Fig. 9.1
The nuclear envelope and the nuclear lamina. The nuclear envelope provides a physical barrier for nuclear compartmentalisation and a platform for communication between the nucleus and cytoskeletal domains via a group of proteins termed the LINC complex. The outer nuclear membrane (ONM) contains the KASH domain protein nesprins, which bind to cytoplasmic filament proteins such as actin. The inner nuclear membrane (INM) contains SUN proteins and LEM domain proteins that connect the KASH domain to the nuclear lamina, consisting of lamins A, C and B. It is thought that the LINC complexes play key roles in nuclear integrity and are thought to regulate transcription, DNA damage response (DDR) and mitosis. Mutations to LINC proteins are known to cause a plethora of disease phenotypes known as laminopathies, often characterised by premature ageing or cardiomyopathy
9.1.1 Nesprins and the ONM
The ONM provides the primary interface for the anchoring of the rod-like NE spectrin repeat proteins (nesprins). Nesprins are derived from the SYNE family of genes encoding four main proteins (nesprin-1, nesprin-2, nesprin-3 and nesprin-4), which can also undergo alternate initiation and termination to produce a number of isoforms that vary in structure and function. Full-length nesprins possess a C-terminal KASH (Klarsicht/ANC-1/SYNE-1 homology) transmembrane-spanning domain, required to provide anchorage within the nuclear bilipid layer (Zhang et al. 2001; Starr and Fischer 2005). Extending into the cytoplasmic domain is a series of spectrin repeats, the number of which varies depending on the nesprin isoform. The largest proteins are nesprin-1 and nesprin-2, encoded by SYNE1 and SYNE2, which extend deep into the cytoplasmic domain with 74 and 56 spectrin repeats, respectively. Both also have N-termini containing calponin homology (CH) domains that link to cytoskeletal actin filaments (Padmakumar et al. 2004; Zhen et al. 2002). Nesprin-3 and nesprin-4 are smaller protein isoforms with fewer spectrin repeats. They differ at the N-termini compared to nesprin-1 and nesprin-2 and link to intermediate filaments such as desmin, via plectin, and microtubules, respectively (Roux et al. 2009; Wilhelmsen et al. 2005). Nesprin isoform expression is also highly cell type specific. Some nesprin-1 and nesprin-2 isoforms show highest expression in muscle tissue types (Zhang et al. 2002) and are likely to play a major role in maintaining the function and integrity of striated muscle as evidenced by their involvement in Emery-Dreifuss muscular dystrophy and cardiomyopathy (Puckelwartz et al. 2010; Zhang et al. 2007).
9.1.2 SUN Domain Proteins
The perinuclear space is occupied by the Sad1 and UNC (SUN) domain proteins, of which there are two main isoforms, SUN1 and SUN2 (Hodzic et al. 2004; Malone et al. 1999). SUN proteins form coiled-coil trimers, which are bound at their C-termini by peptides at the terminus of the nesprin KASH domain on the ONM interface of the NE. The SUN coiled-coil trimer extends across the luminal space of the NE penetrating through the INM and into the nucleoplasmic domain where the N-termini assume a globular shape and form a strong association with the nuclear lamins (Haque et al. 2006; Sosa et al. 2012).
9.1.3 Lamins and the INM
The nuclear lamina is a meshwork of proteins lining the nuclear interface of the INM, consisting of type V IF proteins known as A- and B-type lamins, which form parallel coiled-coil dimers and head-to-tail dimers to produce a large network that covers the entire INM (Ben-Harush et al. 2009). The predominant A-type lamins are lamin A and lamin C which are alternate splice products of the same gene, LMNA (Lin and Worman 1993). Unlike lamin A, lamin C is translated as a mature protein, whereas lamin A is translated as a precursor, prelamin A, which must undergo a number of modifications before becoming mature lamin A (Davies et al. 2009). B-type lamins are lamin B1 arising from the LMNB2 gene and lamins B2 and B3 from the LMNB3 gene (Harborth et al. 2001). Whilst A-type lamins are thought to be specific to differentiated cell and tissue types, B-type lamins are thought to be present ubiquitously (Broers et al. 1997). Lamins have a postulated role in the regulation of gene expression, DNA damage repair, nuclear stability and structure and heterochromatin organisation (Misteli and Scaffidi 2005). To supplement these roles, the INM also has a set of distinct membrane-associated proteins that include lamina-associated polypeptide 2 (LAP 2), emerin and MAN1 (collectively termed the LEM domain proteins) (Lin et al. 2000). Alongside lamins, these proteins are also postulated to play roles in the regulation of gene expression and particularly in the case of emerin, in maintaining the integrity of the nuclear lamina (Bengtsson and Wilson 2004).
