The Pathology
LESION
Duplex Ultrasonography (US) is currently the standard tool for the initial evaluation of a patient suspected of having carotid artery disease. The rating of carotid artery disease by US is based upon recorded velocity waveforms and B-mode images. The latter are generally enhanced with color flow coding.
There are some instances where US does not provide a full assessment of the condition of the patient; the most frequent being (1) carotid lesions located outside the space limited by the jaw above and the clavicle below. Changes in waveform and velocity recorded at the bifurcation can detect the existence of these lesions within the chest or cranium, but they cannot define their significance; (2) a heavily calcified bifurcation that does not permit reliable US imaging or velocity sampling; (3) fibromuscular dysplasia (FMD) or dissection of the ICA that tends to occur distal to the area that can be interrogated by US.
CTA is the diagnostic tool most often used to settle questions that cannot be clarified by US. It is less expensive than MRA and is available at most institutions. A CTA of the head and neck gives information regarding the extra- and intracranial course of both ICA and VA as well as pertinent details regarding the completeness or otherwise of the circle of Willis. The accompanying CT provides a useful scan of the brain parenchyma.
Today non-contrast CT and CTA are the most useful tools in planning an operation or an intervention in the carotid or vertebral arteries of a symptomatic patient, excepting patients with VBI secondary to neck rotation (see below). Modern multisection CT machines can produce a CTA, including post-processing manipulations, in 30 minutes.1 The efficiency of these new systems is quite relevant to the initiation of treatment after acute stroke because most protocols require not exceeding a 3-hour window from the appearance of symptoms before establishing therapy.
The incidence of silent infarction in asymptomatic patients with carotid bifurcation disease is 17%.2 If, in addition to having a known carotid plaque, a patient has experienced a TIA, there is a 45% probability of finding an infarction on CT.3 This high probability of unsuspected infarction should have made it mandatory to obtain a non-contrast CT before recruiting a patient to any randomized study that attempts to compare the efficiency or risk of a carotid procedure. The absence of a baseline CT marrs the interpretation of many randomized studies since we could never know if the lesions that were observed in follow-up were present at the time of recruitment.
Although we think of a TIA as a temporary and reversible perfusion defect, up to 34% of patients who have TIAs actually have an appropriate infarct3 seen in CT.
A CTA obtained prior to a CEA in a symptomatic patient will allow us to rule out lesions (in the common carotid artery or carotid syphon), which are outside the field surveyed by the US. A CTA will also show the location and size of the infarctions that we can anticipate finding in one-third of the patients with clinical TIAs.
A CTA obtained prior to a CEA in a symptomatic patient will allow us to rule out lesions (in the common carotid artery or carotid syphon), which are outside the field surveyed by the US. A CTA will also show the location and size of the infarctions that we can anticipate finding in one-third of the patients with clinical TIAs.
MRA has been proposed as the diagnostic tool to use when calcium deposits block the US. But MRA is not ubiquitous and is expensive. Furthermore, it tends to overestimate the severity of lesions4 located in a segment where flow abruptly changes direction, as in the origin of the ICA.
Angiography is seldom used nowadays in the workup of ICA atherosclerotic disease but it may still be needed to evaluate plaques in the intracranial segment or in the supraortic trunks (SAT). Arteriography may be necessary for the evaluation of some aneurysms and AV fistulae.
The usefulness of CTA is limited in the workup of patients with VBI. If a patient has had a TIA or a stroke of VB distribution, the CT may show a cerebellar infarction but it is unlikely to show the minute pontine infarcts that follow the occlusion of the thin perforating arteries supplying the pons. The dense bone that surrounds the brain stem causes refraction artifacts that dampen the optical resolution of the CT in this area. CTA studies with rotation/extension of the head are not feasible because it is difficult to access the patient’s head and neck and to maintain the head in the trigger position while scanning. MRA, on the other hand, is the best imaging technique to assess the parenchyma of the VB territory particularly to detect the small infarcts that develop in the brain stem that is supplied by small perforating arteries. Patients with VBI triggered by head and neck rotation need to be evaluated by dynamic arteriography, as described in Section “Dynamic Compression of the Vertebral Artery (Bow Hunter Syndrome) p.57.”
