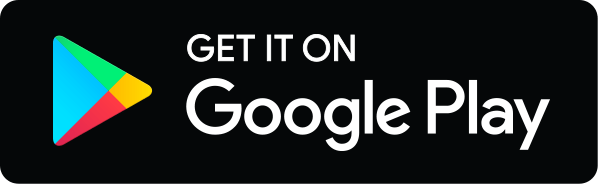