Introduction
The Context
Medical therapy of stable angina aims to address the key factor mediating myocardial ischemia: oxygen supply/demand imbalance. Pain is not the only symptom that a patient with myocardial ischemia may experience; there may also be severe fatigue, dyspnea, abdominal pain, nausea, sweating, and, occasionally, a sense of imminent death (angor animi) . Understanding cardiac pain requires knowledge of the interplay of ischemic, metabolic, and neurologic mechanisms. In this chapter, we will focus on ischemic and metabolic mechanisms and their therapy.
This chapter will not include a discussion of the clinical evaluation of a patient with stable angina, of lifestyle interventions, of antiplatelet, anticoagulant, and lipid-lowering drugs, and of revascularization strategies, all of which are dealt with in other chapters. Instead, we will focus on the narrower challenge of reducing the symptom of angina using pharmacologic agents, both traditional and novel.
Percutaneous Coronary Intervention Versus Optimal Medical Therapy for Symptomatic Ischemia
The Appropriate Use Criteria for Percutaneous Coronary Intervention (PCI) in Stable Ischemic Heart Disease recommend that patients take at least two classes of antianginal agents for symptomatic relief before PCI is considered. Despite these recommendations, many patients undergo revascularization, partly stemming from psychological and emotional elements on the part of both patients and physicians. Two groundbreaking studies, the Bypass Angioplasty Revascularization Investigation (BARI 2D) trial, and Clinical Outcomes Utilizing Revascularization and Aggressive Drug Evaluation (COURAGE) trial, compared optimal medical therapy plus revascularization versus optimal medical therapy alone to reduce mortality or major cardiovascular events.
BARI 2D compared PCI or coronary artery bypass grafting (both in addition to optimal medical therapy) versus optimal medical therapy alone in patients with type 2 diabetes mellitus. No difference was seen in 5-year mortality or in the endpoint of death between revascularization and optimal medical therapy without PCI. Likewise, the COURAGE trial, with a mean follow-up of 4.6 years, revealed no significant difference in the survival rate between optimal medical therapy and PCI for the primary event rates, death, or percentage of subjects angina-free at 5 years, nor was there any difference in survival after an extended 15-year follow-up. On the other hand, a comprehensive network meta-analysis showed that coronary artery bypass grafting or PCI with one of the new generation drug-eluting stents resulted in reductions in death, death caused by myocardial infarction (MI), and repeat revascularization, compared to medical therapy. There was no attempt to assess in these studies whether “medical treatment” was optimal medical therapy.
The ongoing ISCHEMIA (International Study of Comparative Health Effectiveness with Medical and Invasive Approaches) will also address this question. In the meantime it is reasonable to recommend that PCI or coronary artery bypass grafting be reserved for patients whose angina limits daily activity despite optimal medical therapy, which includes at least two traditional antianginal medications in maximally tolerated dosage.
Decreasing Myocardial Oxygen Demand and Increasing Oxygen Supply
Pharmacologic prevention of symptoms of angina during periods of exertion has classically involved the use of agents that reduce myocardial oxygen demand and/or increase myocardial oxygen supply in response to exercise. Importantly, all available classes of traditional antianginal agents have similar effects on exercise duration. Thus, there is no clear indication that one drug is superior to the others based on this outcome.
Management of patients with chronic stable angina has two main objectives: (1) symptomatic relief from ischemia; and (2) cardiovascular risk reduction and improvement of prognosis. These objectives are modulated by two different mechanisms: symptoms of ischemia are due to an insufficient oxygen supply/demand ratio, whereas acute coronary syndromes are due to vulnerable plaque erosion and rupture, resulting in thrombotic coronary occlusion.
Major determinants of myocardial oxygen demand are heart rate, contractility, and wall tension; minor determinants are basal metabolism and activation energy. Oxygen demand can be reduced by decreasing cardiac workload or by shifting myocardial metabolism to substrates that require less oxygen per unit of adenosine triphosphate (ATP) produced. Oxygen supply may be increased by coronary vasodilatation or by increasing the duration of myocardial perfusion during diastole by slowing the heart rate. Antianginal agents either reduce myocardial oxygen demand or increase myocardial oxygen supply to reduce symptoms of angina pectoris and signs of ischemia.
Traditional Anti-Ischemic Therapy
Traditional anti-ischemic therapy includes three antianginal agents: nitrates, β-blockers , and calcium-channel blockers (CCBs) . The traditional agents reduce anginal symptoms and prolong exercise duration and/or time to ST-segment depression on the electrocardiogram (ECG). Frequently, a combination of these drugs is necessary for symptom control, but hard data on the use of all three classes together are lacking. None of these drugs has been shown to be disease modifying: they do not change the risk of MI, sudden cardiac death, or all-cause mortality. Although patients with stable coronary heart disease and preserved left ventricular function on optimal medical therapy may not have symptomatic relief with the addition of an angiotensin converting enzyme (ACE) inhibitor, there is evidence that ACE inhibitors reduce the rates of death from cardiovascular causes, MI, and coronary revascularization. Thus, we will be discussing ACE inhibitors as part of the traditional antianginal therapies.