COMPOSITION OF THE PLAQUE
The classic and now outdated ACAS and NASCET studies rated the severity of a plaque on the basis of percent diameter stenosis (although the referential diameter to which the narrowed lumen was compared was different for each of these studies) and showed a correlation between the severity of the stenosis and the likelihood of brain ischemia. Percent diameter reduction is of standard and general use in describing the severity of an occlusive lesion: it is a metric easy to define and can be used to describe the size of a plaque with any of the current imaging tools: US, CTA, MRA, and angiography. Bur percent stenosis describes only the relative size of the plaque in reference to the lumen of the artery. There is no linearity between the size of the plaque and its virulence.
From NASCET arose the recommendation to restrict surgery to symptomatic plaques rated at greater than 75% diameter stenosis. While this heuristic has become accepted medical practice, there are other characteristics of the plaque, more relevant than the percent diameter stenoses, that have been left out of the decision tree. This fixation on the percent stenosis as the single indicator of the severity of a carotid plaque in most randomized studies has translated into a lack of appreciation of the role played by other equally relevant factors, such as the presence of hypoechoic areas and intraplaque hemorrhage, the rupture of the fibrous cap, or the presence of ulceration.
The largest mechanical stresses bearing on the plaque occur in its fibrous cap. These stresses increase when the lipid core is near the fibrous cap and may cause the cap to rupture, embolize, and result in brain ischemia. If, in addition, there are areas of discrete calcification, the peak stresses may increase by as much as 70%.5
The work of Russell,6 Biasi,7 Sabetai8 and others showed the clinical relevance of assessing the ultrasonic density of plaque by gray scale median (GSM) rating. The ICAROS study,7 done in patients undergoing angioplasty and stenting of the ICA, adopted an arbitrary value of 25 GSM as the boundary between hyperechoic and hypoechoic plaques. Plaques with a low GSM value have a high lipid and hemorrhagic content and are more likely to result in a stroke (microembolization) following angioplasty and stenting.
The characteristics that contribute to the morbidity of a plaque can be summarized as follows: little fibrous tissue, large areas of lipid content or presence of intraplaque hemorrhage, and rupture of the cap with resulting ulceration.
Nearly all ischemic events caused by a plaque result from particulate embolization of the contents of an ulcer or from the detachment of platelet clusters or thrombus that have formed in the downstream boundary of a plaque. Only a minority of ischemic events are caused by the restriction of flow imposed by the plaque. Given that older plaques are larger and contain more degenerative features, it is not surprising that there is some correlation between plaque size and plaque morbidity. But size alone should not be the only criterion to gauge the potential threat of a plaque. A symptomatic patient with a carotid US showing morbid features (ulceration, intraplaque hemorrhage, etc.) in the appropriate carotid should be considered for operation/intervention regardless of whether it satisfies the 70% to 99% criterion. On the other hand, there is no evidence that a 75% stenosis caused by a mostly fibrous lesion (intimal hyperplasia) in an asymptomatic patient requires an endarterectomy to improve the chances that the patient will live stroke-free for the remainder of his life.
FIBROMUSCULAR DYSPLASIA
FMD is a disease of medium-sized muscular arteries affecting their middle and distal segments; their proximal segment is generally spared. FMD is most commonly found in the renal arteries and is followed in frequency by the ICA and VA. It affects predominantly young and middle-aged women. Genetic and familial studies9 suggest that FMD is transmitted as an autosomal dominant trait with reduced penetrance in males. Most patients with FMD of the VA also have FMD lesions in their ICA. If a patient presents with FMD of an ICA, the chance that the contralateral ICA will be affected is approximately 50%. FMD of the ICA is far more common among relatives of patients who have renal FMD. Patients with FMD of the carotid and vertebral arteries also have a high incidence (20% to 50%) of “berry” type intracranial ICA aneurysms.10
The ICA and the VA usually display the characteristic changes of FMD at about the level of C2 and this high location makes US inadequate to visualize the lesion. In older reports, angiography was the diagnostic tool commonly used. In modern series, the diagnosis of FMD is made by MRA or CTA.
Three types of FMD are classically described.11 Most of the cases fall under the medial or perimedial FMD that causes the iconic “string of beads” appearance. The
ridges are made of fibroproliferative tissue and the outpouchings (microaneurysms) occur where smooth muscle is deficient or where the internal elastic membrane is missing. Intimal FMD, which results in focal concentric stenosis, occurs in less than 10% of cases. Adventitial hyperplasia is the rarest form.
ridges are made of fibroproliferative tissue and the outpouchings (microaneurysms) occur where smooth muscle is deficient or where the internal elastic membrane is missing. Intimal FMD, which results in focal concentric stenosis, occurs in less than 10% of cases. Adventitial hyperplasia is the rarest form.