Emerin is the 254 amino acid product of the EMD gene and is a ubiquitously expressed integral protein of the INM (Bione et al. 1994; Manilal et al. 1996; Nagano et al. 1996). Structurally, emerin contains a 220 amino acid N-terminal nucleoplasmic domain, a single transmembrane domain and a short C-terminal tail situated in the NE lumen (Cartegni et al. 1997). As well as the aforementioned roles in gene expression and structural nuclear integrity, emerin is also hypothesised to have a role in regulation of cell cycle and nuclear assembly during mitosis and has a known association with β-catenin which is thought to restrict accumulation of β-catenin in the nucleus (Markiewicz et al. 2006).
The sequential tethering of these NE spanning proteins is thought to be crucial for functions including structural stability and nuclear form, nuclear positioning, chromatin scaffolding and facilitating rapid physically mediated communication to the nucleus, from the cytoplasmic and extracellular environment via mechanotransduction and mechanosignalling.
9.1.4 Nuclear Envelopathies
The general importance of the NE is highlighted by the unique set of pathogenic conditions that arise as a result of perturbation to components of the NE termed nuclear envelopathies. These include a subset of the laminopathies (Table 9.1), which are a set of tissue-specific disorders, often affecting striated muscle tissue and/or with cardiac involvement and displaying elements of premature ageing. Research into the characteristics and mechanisms of such diseases has helped identify a major role for the NE in cardiac cytoarchitecture and disease.
Table 9.1
Known nuclear envelopathies caused by mutations to LMNA and ZMPSTE24, SYNE1, SYNE2 and EMD
Disease | Gene | Heart involvement | Reference |
---|---|---|---|
Autosomal dominant Emery-Dreifuss muscular dystrophy | LMNA, SYNE1, SYNE2 | Yes | |
Cerebellar ataxia | SYNE1 | No | Gros-Louis et al. (2007) |
Charcot-Marie-Tooth disease | LMNA | No | De Sandre-Giovannoli et al. (2002) |
Dilated cardiomyopathy | LMNA, SYNE1 | Yes | |
Familial partial lipodystrophy of the Dunnigan type FPLD | LMNA | Yes | Cao and Hegele (2000) |
Hutchinson-Gilford progeria syndrome | LMNA, ZMPSTE24 | Yes | Eriksson et al. (2003) |
Limb-girdle muscular dystrophy | LMNA | Yes | Muchir et al. (2000) |
Lipoatrophy with diabetes, hepatic steatosis, hypertrophic cardiomyopathy and leukomelanodermic papules (LDHCP) | LMNA | Yes | Caux et al. (2003) |
Mandibuloacral dysplasia with type A lipodystrophy (MADA) | LMNA | Yes | Novelli et al. (2002) |
Mandibuloacral dysplasia with type B lipodystrophy (MADB) | LMNA, ZMPSTE24 | Yes | Agarwal (2003) |
Restrictive dermopathy | ZMPSTE24 | Unknown | Navarro et al. (2004) |
X-linked Emery-Dreifuss muscular dystrophy | EMD | Yes | Bione et al. (1994) |
9.1.5 The LINC Complex in Cardiomyocytes
In the cardiomyocyte (CM), the LINC complex is postulated to mediate a physical interaction between the NE and the sarcomere. It is thought that within the cytoplasm this occurs via interactions between the actin-binding CH domain of nesprins, which can potentially bind actin of the sarcomere, but also via interactions between nesprins, plectin and desmin (Fig. 9.1). The importance of this link is twofold. Firstly, the nucleus is likely to be crucial to the structural integrity of the CM and therefore important in dealing with the perpetual mechanical stress imparted upon the cytoskeleton during contraction. Indeed, mutations in LINC complex components disproportionately affect tissues which are under mechanical strain suggesting that these links are crucial for the structural integrity of CMs. Secondly, the nucleus constantly senses the dynamic changes in the cytoplasmic (and potentially also the extracellular) domain due to mechanosignalling via the LINC complex and the cytoskeleton and consequently adjusts to such changes with appropriate gene expression responses (Meinke et al. 2014). Evidence suggests that these are the principal processes that become deregulated in disease settings, leading to the onset of cardiomyopathies.