Although efficacious, traditional anti-ischemic agents do not produce relief in all patients, and individual variation in responsiveness is well known. The combination of β-blockers with nitrates is favored because both agents lower myocardial oxygen demand and increase subendocardial blood flow through different mechanisms. The β-blockers prevent potential reflex tachycardia from nitrate-induced hypotension. In addition, by slowing the heart rate, β-blockers increase the duration of myocardial perfusion during diastole. Nitrates are vasodilators, thus they increase coronary perfusion and blunt any rise in left ventricular preload and end-diastolic pressure caused by the negative inotropic action of the β-blockers. β-Blockers combined with dihydropyridine CCBs improve exercise duration more than either alone and tolerance is usually acceptable, but the combination of β-blockers with nondihydropyridine CCBs, such as verapamil and diltiazem, should be used with caution, and is contraindicated if there is left ventricular dysfunction or significant conduction system disease.
Novel Anti-Ischemic Therapy
Unfortunately, many patients with chronic angina remain symptomatic despite receiving the combination of two or more conventional drugs (β-blockers, nitrates, CCBs) at maximum tolerated dosages. Many patients with chronic angina also have concomitant comorbidities that make it difficult to up-titrate the dose or that contraindicate use of conventional antianginal drugs due to the fear of inducing dose-related adverse effects such as hypotension, bradycardia, or atrioventricular (AV) block. In addition, persistent angina occurs in approximately 10% to 25% of patients subjected to coronary bypass surgery and/or percutaneous interventions, and 60% to 80% require antianginal therapy 1 year after the procedure.
Novel antianginal medications include the following: (1) ranolazine , a drug that reduces myocardial ischemia by its action on the late sodium current; (2) ivabradine , a selective, heart rate slowing drug that inhibits the I f current in pacemaker cells of the sinoatrial node; (3) nicorandil , a nicotinamide-nitrate ester that acts as an ATP-sensitive potassium channel opener, with nitric oxide donor capacity and antianginal properties that lead to direct coronary vasodilatation; (4) trimetazidine , a drug that inhibits β-oxidation of fatty acids, increases myocardial glucose utilization, and prevents reduction in ATP and phosphocreatine levels in response to hypoxia or ischemia; and (5) molsidomine , a drug that dilates venous capacitance vessels (reducing preload), dilates coronary arterial segments, exerts a platelet stabilizing effect by inhibiting thromboxane, and has a nitric oxide-donating effect.
These drugs have considerable potential as adjunctive therapy for angina, particularly in patients who are refractory to standard therapies, and they may be a primary therapeutic option in certain circumstances because they generally do not adversely affect blood pressure, pulse rate, or left ventricular systolic function. Thus, they offer an advantage in patients in whom conventional agents may induce symptomatic hypotension, inappropriate bradycardia, or worsening heart failure (HF). The clinical utility of each of these will be discussed later in this chapter.
Investigational Anti-Ischemic Therapy
Because of the inability of current antianginal drugs to optimally control chronic angina in all cases, such as in patients with severe coronary artery disease not amenable to revascularization, or with high risk of death and repeated hospital admissions, there is an unmet need for new drugs with different, but complementary, mechanisms of action devoid of the limitations of current treatments (with no or minimal hemodynamic effects) and that can be safely added to current therapy. In this chapter, in addition to addressing some of the newest prescribable antianginal drugs, we will briefly review drugs currently under development for the treatment of chronic angina.
Do Antianginal Drugs Prevent Coronary Events?
The choice of disease-modifying treatments that influence prognosis raises a few problems. The COURAGE study clearly demonstrated that all of these treatments should be used at the maximum tolerated doses, closest to those that have been proven to be effective in improving prognosis. As the population ages patients with stable angina will have an increasing number of coexisting diseases, such as hypertension, diabetes, and dyslipidemia, which predispose to acute coronary syndromes. Different mechanisms of action for the anti-ischemic drugs allow treatment to be targeted to the individual patient, dependent on comorbidities and cardiac function. For example, in the absence of contraindications, β-blockers remain the reference treatment to prevent angina attacks and for the secondary prevention of MI. The most common coexisting cardiovascular risk factor is hypertension and, therefore, dihydropyridine CCBs have a role in combined treatment with β-blockers.
A combination of anti-ischemic drugs might improve the benefit of treatment with an additive or even synergistic effect. The findings of the Heart Outcomes Prevention Evaluation (HOPE) and EURopean trial On reduction of cardiac events with Perindopril in stable coronary Artery disease (EUROPA), discussed hereafter, support the use of an ACE inhibitor for the prevention of cardiovascular complications in all high-risk patients, including those with stable angina.
In all cases, the importance of risk profiling, management of comorbidities that increase the risk of cardiovascular events, such as hypertension, diabetes, and dyslipidemia, and aggressive lifestyle modification in all patients cannot be overstated.