CAROTID AND VERTEBRAL ARTERY DISSECTION
Although we talk about an “intimal flap” in dissections of the ICA or VA, the plane of dissection is not beneath the intima but rather within the media. In the extracranial ICA and VA (muscular arteries), the flap (inner layer) is made up of intima, internal elastic membrane, and a thin layer of media. The remaining wall (outer layer) is composed of the remaining media and adventitia.
A dissection is typically described as a progressing intramural hematoma resulting from a rent of the inner layer of the artery through which blood insinuates itself into the media. And indeed, all recent dissections have blood within the media, but, in some cases, the intimal tear that is presumed to be the source of this medial hematoma is not found. It is believed that these latter patients may have experienced shear forces in the media that tore the radially laid vasa vasora causing the intramural hemorrhage. In most cases, the dissecting hematoma will develop a distal tear through which the hematoma decompresses into the arterial blood stream. Thrombus within the false lumen may embolize downstream through this distal tear.
In the ICA and VA, the plane of dissection is beneath the internal elastic membrane and therefore the flap is thin (Fig. 3.1). The intramural hematoma makes the thin flap bulge into the lumen causing the latter to narrow (“string sign” in arteriograms) or to occlude. This intramural hematoma may continue to grow, distending the cleavage layer it occupies and eventually expanding under the adventitia. This sequence of events usually results in an aneurysm.12 If the hematoma ruptures through the adventitia, a false aneurysm will develop.
Dissections of the IA or CCA are either due to trauma (Figs. 3.2 and 3.3) or due to the extension of a Type A aortic dissection.
![]() FIGURE 3.1. Cross-section of a dissection in the cervical ICA. |
In their intracranial segments, the ICA and the VA have a much thinner wall and, as a consequence, the dissecting hematoma may rupture through the adventitia resulting in a subarachnoid hemorrhage, most often fatal.13 Dissection of the intracranial ICA or VA is rare but carries a grim prognosis. Intracranial dissection if the ICA is rare in adults but more common in children.14 For reasons unknown, boys experience 73% of all the dissection seen in children and this male predominance is found in dissections that are preceded by trauma (14%) and in those that are “spontaneous” (86%).
The considerations that follow apply to the far more common extracranial dissections of the ICA and VA. There must be either a new applied mechanical force or a tissue defect from an underlying arteriopathy to explain a dissection of the ICA. This latter proposition is supported by the fact that in spontaneous ICA dissection we find a synchronous dissection of the other ICA or of the VA in 6% to 15% of the cases. Presumably in these latter cases the ICAs and VAs would share the arteriopathy that eventually resulted in the symptomatic dissection of the one ICA. Furthermore, the ICAs of patients with spontaneous dissection display a higher than normal Young’s elastic modulus and circumferential wall stress in both the dissected and non-dissected sides.
However, patients with spontaneous carotid dissection have normal elastic parameters in their aorta and radial arteries.15 Ehler-Danlos patients,16 who are at increased risk for dissection or rupture of their vascular lesions, have a thinner intima-media layer and higher circumferential wall stress in their carotid arteries but, again, the elastic parameters of their radial arteries are normal. These findings suggest that the matrix defect in these cases is confined to the arteries supplying the brain.
There is a statistically weak association between spontaneous dissection of the ICA and connective tissue disorders such as aortic root dilatation, intracranial aneurysm, and FMD.17, 18 Lesions of FMD are seen in 15% of patients with spontaneous ICA dissection and 5% of patients with spontaneous ICA dissection have a family member with a history of dissection of the aorta or its branches. A hypothesis proposed for this association, weak as it is, is that the latter arteries share some unknown matrix defect with the ICA.
A load straining the wall of the carotid artery results first in the failure of the intima-media layer. Such failure may be caused by a “normal” load on a weakened wall (spontaneous) (Fig. 3.4) or a very high load on a normal arterial wall (trauma) (Fig. 3.5). The sequence of failure of the two layers can be shown (Fig. 3.6) by placing a strip of CCA in a tensile strength machine and progressively straining it.19 The first layer to tear is the intima-media layer. As straining continues, additional tears develop in this layer and, eventually, the adventitia-media layer ruptures.