9.2 The Role of the NE in Cardiac Disease
Cardiac abnormalities associated with NE mutations are clinically varied and include dilated cardiomyopathy (DCM), hypertrophic cardiomyopathy (HCM) and arrhythmogenic right ventricular cardiomyopathy (ARVC). Often these present in combination with other muscle phenotypes, such as limb girdle muscular dystrophy, LGMD, or more commonly Emery-Dreifuss muscular dystrophy, EDMD, reflecting the major influence of NE perturbations on mechanical tissues. EDMD is a condition in which patients experience early-onset contractures of the elbows, ankles and spine and endure subsequent humero-peroneal muscle wasting, and by adulthood, patients develop cardiomyopathy with conduction defects (Emery 1987, 1989, 2000). EDMD occurs by two primary means, both arising from components of the NE; one is described as X linked, in which mutations to the emerin gene EMD are identified as causative (Bione et al. 1994), and also autosomal dominant EDMD due to mutations in LMNA (Bonne et al. 1999). Of the NE components, LMNA mutations are currently thought to be the most common cause of NE-associated DCM. In fact, mutations in the LMNA gene are relatively commonly identified in patients with sporadic or inherited DCM and often lead to sudden cardiac death (van Berlo et al. 2005). As a result of this, LMNA is also the most rigorously studied NE protein in the context of heart disease.
HCM has also been described in conjunction with LMNA mutations. One instance involved a patient with familial partial lipodystrophy type 2 (FPLD2) caused by a missense mutation to LMNA that confers an amino acid substitution at residue 591 (Araújo-Vilar et al. 2008). This proband was also insulin resistant indicating a potentially wider role for lamins in heart disease, as perturbations to lamins may also be involved in diabetes and metabolic syndrome (Hegele 2000, 2003). Additionally, there was a case reported whereby a patient with the R644C mutation, arising in the C-terminal domain in close proximity to the prelamin A processing site, displayed pathogenicity consistent with HCM (Mercuri et al. 2005).
LMNA mutations have also been shown to be responsible for ARVC phenotypes. ARVC is characterised by pathological remodelling primarily of the right ventricle, in which myocyte death, inflammation and fibrofatty replacement of the myocardium are the main histological features (Sen-Chowdhry et al. 2010). ARVC arises primarily due to mutations in genes whose protein products contribute to the composition of the desmosomes such as desmoplakin, desmoglein, plakoglobin and plakophilin. Desmosomes are found at the plasma membrane in the intercalated disc (ID) regions where adjacent CMs join. The role of the desmosome is to tether the IFs of one cell to the cytoplasmic membrane of another to create a lattice structure that provides mechanical strength to tissue. However, a number of patients presenting with ARVC who did not have mutations to desmosomal genes were found to have LMNA mutations, suggesting that NE perturbations can influence CM cytoarchitecture at peripheral subcellular locations (Larsen et al. 2012; Quarta et al. 2011).