Traditional Anti-Ischemic Therapy
Nitrates
Mechanism of Action
Endogenous nitric oxide (NO) production is mediated by the enzyme nitric oxide synthase (NOS). In coronary artery disease, NO generation by NOS may be inhibited by ischemia, because NOS activity requires the availability of oxygen. Nitrates are antianginal agents by virtue of their biotransformation to NO. Nitrates, by generating NO, are coronary vasodilators, reduce preload by venodilatation, and are arteriolar dilators. The arteriolar dilatation affects the arterial wave reflection from the periphery back to the aorta so that there is lowering of left ventricular afterload. Accordingly, aortic systolic pressure is reduced with little or no decrease in brachial systolic pressure. However, nitrates are better venous than arteriolar dilators probably because of reflex activation of the sympathetic nervous system, which limits arteriolar dilatation.
In experimental studies, NO also possesses antiatherogenic actions, reducing endothelial cell–leukocyte interaction, smooth muscle proliferation, and platelet adherence and aggregation ( Fig. 20.1 ). In one study, long-term supplementation with L-arginine, a precursor of NO, improved small-vessel coronary endothelial function in humans.

The most commonly used nitrates are nitroglycerin (glyceryl trinitrate [GTN]), isosorbide dinitrate (IDN), and isosorbide-5-mononitrate (IMN). These may be available as sublingual, oral, sustained oral, buccal, oral spray, transdermal patch, and intravenous formulations. Exogenous nitrates are organic prodrugs that undergo enzymatic bioactivation to vasoactive NO in several steps. GTN produces NO via the action of mitochondrial aldehyde dehydrogenase-2 (mtALDH-2). IDN undergoes hepatic biotransformation to IMN. IMN, bypassing the miALDH-2 step, forms NO. NO relaxes vascular smooth muscle by activating guanylyl cyclase, leading to an increase in cyclic guanosine monophosphate (cGMP), which inhibits calcium entry into smooth muscle cells, causing vasorelaxation. NO also relaxes smooth muscle cells by inhibiting potassium channels and hyperpolarizing the membrane.
Endothelial dysfunction decreases production (or enhances inactivation) of NO. Organic nitrates, by their conversion to NO, have the ability to replenish deficient levels of NO in patients with coronary artery disease. In these patients, exogenous nitrate administration substitutes for the impaired activity of the endothelial L-arginine/NO pathway.
Pharmacokinetics
Sublingual GTN is the nitrate of choice for acute angina attacks. GTN is rapidly absorbed from mucus membranes, skin, and the gastrointestinal tract and has a plasma half-life of only a few minutes. GTN is metabolized by a liver reductase enzyme to biologically active glyceryl dinitrate and biologically inactive glyceryl mononitrate. The elimination half-life of glyceryl dinitrate is 10-fold longer than the parent GTN, which may account for the antianginal effect lasting more than a few minutes.
Isosorbide mononitrate is bioavailable without any hepatic metabolism, whereas isosorbide dinitrate (IDN) needs to be converted in the liver to active mononitrates such as isosorbide mononitrate (IMN), with a half-life of 4 to 6 hours. IDN may also be given sublingually, but because IDN needs to undergo hepatic bioactivation, the onset of antianginal action is slower than for GTN. IDN and IMN are usually prescribed for daily oral administration for angina prophylaxis, but the development of tolerance is a significant problem.
Drug-Drug Interactions
The most important interaction is with the selective phosphodiesterase (PDE)-5 inhibitors, such as sildenafil, tadalafil, and vardenafil, which inhibit the degradation of cGMP and are used for the therapy of erectile dysfunction and sometimes for pulmonary arterial hypertension. The combination of nitrates and a PDE-5 inhibitor can cause serious hypotensive reactions and should not be taken on the same day. Alcohol and other vasodilators, including some antihypertensive drugs (eg, CCBs, α-blockers, hydralazine, minoxidil) may augment hypotension.
Side Effects
Common side effects include headaches, facial flushing, hypotension and syncope, and tachycardia. In hypertrophic cardiomyopathy, nitrates may exaggerate left ventricular outflow obstruction. Reduced venous return due to venous dilatation may compromise cardiac output in acute coronary syndromes, hypertrophic cardiomyopathy, constrictive pericarditis, and tight mitral or aortic stenosis.
Hemodynamics
Nitrates alleviate anginal symptoms by increasing myocardial oxygen supply and reducing oxygen demand. Increasing oxygen supply is achieved by (1) coronary vasodilatation; (2) venodilatation, thereby reducing cardiac filling pressure, lowering left ventricular diastolic pressure, and thus allowing for increased subendocardial myocardial perfusion; (3) preventing coronary artery vasospasm; and (4) replacing endogenous NO in vascular smooth muscle cells exhibiting endothelial dysfunction. Decreased myocardial oxygen demand occurs by (1) dilatation of venous capacitance vessels, reducing preload, and resulting in diminished systolic wall stress; and (2) reduction in systolic wall stress by reducing left ventricular afterload.
