Fig. 21.1
From left to right: Arthur Otis, Hermann Rahn, and Wallace Fenn at the 1963 Fall meeting of the American Physiological Society in Coral Gables, Florida. (From [31])
21.2 Unlikely Beginnings
Wallace Fenn (1893–1971) was the leader of the group and he was some 20 years older than his two colleagues. His father was a Unitarian minister who became Professor of Theology at Harvard, and Wallace entered Harvard planning to prepare for the ministry [22]. However his interests turned to physiology and he obtained his PhD at Harvard in plant physiology. He then spent a period in the laboratory of A.V. Hill in London where a major interest was muscle energetics. There he described the “Fenn effect” which refers to the heat produced by muscle during contraction [2]. He is also well known for his work on potassium efflux from contracting muscle [4]. In addition his group showed for the first time that both oxygen poisoning and the biological effects of X-irradiation exert their damaging effects by the action of free radicals [9]. Fenn was a towering figure in American and international physiology being president of both the American Physiological Society and the International Union of Physiological Sciences, and a member of the National Academy of Sciences.
Hermann Rahn (1912–1990) was born in Lansing, Michigan, and his early life was remarkably disruptive [17]. His father, Otto, was on the faculty of Michigan State University having emigrated from Germany to the US in 1907. In 1914 Otto and his wife took the 2-year-old Hermann to Germany to visit his grandparents but World War I began while they were there. Otto was not allowed to return to the US in spite of the fact that he was in the process of becoming a US citizen but was drafted into the German army. After the war he was not invited to return to Michigan because of the anti-German sentiment at that time and the family suffered severe deprivation. It was not until 1926 that the 14-year-old Hermann found himself in Ithaca, NY where his father had obtained a faculty appointment at Cornell University. Hermann then had the task of learning English. Not surprisingly he had strong links with Germany and at one stage it was uncertain whether he would return or decide to stay in the US. Fortunately he remained, and after graduating from Cornell he obtained his PhD at the University of Rochester where his work was on the placenta and corpus luteum of snakes [18] and the development of the pituitary gland in birds [10]. He then went to the University of Wyoming at Laramie to study the reproductive behavior of rattlesnakes [19]. In 1941 he stopped briefly in Rochester en route from Woods Hole to Laramie and called on Fenn whom he admired. At the end of their conversation Rahn accepted a job as instructor of physiology in Rochester at the princely salary of $ 60 per month.
Arthur Otis (1913–2008) received his PhD from Brown University where his dissertation was on the study of the effects of drugs and ions on the oyster heart. Subsequently he spent a post-doctoral year at Iowa working on the development of grasshopper eggs [1]. He then moved to Rochester in 1942 to work in Fenn’s department. Later he spent a period at Johns Hopkins Medical School and finally he became chairman of the department of physiology at the new medical school at the University of Florida in Gainesville [12].
So here we have a trio of scientists who are working on very different areas of cellular physiology in a variety of animals. Fenn has made important contributions to muscle contraction, Rahn is working on reproduction in rattlesnakes, and Otis is studying the activation of the enzyme tyrosinase in grasshopper eggs. None of them has had any serious exposure to respiratory physiology. On the face of it this seems an unlikely group to lay the foundations of both respiratory gas exchange and mechanics as they exist today. However this is what happened as a result of the demands of the war.
21.3 The Initial Research Topic
Rahn relates that he started work in Fenn’s department on September 1, 1941 on hormone assays, but on December 7 when the attack on Pearl Harbor occurred, Fenn decided that the research should be switched to problems related to the war effort [14]. Apparently Fenn had previously been apprised of the likely need of research on aviation physiology and after the war began he was contracted by A.N. Richards of the Office of Scientific Research and Development (OSRD) to work on the physiologic effects of pressure breathing. Fenn later wrote that he “timidly obtained” a grant of $ 500 (sic) from the OSRD and that this was the first research grant he had ever received in 17 years as chairman of the department of physiology [3] Fenn was always ambivalent about federal grant support and after mentioning the $ 500 stated “It must be admitted, however, that there are dangers in this easy money regime which must be recognized and guarded against”.