Mutations of the LMNA gene in humans have most frequently been shown to result in DCM leading to conduction defects resulting in arrhythmias and even leading to sudden cardiac death (Cowan et al. 2010). However, the recurrence of common DCM-causing mutations is low; the university mutation database states that there are currently 132 unique DCM-causing mutations to LMNA. As such, correlations between genotype and phenotype provide little idea on the mechanisms leading to cardiomyopathy. There are a subset of laminopathies which are caused by a few ‘hot spot’ mutations which lead to specific phenotypes and are likely to share mechanistic pathways (Scharner et al. 2010) including classical Hutchinson-Gilford progeria syndrome (HGPS), Werner syndrome, Charcot-Marie-Tooth disorder and MAD. However, the more common nuclear envelopathies, which are primarily striated muscle disorders, A-EDMD, LGMD1B and DCM, are caused by seemingly indiscriminate and promiscuous mutations which give little indication of the mechanistic milieu. For example, mutations that cause DCM have been found in all 12 exons of the LMNA gene. Moreover, mutations that cause DCM can also cause EDMD, LGMD1B and FPLD in separate individuals or can lead to any of these diseases with associated DCM. The level of pleiotropy seen with some of these mutations is unusual. For example, one curious mutation to the LMNA gene, R644C, displays extreme phenotypic diversity in the clinic (Rankin et al. 2008). These findings suggest that epigenetic or other extrinsic factors may be important in disease pathogenesis and progression. This diversity makes mechanistic analysis challenging, although some DCM-causing LMNA mutations have been investigated in order to provide some insight into the workings of LMNA-associated DCM and are discussed in a later section (Table 9.2).
Table 9.2
Phenotypic comparison of existing models of LMNA-associated DCM
Model | Survival | Heart pathology | Fibrosis | Inflammation | Cell death | Nuclear morphology defects |
---|---|---|---|---|---|---|
Lmna −/− | 6–8 weeks | DCM and heart failure | + | − | + | + |
Lmna +/− | Long-lived | Late-onset DCM-CD | + | − | + | + |
Lmna N195K/N195K | 12–14 weeks | DCM and heart failure | + | − | + | |
Lmna H222P/H222P | Males: 4–9 months Females: 9–13 months | DCM and heart failure | + | − | − | + |
Lmna G609G/G609G | 3–4 months | LQT and arrhythmia | ? | − | ? | ? |
Zmpste24 −/− | 3–4 months | Nonspecific | − | + | − | ? |
Lmna MHC-M371K | 2–7 weeks and prenatal lethal | Acute and subacute heart failure | − | − | − | + |
Lmna nPLAO/nPLAO | Males live up to 40 weeks and females live up to 80 weeks | DCM and heart failure | + | ? | ? | ? |
Regarding nesprins, the situation is also complex. Mutations to SYNE1 can lead to autosomal recessive cerebellar ataxia due to nonsense mutations causing truncation of the protein (Li et al. 2014). However, SYNE1 and SYNE2 mutations have also been identified in probands with EDMD (Zhang et al. 2007) and DCM (Puckelwartz et al. 2010), and these missense mutations occur near the C-terminus of SYNE1/2 which encode muscle-specific isoforms that bind to other LINC complex components including emerin, lamin A/C and SUN (Zhang et al. 2007), suggesting that uncoupling of the LINC complex may contribute to cardiomyocyte dysfunction.
As mentioned above, mutations to emerin lead to EDMD with associated DCM. The mutations involved lead to loss of function of emerin and correlations with phenotype suggest that a fully functional emerin is required for skeletal muscle development and maintenance, whilst also important for cardiac muscle maintenance (Bione et al. 1994; Nagano et al. 1996; Patel et al. 2014).
Mutations to SUN proteins alone have recently been putatively associated to the onset of musculoskeletal and cardiomyopathic disease (Meinke et al. 2014). They have also been identified in combination with mutations to myosin-binding protein C, lamin A/C, emerin and LAP2α, in all instances leading to EDMD-like or cardiomyopathic phenotypes (Meinke et al. 2014; Li et al. 2014). This digenic pattern of mutation accumulation, whether sporadic or heritable, is believed to have disease-modifying effects, including early onset of disease and increased disease severity (Meinke et al. 2014). Mechanistically, it was shown, in vitro, that the identified mutations to SUN proteins led to defective myonuclear positioning via nuclear-microtubule uncoupling in human and C2C12 murine myotubes (Meinke et al. 2014).