Organic nitrates exert their maximal vasodilatory effect at the level of venous capacitance vessels. Large and medium-sized coronary arteries and their collateral vessels are affected, whereas arterioles with a diameter of less than 100 mm are relatively less affected. The vasodilatory effect on epicardial coronary arteries with or without atherosclerotic coronary artery disease helps relieve coronary vasospasm.
Antiaggregant Action of Nitrates
GTN exerts limited antithrombotic and antiplatelet effects in patients with stable angina, by an action on platelet cGMP. NO is a potent activator of platelet guanylyl cyclase, which increases platelet cGMP levels and leads to decreased fibrinogen binding to the glycoprotein IIb/IIIa receptor. Fibrinogen binding is essential for platelet aggregation, and its inhibition leads to impairment of platelet function.
Ischemic Preconditioning
The term ischemic preconditioning describes a protective phenotype characterized by reduced sensitivity to ischemia and reperfusion injury following previous episodes of ischemia. Several lines of evidence from animal and human studies have demonstrated that the administration of GTN is associated with an increased ischemic threshold, as manifested by reduced infarct size, reduced ECG changes in the setting of percutaneous angioplasty, and reduced endothelial dysfunction after ischemia and reperfusion.
Indications
Short-acting nitrates are often given to relieve acute anginal pain and can also be used prophylactically to improve exercise tolerance and prevent exercise-induced ischemia. The use of prophylactic GTN may be particularly appropriate for patients with predictable angina precipitated by exertion or specific activities. Short-acting nitrates may also be used to supplement long-acting nitrates when patients experience acute attacks. Long-acting nitrates, either as monotherapy or in combination with β-blockers or CCBs, are used to prevent or reduce the frequency of angina in patients with coronary artery disease.
Some physicians recommend short-acting nitrates if angina occurs only a few times per week and long-acting nitrates if angina is more frequent. In patients who have angina with exertion, long-acting nitrates are sometimes preferred to prevent symptoms throughout the day. It is well known that continuous treatment with organic nitrates can lead to the development of tolerance, with loss of clinical efficacy. Tolerance can be reduced by nitrate-free intervals of 10–12 hours; therefore, it is not clinically feasible to provide continuous antianginal prophylaxis with any of the currently available long-acting nitrates.
Dosage
Three different nitrates—GTN, IMN, IDN—are available in a variety of formulations including sublingual, buccal, oral, spray, ointment, and transdermal preparations. Short-acting nitrates are available as sublingual/buccal preparations, whereas long-acting preparations include oral-sustained forms and slow-release transdermal patches and ointments, which increase their duration of action ( Table 20.1 ).
Nitrates | Routes of Administration | Dosages | Frequency |
---|---|---|---|
| Sublingual tablet | 0.15–0.6 mg | As needed |
Sublingual spray | 0.4 mg | As needed | |
Intravenous | 5–400 μg/min | As needed | |
Sublingual tablet | 0.3–0.6 mg | As needed | |
Ointment (topical) 2% | 0.5–2 in (7.5–30 mg) | As needed | |
Patch | 1 Patch (2.5–15 mg) | 2 × daily | |
| Sublingual/oral tablet | 10–40 mg | 2 × daily |
Sustained release | 30–120 mg | 1 × daily | |
Sustained release | 5–20 mg | 2 × daily | |
| Sublingual/oral tablet | 2.5–10 mg | Every 2–3 h |
Sustained release tablet | 5–40 mg | 2–3 × daily | |
Sustained release tablet | 40–160 mg | 2 × daily | |
| Sublingual tablet | 5–10 mg | As needed |
Oral tablet | 10 mg | 3 × daily |
Among short-acting nitrates, sublingual GTN tablets are the standard initial therapy for effort-induced angina in the United States, whereas sublingual nitroglycerin spray is favored in most European countries as the preferred short-acting formulation. Sublingual GTN can be administered by metered dose spray that dispenses 0.4 mg of the drug. One to two sprays can be used at the start of an attack and up to three sprays can be used in a 15-minute period.
The onset of action of transdermal patches of nitroglycerin is 30 minutes, with duration of action of 8 to 14 hours. The transdermal patch comes in several sizes: each cm 2 of applied system delivers approximately 0.02 mg of nitroglycerin per hour. Thus, the 5-, 10-, 15-, 20-, 30-, and 40-cm 2 systems deliver approximately 0.1, 0.2, 0.3, 0.4, 0.6, and 0.8 mg of nitroglycerin per hour, respectively. The remainder of the nitroglycerin in each system serves as a reservoir and is not delivered in normal use. After 12 hours, for example, each system has delivered approximately only 6% of its original content of nitroglycerin.
Nitrate Resistance and Tolerance
Although acute treatment, including sublingual administration, is immediate and effective, the benefit of nitroglycerin has been limited because of in vivo tolerance that rapidly develops on continuous treatment. Nitroglycerin tolerance is manifested as a reduced vasodilatory response and the requirement of higher doses of the drug after continuous treatment. Tolerance is a major limiting factor in the use of nitrates. Traditionally, the pathogenesis of tolerance has been described as a result of metabolic or functional effects. The metabolic effect of organic nitrates declines during continuous use as a result of decreased biotransformation to NO or decreased activity of NO released by this process (end-organ tolerance). Functional effects consisting of counter-regulatory mechanisms to NO (called “pseudotolerance”) may occur in response to nitrate therapy. For example, neurohormonal activation and plasma volume expansion may counterbalance or vitiate the therapeutic effects of nitrates.