The dissection of the ICA generally stops at the entrance of the bony temporal canal where the adventitia of the carotid is bound to the surrounding periosteum (Fig. 3.7). Rarely, an ICA dissection results in multiple aneurysms (Fig. 3.8). Similarly, the dissection of the extracranial VA usually stops at the point where the artery penetrates the duramater and exchanges collagen anchors with it. In 10% of cases a dissection of either ICA or VA may extend intracranially. The distal extension of an intracranial VA dissection is extremely risky: if it reaches the BA the mortality is 90%.20
ICA/VA dissection is the cause of 20% of the strokes seen in people younger than 50 years old.12 By far the most common dissection site is the high cervical
portion of the ICA/VA. In the ICA this preferred location is the consequence of its close relationship with the transverse process of C1 (Fig. 3.4). For the VA it is the result of the exceptional mobility of the C1-C2 joint that can mechanically stress the artery (Fig. 3.5). Of these extracranial dissections, one-third of them follow blunt trauma but in two-thirds of cases we call them “spontaneous” because no proximate cause is associated with their appearance.
portion of the ICA/VA. In the ICA this preferred location is the consequence of its close relationship with the transverse process of C1 (Fig. 3.4). For the VA it is the result of the exceptional mobility of the C1-C2 joint that can mechanically stress the artery (Fig. 3.5). Of these extracranial dissections, one-third of them follow blunt trauma but in two-thirds of cases we call them “spontaneous” because no proximate cause is associated with their appearance.
![]() FIGURE 3.4. Spontaneous dissection of the ICA at C2. |
![]() FIGURE 3.6. A strip of ICA (with location dots placed on the intima) is stretched between the jaws of a tensile strength machine (A). Note the initial fractures (B, C) of the inner layers (arrows) that preceded the rupture of the specimen. (Reproduced with permission from Stemper BD, Yoganandan N, Pintar FA. Methodology to study intimal failure mechanics in human internal carotid arteries. J Biomech. 2005;38:2491-2496.) |
![]() FIGURE 3.7. The dissecting hematoma in the ICA stops at the carotid canal (arrow). |
![]() FIGURE 3.8. Unusual development of two sequential aneurysms from an ICA dissection. |
Most imaging studies done in patients with ICA dissection in the last 20 years have used MR.21 But a comparative study22 of different modalities concluded that CTA offers the better spatial resolution for the local features of ICA dissection (seeing the flap, identifying the thrombus in the false lumen, etc). MR, on the other hand, is better at assessing the embolic consequences of dissection (infarction) in the brain parenchyma.
The typical presentation of an ICA dissection is head and neck pain and a partial (miosis and ptosis) Horner’s.23, i Patients with VA dissection present with pain in the occipital and posterior neck regions that may be misinterpreted as being of musculoskeletal origin.
A few days later (on an average four) brain ischemic symptoms appear, most commonly a stroke (84%) and less frequently a TIA. In both ICA and VA dissection, there is an interval of several days between the appearance of local symptoms (neck pain,
Horner’s) and the development of brain ischemic symptoms. This delay is the time it takes for the dissecting hematoma to occlude the lumen or to release thromboembolic fragments from the initial tear or progress distally and, through a distal tear, reenter the true lumen discharging fragments of thrombus into it. The end-organ effects in ICA dissection are hemispheric or retinal ischemia. In VA dissection, the infarction will be in the brain stem (lateral medullary syndrome), thalamus, cerebellum, and occipital lobes.
Horner’s) and the development of brain ischemic symptoms. This delay is the time it takes for the dissecting hematoma to occlude the lumen or to release thromboembolic fragments from the initial tear or progress distally and, through a distal tear, reenter the true lumen discharging fragments of thrombus into it. The end-organ effects in ICA dissection are hemispheric or retinal ischemia. In VA dissection, the infarction will be in the brain stem (lateral medullary syndrome), thalamus, cerebellum, and occipital lobes.
One-half of patients with spontaneous ICA dissection develop a brain infarction and this infarct is embolic in 90% of casesii; not due to low flow as it is often surmised.24, 25
The rate of clinical redissection of the same ICA or VA is reported to be low from 0.4%26 to 8.0%.27 Redissection is more common in patients with underlying arteriopathy.28 It is believed that an ICA/VA that has dissected and eventually healed has added enough scarring to its media to prevent the reoccurrence of separation of the wall layers. This incidence of redissection turns out to be higher when the patients who have had a dissection are followed with routine imaging. In this latter group, 6% will have a silent synchronous dissection of the opposite ICA or VA and 25% will eventually show a redissection or a new dissection in an ICA or VA.