9.3 Mechanistic Insights into NE-Associated Cardiomyopathy
There are two prominent hypotheses to explain why defects in the NE lead to cardiac cell dysfunction. The first of these suggests that mutations to NE proteins lead to structural changes that have a detrimental effect on the nucleus-cytoskeleton coupling and render cells more susceptible to mechanical stress (Worman and Courvalin 2004; Worman and Bonne 2007). The second suggests that mutations in NE proteins lead to abnormal gene expression (Worman and Courvalin 2004; Worman and Bonne 2007; Capell and Collins 2006). These hypotheses are not mutually exclusive and observations have identified the deregulation of multiple cell signalling pathways and cell maintenance systems, defects to gap junctions and fibrosis of cardiac tissue in a number of in vivo mouse models, suggesting that perturbations to the NE can have a disruptive influence on tissue organisation and integrity.
9.3.1 Mechanical Efficiency and Structural Instability
The stability and structure of a cell is undoubtedly important to its function, and striated muscle cells are uniquely designed to both exert force and cope with extreme loads. At the tissue level, perturbations of the LINC complex result in histological reports of myocyte disarray (Pendás et al. 2002), whilst at the cellular level, misexpression and mislocalisation of intermediate filament proteins such as desmin (Nikolova et al. 2004), therefore disrupting the connection of the NE to the sarcomeres of the myocyte, is apparent, suggesting uncoupling of the entire structural framework of the cell. In such scenarios, it is hypothesised that muscle is unable to exert the required force or cope with sufficient loads for efficient mechanical functionality.
In vivo, signs that mechanical instability occurs in cardiac tissue as a result of NE perturbations are compelling. The first animal model developed to investigate the role of the nuclear lamina in DCM was the lamin A/C global knockout mouse. Lmna −/− mice die between 6 and 8 weeks of age because of DCM and heart failure (Nikolova et al. 2004). Phenotypically, hearts of Lmna −/− mice have irregular patterns of heart tissue histology and skeletal muscle dystrophy (Sullivan et al. 1999) and succumb to severe systolic dysfunction (Nikolova et al. 2004). Detailed cardiac characterisation has shown Lmna −/− CMs to be mechanically unsound. They have nuclear morphology defects and appear to undergo disruption of the LINC complex via nesprin1α leading to uncoupling of the nucleus from the cytoskeleton resulting in mechanical instability and defective force transmission (Nikolova-Krstevski et al. 2011). Additionally, the IF protein desmin, which serves to link the sarcolemma to the sarcomere and also the sarcomere to the nucleus, is mislocalised in these mice further supporting this hypothesis (Nikolova et al. 2004). However, Lmna −/− mice are not relevant in the clinical setting therefore a number of animal knockin models of human mutations have been developed.
The H222P-LMNA mutation causes EDMD and DCM in humans and has been investigated using Lmna H222P/H222P mice. Whilst these mice are born healthy, at 16 weeks, they display a classical DCM progression leading to heart failure and death in males at 5–9 months and in females at 7–13 months (Arimura et al. 2005). Moreover, studies of mice lacking the C-terminus and KASH domain of nesprin-1, which mimic to some extent human mutations observed in DCM, also showed a DCM-like phenotype, characterised by reduced fractional shortening, indicating reduced contractility of the myocardium (Puckelwartz et al. 2010).
Additionally, the importance of the NE for structural stability in CMs has also been highlighted by experiments performed in vitro, which showed that when DCM-causing mutations were introduced to the genes of the NE components emerin and lamin A/C, and the cells then subjected to mechanical stress, uncoupling of the nucleoskeleton from the cytoskeleton was observed (Zwerger et al. 2013). In this instance, investigators were able to show that when healthy cells are stretched at the plasma membrane, the nucleus is displaced, indicating that the nucleus, cytoskeleton and plasma membrane are mechanically linked. However, when cells with mutated lamin A/C were stretched, the nucleus was unmoved, suggesting the link between the plasma membrane and the nucleus had been broken (Zwerger et al. 2013).