Several postulated mechanisms underlie nitrate tolerance :
- 1.
Nitrate resistance is sometimes associated with incremental impairment of endothelial function, largely due to generation of the superoxide anion (O 2 − ) free radicals. Chronic continuous in vivo exposure to high doses of GTN sometimes induces incremental O 2 − generation with resulting evidence of redox stress. Prolonged nitrate therapy may result in the formation of peroxynitrite, which inhibits endothelial NOS, thus decreasing NO formation.
- 2.
True nitrate tolerance
- (a)
Impaired nitrate bioactivation. The activation of GTN is catalyzed by mitochondrial aldehyde dehydrogenase-2 (mtALDH-2) and, conversely, nitroglycerin can inactivate mtALDH-2. Nitrate tolerance may therefore reflect progressive inhibition of mtALDH-2 activity. Bioactivation of GTN also requires thiols or sulfhydryl-containing compounds. Interactions between GTN and sulfhydryl-containing receptors are necessary for vascular smooth muscle relaxation, and repeated administration of GTN results in sulfhydryl depletion and the development of tolerance.
- (b)
Formation of aldehydes. Aldehydes are highly toxic compounds that generate reactive oxygen species (ROS), and are normally restricted by the action of ALDH. Because organic nitrates inactivate ALDH, including mtALDH-2, excessive production of ROS results, leading to endothelial dysfunction.
- (c)
Other enzymes have been implicated in the vascular metabolism of GTN and other organic nitrates, including glutathione-S-transferase (GST), cytochrome P3A, other cytochrome P450 isoforms, and xanthine oxidoreductase (XOR).
- (d)
Reduced bioactivity of NOS. Clear evidence now exists that GTN therapy has negative effects on the function of NOS, the enzyme responsible for the endothelial control of vascular tone. GTN therapy appears to induce a dysfunctional state of NOS, in which the reductive activation of molecular oxygen to form O 2 − is not followed by oxidation of L-arginine and NO synthesis, resulting in the net generation of “NOS uncoupling.” Uncoupling can be triggered by reduced bioavailability of tetrahydrobiopterin and/or L-arginine, cofactor and substrate, respectively, for NOS.
- (e)
Formation of peroxynitrite. As an unstable structural isomer of nitrate, NO 3 − , peroxynitrite is a powerful oxidant that may influence nitrate resistance. Increased concentrations of peroxynitrite have been associated with prolonged nitrate therapy and may destabilize the metabolite isomer of nitroglycerin. This inhibits endothelial NOS, decreasing NO formation.
- (a)
Strategies to Reduce Nitrate Tolerance
Various measures have been tried to prevent nitrate tolerance. The only effective approach to mitigate tolerance has been the use of “interval dosing” or “intermittent therapy,” that is, dosing regimens or nitrate formulations that yield low or nitrate-free plasma concentrations for 10–12 hours per day. One example is to prescribe 12-hour intervals of isosorbide mononitrate instead of the standard 7 hours, or to recommend nitrate-free days. This approach, however, carries a risk for increased angina episodes during nitrate-free periods and precludes a continuous therapeutic effect.
There have been some studies, either in animals or in small numbers of humans, which suggest other strategies for managing nitrate tolerance. Some human studies have suggested that vitamin E, vitamin C, and statins are effective. In animal studies, pravastatin and atorvastatin prevented nitrate tolerance and vascular superoxide formation induced by subcutaneous GTN injections, an effect associated with increased basal cGMP levels that was abolished when rats received an inhibitor of eNOS concomitantly with GTN ( Table 20.2 ).
Agent | Potential Mechanism(S) of Action |
---|---|
ACE inhibitors | Prevention of NAD(P)H oxidase activation |
Hydralazine | Inhibition of NAD(P)H oxidase |
Ascorbic acid | Limitation of oxidative stress |
Folic acid | Re-coupling of NO synthase |
L-Arginine | Increased NO generation |
N -Acetylcysteine |
|
Folic acid can reverse endothelial dysfunction, possibly by restoring the bioavailability of tetrahydrobiopterin (a cofactor for NO synthase), arginine (its substrate), or both. This observation suggests a possible role of folic acid in preventing nitrate tolerance.
Treatment for 5 to 10 days with L-arginine, the substrate for NO synthesis, can modify or prevent development of nitrate tolerance during continuous transdermal GTN use.
Hydralazine may attenuate nitrate tolerance, perhaps by preventing superoxide generation. This relationship could contribute to the efficacy of combined nitrate-hydralazine therapy in patients with HF. Hydralazine is a vasodilator that also may overcome the effect of the formation of free radicals; indeed, the combination of nitrates and hydralazine has an established place in the treatment of HF. In patients with angina pectoris, a β-blocker should be given in combination with hydralazine because of reflex sympathetic activation.