The pace of work must have been exceptionally fast because on January 7, 1943 the first report appeared [5] and Fenn stated that it covered the first 6 months of the work. The cover page of the report is shown in Fig. 21.2 and as the table of contents indicates, a number of projects were reported on. The principal authors were Fenn, Rahn and Otis but several other investigators were included.
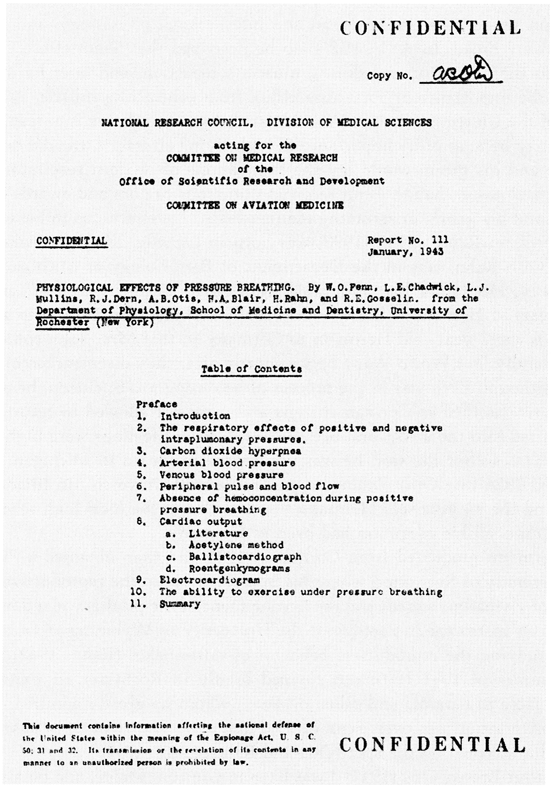
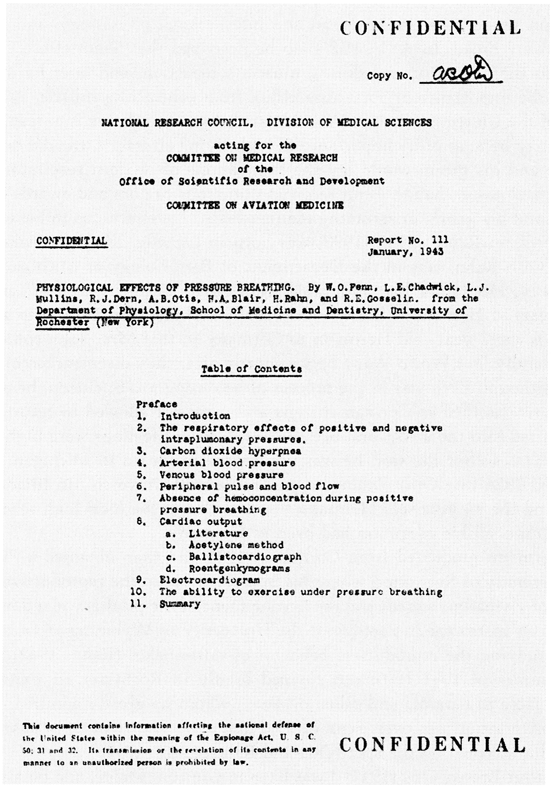
Fig. 21.2
Title page of the first report made after 6 months of work principally by Fenn, Rahn and Otis. See text for details. (From [5])
In retrospect it seems odd that pressure breathing should have been given such emphasis when there were so many other important physiological issues in aviation physiology. The reason was the belief at the time that if fighter aircraft could fly as high as 40,000 ft they would have a commanding advantage. Interestingly this belief was also shared by the air forces of the US, UK and Canada. In fact most of the sorties in the war were carried out at altitudes of 20,000–25,000 ft although reconnaissance flights were made higher. Pressurization of aircraft cabins had begun in about 1939 with the first commercial example being the Boeing 307 Stratoliner but essentially no fighter aircraft were pressurized during World War II.