9.3.2 Mechanosignalling and Gene Regulation
Mechanosignalling is the conversion of physical cues into intracellular signals (biochemical and biophysical) that mediate a cellular response to external stimuli. Indeed, research has shown that the NE plays a pivotal role in the biophysical signalling cascade, which can regulate gene transcription and elicit a physiological response (Wang et al. 2009). Furthermore, it has been suggested that the inability of the cell to convert environmental/external signals efficiently into a transcriptional response makes the cell susceptible to stress and contributes to the onset of pathogenesis, especially in the case of NE- and LINC-associated proteins (Isermann and Lammerding 2013; Jaalouk and Lammerding 2009).
There is evidence from animal models to suggest that A-type lamins play a role in mechanosignalling. In Lmna −/− mice, activation of genes appears to be attenuated, and the suggestion is that Lmna −/− mice are unable to adapt to DCM progression with compensatory hypertrophy, which accounts for the speed of disease progression and short lifespan. Moreover, it has been reported that haploinsufficiency of Lmna ameliorates pressure overload-induced hypertrophy in a mouse model of transverse aortic constriction (Cupesi et al. 2010). The mechanisms identified imply that impaired activation of mechanosensitive genes such as Egr1, which encodes the transcriptional regulator early growth response protein 1, is crucial to this phenotype. In this study, haploinsufficiency of the LMNA gene was investigated using Lmna +/− mice, which have a baseline phenotype of early-onset cardiac conduction system (CCS) disease and late-onset DCM (Wolf et al. 2008).
In vitro, single-cell models of mechanical stretch showed that mouse embryonic fibroblasts (MEFs) lacking emerin have impaired mechanotransduction associated with reduced Egr1 gene expression (Lammerding et al. 2006). Similarly, MEFs lacking Lmna experience attenuated gene expression responses to mechanical strain and cytokine stimulation via reduced nuclear factor-kappa-B (NF-κB) transcription (Lammerding et al. 2004). Moreover, Lmna-null MEFs and MEFs harbouring the N195K mutation to Lmna are known to experience impaired nuclear translocation and downstream signalling of the mechanosensitive transcription factor megakaryoblastic leukaemia 1 (MLK1), a member of the myocardin family thought to be crucial to cardiac development and function (Meinke et al. 2014). It has also been shown that the NE may be able to detect perturbations in force and convert these perturbations into adaptive or pathological gene expression responses (Lammerding et al. 2004, 2005; Lammerding and Lee 2005).
9.3.3 Intercalated Disc Disorganisation
Intercalated discs (IDs) are polarised regions of the CM plasma membrane, which serve to mechanically couple adjacent CMs to one another and facilitate the conductance of the action potential between cells. This occurs via numerous junctional complexes, which characterise the structure and function of IDs. The importance of IDs in the heart is highlighted by the discovery that mutations to ID proteins are known to lead to cardiomyopathies and arrhythmia in humans, including plakophilin (Gerull et al. 2004), desmoplakin (Sen-Chowdhry et al. 2005) and N-cadherin (Li et al. 2005). The idea that IDs might be involved in NE-mediated cardiomyopathy stems from the observation that conduction defects and arrhythmia are also frequently observed in DCM patients with NE mutations. In vivo, several lamin models, including lamin-deficient mice and mice harbouring the N195K mutation, exhibited mislocalisation and aberrant expression of connexins (Cx) 40 and 43 (Frock et al. 2012; Mounkes et al. 2005). Connexins are gap junction (GJ) proteins whose primary role is to allow the conduction of stimulatory potassium current from one CM to the next, thereby facilitating contractility and synchronous heart rhythm across the myocardium. Consequently, deregulation of these processes potentially leads to systolic dysfunction and arrhythmia phenotypes and is the primary cause of death in these models. Accordingly, postmortem analysis of cardiac tissue from patients who suffered sudden cardiac death showed significantly reduced Cx43 expression (Chen and Zhang 2006). Currently, it is not known how perturbations to the NE lead to disorganisation of GJs.