Clinical Utility of Nitrates
- 1.
Short-acting nitrates for acute effort angina: Sublingual GTN 0.15 to 0.6 mg every 5 minutes until there is pain relief, up to a maximum of four to five tablets. Side effects are headaches and postural hypotension. Sublingual IDN 2.5 to 10 mg every 2 to 3 hours is recommended only if the patient is unresponsive to or intolerant of sublingual GTN. This is because dinitrate requires hepatic conversion to the active mononitrate, so the onset of antianginal action is slower (3–4 minutes) than with GTN, but the antianginal effects, however, last for up to 1 hour.
- 2.
Long-acting nitrates for angina prophylaxis: Oral sustained release IDN is used at an initial dose 5–40 mg twice daily or three times daily, with a maintenance dose of 10–40 mg twice daily or three times daily. Tolerance is a major limiting factor, and several strategies have been proposed to limit tolerance, including interval dosing, and dosing-free days. Oral sustained release IMN carries similar dosage, indications, and effects as isosorbide dinitrate. Again, tolerance can be minimized by eccentric (twice daily or three times daily) dosing, or by the use of a slow-release preparation (Imdur), initial dose 30–60 mg daily, titrating up to 120 mg daily over 7 days. Rarely, 240 mg daily may be required. Transdermal nitroglycerin patches permit the timed release of GTN over a 24-h period, but have not been shown to be superior to oral preparations.
Side Effects of Nitrates
Common side effects are hypotension, syncope, tachycardia, headache, and facial flushing. Less common is methemoglobinemia, treated with intravenous methylene blue. Contraindications include hypertrophic cardiomyopathy, where nitrates may increase outflow tract obstruction, constrictive pericarditis (because nitrates are potent venodilators and will reduce cardiac filling pressures), and concomitant administration on the same day with selective phosphodiesterase inhibitors (PDE-5) such as sildenafil, tadalafil, and vardenafil. A systolic blood pressure of less than 90 mm Hg is also a contraindication.
Conclusion
Organic nitrates, such as GTN, IMN, and IDN, when given acutely, have potent vasodilator effects, improving angina symptoms in patients with stable coronary artery disease. The mechanisms underlying vasodilation include intracellular bioactivation of the nitrates (IDN to IMN in the liver, GTN by vascular mitochondrial aldehyde dehydrogenase), the release of NO, and activation of the enzyme soluble guanylyl cyclase. Increasing cyclic guanosine-3′,5′-monophosphate (cGMP) leads to an activation of the cGMP-dependent kinase I, thereby causing the relaxation of the vascular smooth muscle by decreasing intracellular calcium concentration. The hemodynamic and anti-ischemic effects of organic nitrates are rapidly lost upon long-term (low-dose) administration due to the rapid development of tolerance and endothelial dysfunction, which in most cases is linked to impaired bioactivation by miALDH-2 and intracellular oxidative stress.
β-Blockers
Introduction
β-Adrenergic receptors are G-protein coupled transmembrane proteins. Their main antianginal action lies in the intracellular part of the β-receptor that is coupled to the G-protein complex: G s (stimulatory) and G i (inhibitory). Agonists bind to the ligand site and stimulate the β-adrenergic receptors (β 1 , β 2 , and β 3 subtypes). This binding triggers adenylyl cyclase, another transmembrane enzyme, to convert adenosine triphosphate to cyclic adenosine monophosphate, which acts as a second messenger, initiating a cascade of variable events that are organ dependent. By interacting mainly with G s , β 1 -adrenoreceptor activation of adenylyl cyclase mediates the positive inotropic and chronotropic functions in cardiac myocytes. β 2 -Adrenoreceptors interact with cardiomyocyte G s and G i ; one of the G i protein pathways may mediate the antiapoptotic effect of β 2 -adrenoreceptors. β 3 -Adrenoreceptors couple with G i only and activate NO, but not via the adenylyl cyclase pathway, to reduce vascular tone ( Table 20.3 ).
Activation | Selective Blockers | Non-Selective Blockers | |||
---|---|---|---|---|---|
β 1 | β 2 | β 1 | β 2 | β 1 , β 2 | α 1 , β 1 , β 2 (Vasodilators) |
|
|
| None |
|
|
Mechanism of Action and Pharmacology
β-Blockers exert several cardioprotective effects. Despite the action of β 1 inhibition in blocking adrenergically mediated coronary vasodilation, thus potentially reducing coronary blood flow, β 1 inhibition is most important for angina management. However, because nearly all of myocardial perfusion occurs in diastole, the slower heart rate with its longer diastolic filling time actually improves myocardial blood flow. In addition, by reducing cardiac output and by inhibiting renin release from juxtaglomerular cells, β-blockers lower blood pressure, thus reducing systolic wall stress.