The development of equipment for the new research project makes entertaining reading. Otis and Rahn described [14] how Fenn bought a steel tank designed for the transport of beer, borrowed a tree sprayer pump from the university grounds department, reversed its valves, and thus provided a high-altitude chamber that could decompress at the rate of 5000 ft/min (Fig. 21.3). Subjects entered the chamber by lowering themselves through a small circular hatch at the top. A Fleisch pneumotachograph was made from a cluster of soda straws inside a brass tube with the pressure difference between the two ends being measured with a sensitive membrane manometer. A later version replaced the soda straws with glass wool enclosed in a ladies’ hair net. Fenn was endowed with Yankee ingenuity as well as parsimony and loved to improvise. A key piece of equipment for the studies of gas exchange was an end-tidal gas sampler using a condom [27].
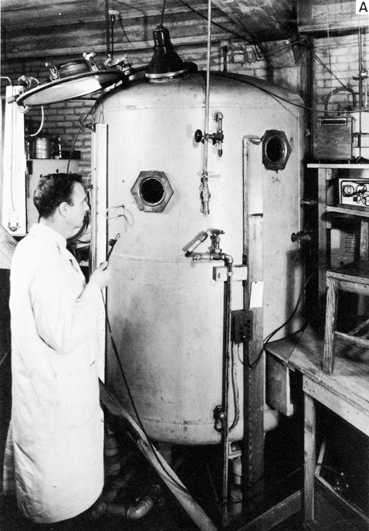
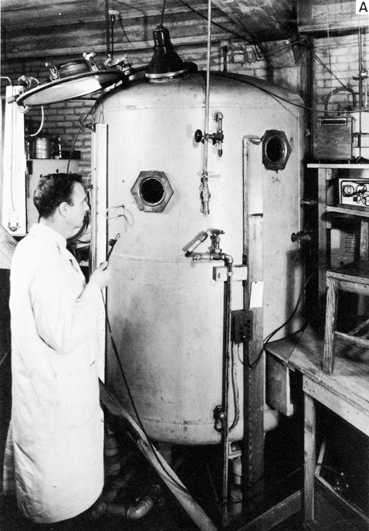
Fig. 21.3
The high altitude chamber used for the work by Fenn, Rahn and Otis. The original tank was for transporting beer. Arthur Otis is the observer. (From [14])
The report itself [14]) makes fascinating reading even today. One’s first impression is that it is extraordinary how much was accomplished in 6 months. The report begins by stating that it has been shown that positive-pressure breathing is a very effective way of gaining altitude in that for each 5 cm H2O increase in pressure, the tolerable altitude was increased by about 1000 ft. The authors noted that there were a number of measurements of very brief periods of pressure breathing such as the Valsalva maneuver in the literature as well as studies on animals, but few if any on humans. In the report, studies were made at positive pressures as high as 40 cm H2O, and negative pressure breathing was also investigated down to − 30 cm H2O. The periods of pressure breathing varied from 15 s to 2 h. The general conclusion was that pressure breathing of 20–25 mm Hg (27–33 cm H2O) was well-tolerated by normal men.
One of the early figures in the report shows the variation in maximal inspiratory and expiratory pressure with changes in lung volume (Fig. 21.4). This figure remains part of the classical teaching today and emphasizes how the research into a very practical problem such as pressure breathing throws light on general physiological principles. The report then goes on to calculate the work of breathing and they discuss both the elastic resistance of the lung and chest wall and the viscous losses. The oxygen cost of pressure breathing was also measured. Incidentally it is sometimes stated that this group were unaware of previous studies of respiratory mechanics especially those reported in the German literature. To some extent this is true (see later) but the list of references on page 11 includes four in German, a language that Rahn was fluent in.