Another ID protein, β-catenin that forms part of the adherens junction (AJ) is regulated by emerin. In CMs, emerin also localises to the AJs, some distance away from the typical NE localisation. This interaction appears to be crucial to AJ organisation, because when CMs from emerin-null mice were analysed, β-catenin distribution, and subsequently ID architecture, was found to be aberrant. Loss of emerin also deregulates β-catenin signalling in CMs, which may provide a common mechanistic link between EDMD-associated DCM and ARVC phenotypes as ARVC pathogenesis has been attributed to altered Wnt/β-catenin signalling.
Interestingly, atypical localisation of LINC complex proteins in CMs has also been reported for nesprins. Nesprin-1 was shown to localise to the Z-disc of the sarcomere in human cardiac tissue (Zhang et al. 2002) indicating that mutations to nesprin-1 that lead to EDMD or cardiomyopathy could potentially cause pathogenesis by also disrupting sarcomere structure and function; however, this has not been experimentally tested as yet.
9.3.4 Autophagy and Related Signalling Mechanisms
Autophagy is a cellular housekeeping process involving the sequestration of cytoplasmic debris by double membrane vacuoles termed autophagosomes, which then fuse with lysosomes for the degradation of potentially toxic matter. It is activated in response to nutrient deprivation so that cells can scavenge amino acids for use as an emergency fuel source. In the context of the heart, autophagy has been found to protect the heart from haemodynamic stress. Activation of autophagy is reliant on complex signalling cascades, and aberrant modulation of these signalling cascades can prevent the activation of autophagic responses in circumstances in which they are required.
Evidence from models of NE-mediated cardiomyopathy suggests that NE perturbations are capable of inhibiting the autophagic response. In global Lmna −/− mice, for example, autophagy was found to be impaired (Ramos et al. 2012). Evidence suggested that this impairment was caused by hyperactivation of the Akt/mTOR signalling pathway. Subsequently, intervention with the mTOR inhibitor rapamycin significantly improved cardiac function and survival in Lmna −/− mice, suggesting a key role for mTOR signalling in LMNA-associated DCM. Additional analysis suggests a role for dual specificity phosphatase 4 (Dusp4), which is elevated in Lmna H222P/H222P mice. Conditional overexpression of Dusp4 leads to DCM similar to that of LMNA-associated DCM and mechanistically results in activation of the Akt/mTOR signalling pathway and subsequently, negatively regulates autophagy (Choi et al. 2012a), reiterating the phenomenon that was observed in Lmna −/− mice. Treatment of the Lmna H222P/H222P mice with an inhibitor of mTOR, temsirolimus, subsequently led to activation of autophagy, coupled with amelioration of cardiac decline (Choi et al. 2012b).
The proteasome, another method of protein degradation independent of autophagy, has also been implicated in regulating LMNA-mediated disease. Deletion of lysine at position 32 in the lamin A amino acid sequence is known to cause severe congenital muscular dystrophy phenotypes in humans (Quijano-Roy et al. 2008). Recapitulation of this mutation in vivo, using Lmna ΔK32/+ mice, also led to pathogenesis (Cattin et al. 2013). These mice presented with a DCM manifestation that resulted in death between 35 and 70 weeks. Mechanistically, this phenotype was characterised by dysfunction of the ubiquitin proteasome system (UPS) leading to nuclear aggregation of the toxic Δ32 lamin variant.