β 1 -Selective blockers become less selective at higher doses and may begin to block β 2 -adrenoreceptors in the tracheobronchial tree causing bronchospasm. Therefore, β-blockers, even β 1 -selective blockers, are contraindicated in patients with bronchial asthma. However, at conventional doses, selective β 1 -receptor blockers are not contraindicated in patients with chronic obstructive pulmonary disease (COPD) unless there is a significant bronchospastic component. Furthermore, β 1 -selective blockers should be used with care in patients with peripheral arterial disease, as described hereafter.
The vasodilator activity of some of the newer-generation β-blockers is achieved through two pathways: either combined β- and α-adrenergic receptor blockade (eg, labetalol and carvedilol) or direct vasodilator activity through the activation of NOS and the release of NO (eg, nebivolol). Carvedilol may also ameliorate myocardial ischemic effects through inhibition of monocyte adhesion to the endothelium, by scavenging oxygen-free radicals, protection of endothelial function, direct vasodilation, and inhibition of oxidation of low-density lipoprotein, thus slowing the development of atherosclerotic plaques. Nebivolol exhibits greater selectivity for β 1 -adrenergic receptors than other β-blockers. The NO potentiating, vasodilatory effect of nebivolol is unique among β-blockers and, at doses below 10 mg/d, does not inhibit the increase in heart rate normally seen with exercise.
β-Blockers can also be classified according to their lipophilicity and hydrophilicity. Lipid-soluble β-blockers (eg, propranolol, timolol, metoprolol, oxprenolol) are absorbed completely by the small intestine, metabolized by the liver, and can penetrate the blood-brain barrier. Water-soluble β-blockers (eg, atenolol, sotalol, and nadolol) are absorbed incompletely through the gut and cleared by the kidneys. There are β-blockers (eg, betaxolol, bisoprolol, and pindolol) that are cleared partly by the liver and partly by the kidney. Some of the central nervous system side effects of β-blockers, such as depression and fatigue, have been linked to lipophilicity, but evidence for this is sparse.
Adverse Effects of β-Blockers
β-Blockers can increase insulin resistance and predispose patients to incident diabetes. In a network meta-analysis of 22 clinical trials with 143,153 participants who did not have diabetes at randomization, the risk of new-onset diabetes was most pronounced with diuretics and β-blockers (more so than with other classes of antihypertensive agents), implying a negative metabolic effect by the β-blockers. β-Blockers may contribute to the development of diabetes by weight gain (as much as 1.2 kg in one study), attenuation of the β-receptor mediated release of insulin from pancreatic β-cells, and decreased blood flow through microcirculation in skeletal muscle tissue, which results in decreased insulin sensitivity.
There may also be worsening by some, but not all, β-blockers of glycemic control in patients with established diabetes. In the GEMINI (Glycemic Effects in Diabetes Mellitus Carvedilol-Metoprolol Comparison in Hypertensives) trial, diabetics newly treated with metoprolol had an increase in hemoglobin (Hgb) A1c, whereas treatment with carvedilol did not elevate Hgb A1c. This finding suggests not all β-blockers have an equally adverse effect on diabetes control. A caution in diabetic patients is that β-blockers may mask the tachycardia that signals hypoglycemia.
Exercise endurance depends, in part, on a properly functioning sympathetic nervous system. β-Blockers may hamper exercise tolerance by antagonizing this effect. β-Blockers may also worsen depressive symptoms and induce sexual dysfunction. These drugs have been associated with Raynaud’s phenomenon and aggravation of peripheral arterial disease. β 2 -blockade inhibits the vasodilating effects of catecholamines in peripheral blood vessels and leaves the constrictor (α-adrenergic) receptors unopposed, thereby enhancing vasoconstriction. Severe peripheral arterial disease, with claudication or physical signs of poor peripheral perfusion, should be regarded as a contraindication to the use of β-blockers.
Abrupt withdrawal of β-blockers after prolonged administration can result in increased total ischemic activity in patients with chronic stable angina. Chronic β-blocker therapy can be safely discontinued by slowly withdrawing the drug in a stepwise manner over the course of 2 to 3 weeks. If abrupt withdrawal of β-blockers is required, patients should be instructed to reduce exertion and manage angina episodes with sublingual nitroglycerin, substituting a calcium antagonist, or using both agents in combination.
β-Blockers should not be prescribed for patients with bradycardia associated with third-degree heart block or sick-sinus syndrome or in untreated pheochromocytoma. They may mask the clinical signs of hyperthyroidism and precipitate thyroid storm with abrupt discontinuation.
The most common comorbid conditions cited for withholding β-blockers in elderly patients after myocardial ischemia are COPD and asthma. The safety of β-blockers in COPD patients who do not have significant bronchospasm has been demonstrated, but their use in patients who have COPD and angina remains low. Patients diagnosed with COPD tend to be treated with inhalers despite lack of objective evidence of bronchospasm, including pulmonary function testing. Patients should not be denied the benefits of β-blockers if they do not have significant bronchospasm. It may be necessary to discontinue the drug in a few patients because of bronchoconstriction, but, from the published literature, the potential benefit appears large enough to warrant taking this small risk. Metoprolol, a cardioselective β-blocker with a short half-life, has been shown to be safe and effective in patients with COPD and may be the β-blocker of choice for initiating therapy.