There is a short section on the effects of raising the inspired Pco2 during pressure breathing which shows the expected increase in ventilation. One finding was that during positive-pressure breathing the alveolar Pco2 tends to increase which the authors attribute to an increase in dead space. The effects of positive-pressure breathing on arterial blood pressure were studied and both systolic and diastolic pressure were shown to increase. It was also found that peripheral venous pressure rose with positive-pressure breathing presumably because of obstruction to venous return. Because of the possibility that the increased venous pressure could cause movement of fluid out of the circulation as a result of disturbing the Starling equilibrium, the hemoglobin concentration was measured but shown not to be affected.
The effect of positive-pressure breathing on cardiac output was examined. The authors pointed out that there are numerous studies showing that in animals cardiac output is decreased with increased intrathoracic pressure presumably because of restricted venous return. However cardiac output in humans proved to be difficult to study. Measurements were made using the uptake of acetylene during rebreathing and these appeared to show a reduction in cardiac output although the scatter of the results was high. Some measurements were also made with a homemade ballistocardiograph and these seem to show that pressure breathing caused a decrease in stroke volume but that this was compensated by an increase in heart rate. A few measurements of stroke volume were also made using images of the heart obtained by chest x-ray. These showed a small reduction in stroke volume.
There is a remarkable sentence on page 35 of the report that states “The large decrease [of stroke volume] found for W.O.F. seems very improbable although this subject has collapsed three times under pressure breathing and might have been near this when the film was taken”. That rather ominous sentence fits with a short section in the account by Otis and Rahn [14]. “In the early days of our altitude chamber operations, Wallace Fenn was always the first to volunteer when a new procedure was to be tried out, but when the dean of our school, Dr. George Whipple, one day came by at an opportune time to see Wallace passing out from a mask leak at high altitude, he immediately gave strict orders to us that Fenn should henceforth not be allowed to enter the chamber”.
This initial report dealing with the first 6 months of research emphasizes the flying start of the research group. Following this there was a very fertile period of some 15 years devoted mainly to pulmonary gas exchange and pulmonary mechanics although some other topics were investigated as well. Initially all the work was classified and could not appear in the open literature. The result was a series of classified technical reports, several of them titled “Studies in respiratory physiology” that were put out by the US Air Force, Dayton, Ohio [7, 21, 23]. It was through contracts with this organization that most of the work was done. The reports continued to appear after Rahn moved to Buffalo and eventually there were a total of seven [31]. They make good reading even today.
In the summer of 1945 shortly after the end of World War II, the material was declassified, and the authors began to prepare articles for the open literature. The first public oral presentations were made at the FASEB Meeting in Atlantic City in the spring of 1946, and in that year the first publications occurred in the American Journal of Physiology and the Journal of Aviation Medicine [7, 15, 26]. At about this time it was felt that a new journal might be more appropriate than the American Journal of Physiology for these studies in applied physiology. This was one of the reasons why the American Physiological Society started the Journal of Applied Physiology in 1949. The Foreword to volume I of the Journal includes the sentence “In connection with the Journal, the term ‘applied’ will broadly connote human physiology, with particular emphasis on man in relation to his environment”. The early volumes of this journal contain much of the work of this group. However not all of the 100 or so reports in the “Studies in respiratory physiology” series ended up in the open literature.