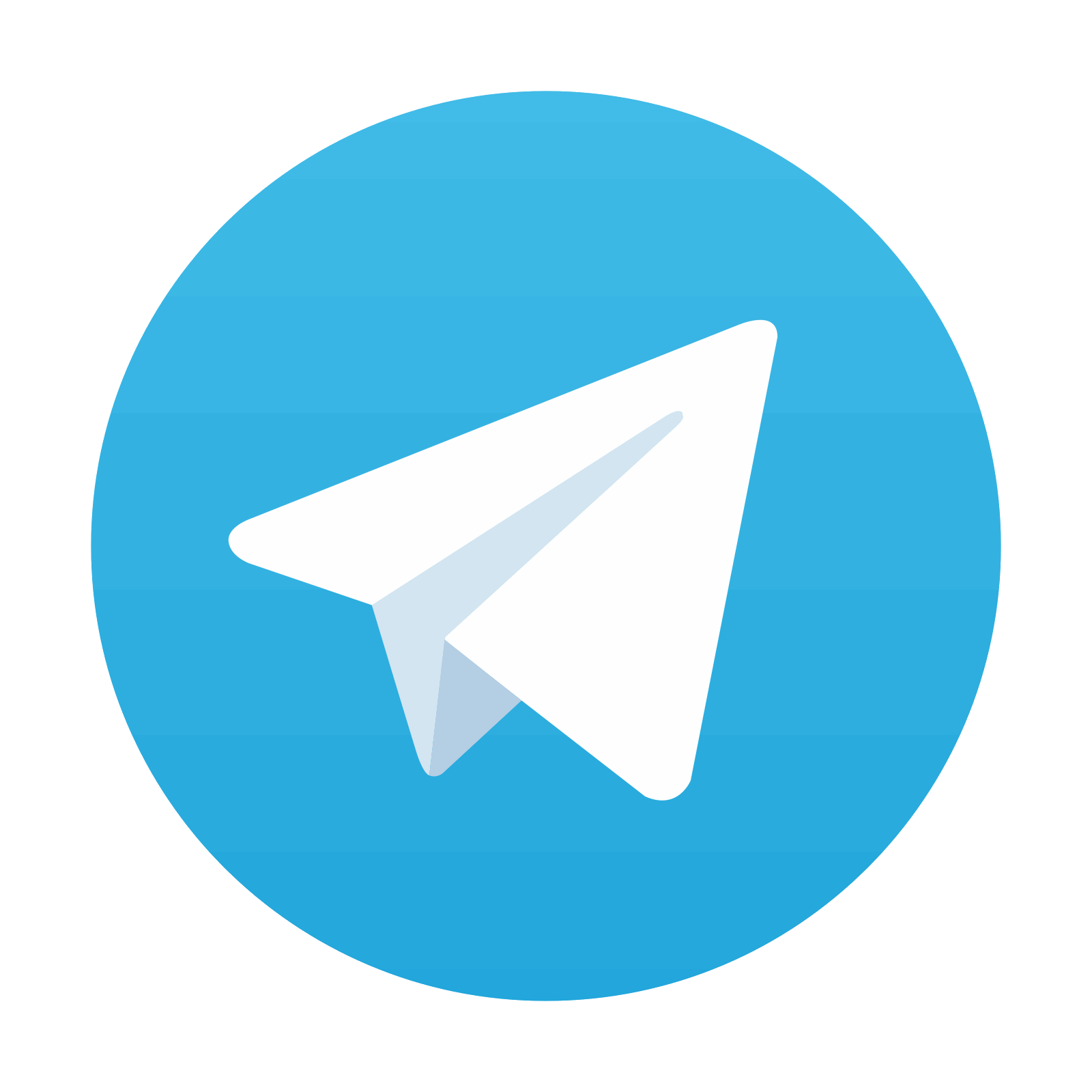
Stay updated, free articles. Join our Telegram channel

Full access? Get Clinical Tree
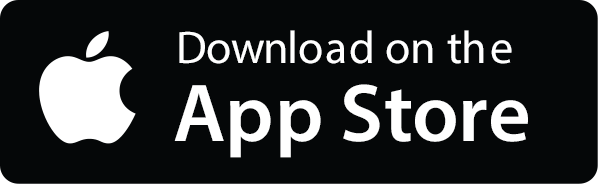
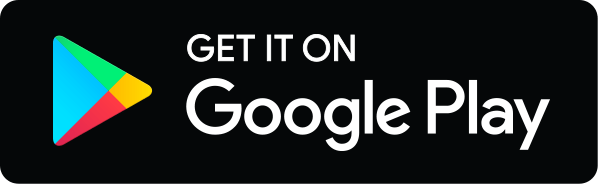