< div class='tao-gold-member'>
Only gold members can continue reading. Log In or Register a > to continue
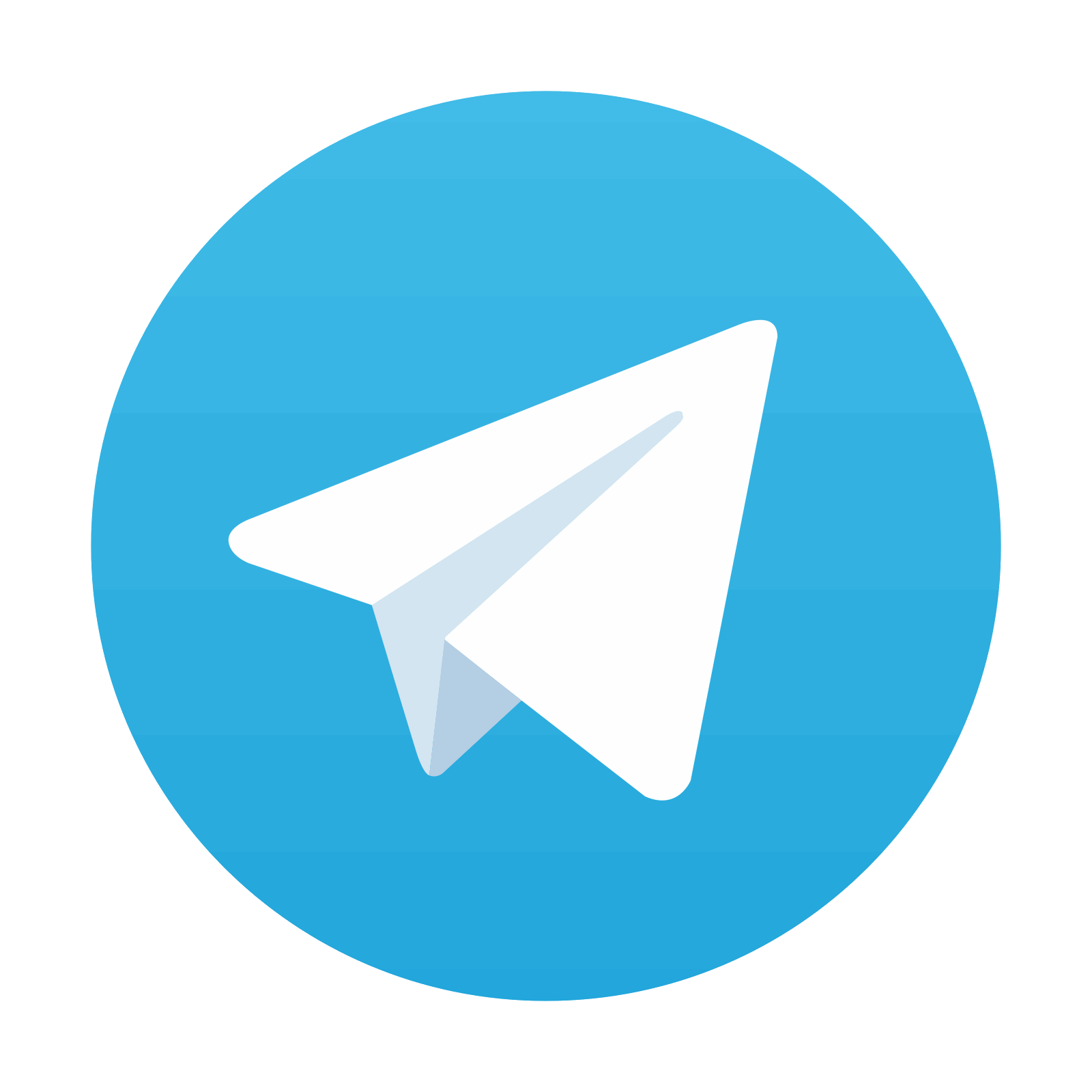
Stay updated, free articles. Join our Telegram channel

Full access? Get Clinical Tree
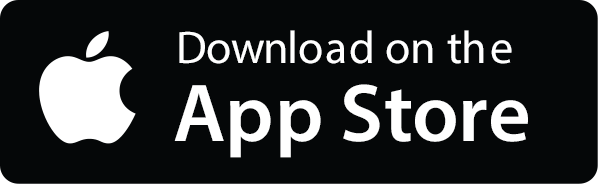
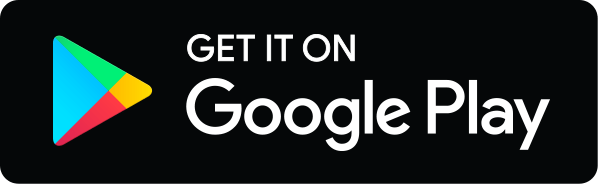