< div class='tao-gold-member'>
Only gold members can continue reading. Log In or Register a > to continue
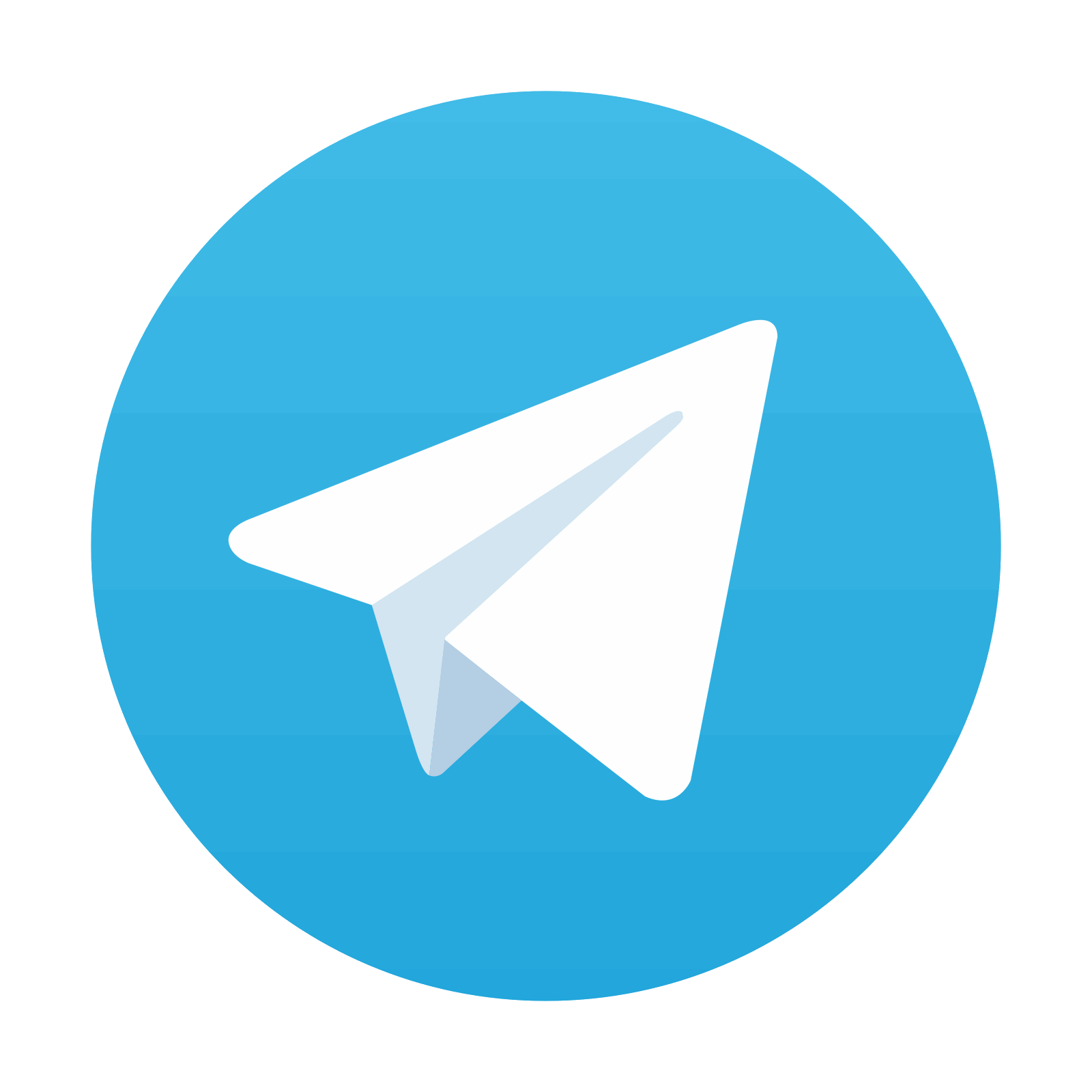
Stay updated, free articles. Join our Telegram channel

Full access? Get Clinical Tree
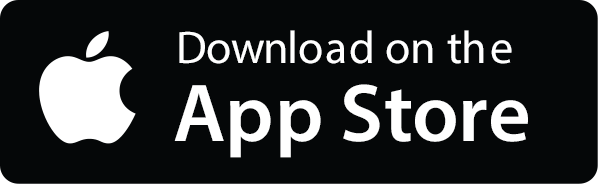
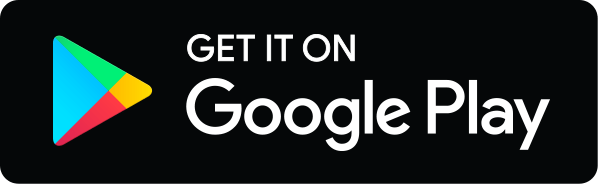