β-Blockers and Cardioprotection
The use of β-blockers is recommended in patients with stable ischemic heart disease (SIHD) and reduced ejection fraction and/or history of myocardial ischemia or acute coronary syndrome within the last 3 years.
The role of β-blockers in the management of patients with HF with reduced ejection fraction is well established. The Metoprolol CR/XL Randomized Intervention Trial in Heart Failure (MERIT-HF), in patients with New York Heart Association (NYHA) class II to IV HF, showed a 34% reduction in mortality in patients treated with metoprolol succinate versus placebo. The CarvedilOl ProspEctive RaNdomIzed CUmulative Survival (COPERNICUS) trial included patients with a left ventricular ejection fraction (LVEF) of less than 25%. Compared with placebo, carvedilol reduced mortality risk at 12 months by 38% and the risk of death or hospitalization for HF by 31%.
Another longer-acting β-blocker, bisoprolol, showed similar long-term benefit on survival in patients with HF. The Cardiac Insufficiency Bisoprolol Study (CIBIS-II) showed a 32% reduction in all-cause mortality in bisoprolol-treated patients with NYHA class III or IV HF caused by ischemic and nonischemic cardiomyopathy.
Although all three of these agents (metoprolol, carvedilol, and bisoprolol) are beneficial in patients with HF, the Carvedilol or Metoprolol European Trial (COMET) demonstrated a 17% greater mortality reduction in favor of carvedilol compared with metoprolol XL, with mean daily doses of 85 and 42 mg/d, respectively. As a result of these studies, β-blockers, particularly metoprolol, carvedilol, and bisoprolol, are recommended for the long-term management of patients with angina and left ventricle (LV) systolic dysfunction.
On the other hand, there is insufficient evidence to support β-blockers to prevent coronary events in patients with normal ejection fraction and no history of symptomatic myocardial ischemia. Patients with ischemic heart disease from the Reduction of Atherothrombosis for Continued Health (REACH) registry ( n = 21,860) were divided into three cohorts: prior MI, coronary artery disease (CAD) without MI, or those with CAD risk factors only. The study showed no significant difference in the primary composite outcome of cardiovascular death, nonfatal MI, and nonfatal stroke in patients on β-blockers versus those not on β-blockers for all three cohorts. Only in those with recent MI (≤1 year), was β-blocker use associated with a lower incidence of the secondary outcome, defined as the primary outcome plus hospitalization for atherothrombotic events or a revascularization procedure.
β-Blockers and Atrial Fibrillation
In atrial fibrillation (AF) a rapid ventricular rate may be associated with angina. β-Blockers are well established as useful and effective agents for rate control in patients with AF. Whereas β-blockers have been shown to improve cardiovascular outcomes in HF with reduced ejection fraction, this action seems to be attenuated in the presence of AF. In a meta-analysis of four studies that enrolled patients with HF, 1677 of them with AF, half were treated with β-blocker, and half with placebo. In patients with sinus rhythm there was a significant reduction in mortality and HF hospitalizations, but not in those with AF. In addition, whereas β-blockers predominantly slow AV conduction, they do not exhibit specific antifibrillation properties in the atria. Thus, there is no significant effect of acute administration of a β-blocker in eliciting the conversion of AF or flutter to sinus rhythm.
Conclusion
The anti-ischemic and antiarrhythmic activities of β-blockers have made this class of agents, together with nitrates, the mainstay of angina therapy. Generally, β 1 -selective blockers are preferred. Examples are metoprolol tartrate (short acting) or succinate (long acting), and bisoprolol. Carvedilol, a β-blocker with α-antagonist activity, and nebivolol, a β-blocker that also generates NO, add to the established benefits of this exceptional class of drugs in ameliorating angina. β-Blockers remain the standard of care after episodes of myocardial ischemia and in patients with HF with reduced ejection fraction. However, other than their antianginal and antihypertensive actions, there is a scarcity of evidence to support the use of β-blockers in patients with chronic ischemic heart disease who have a normal ejection fraction. None of the relevant studies have demonstrated a prognostic advantage of β-blockers over other antianginal drugs for preventing adverse cardiovascular events. Future studies should assess the use of β-blockers combined with other therapies (ie, antiplatelets, statins, nitrates, additional classes of antihypertensive medications, and PCI) in these patients.
Calcium-Channel Blockers
Introduction
The actions of CCBs on coronary blood flow are complex. The change in blood flow that they induce depends on their actions at rest versus during exercise, effects on coronary perfusion pressure, variable changes in myocardial oxygen demand, autoregulation of coronary flow, and differential effects on diastolic perfusion time.
Classification
Based on their chemical structure, CCBs fall into the following groups ( Table 20.4 ):
- 1.
Dihydropyridines (eg, amlodipine, nifedipine, nicardipine, felodipine). These act mainly on arterial vascular smooth muscle cells to dilate vessels, consequently lowering blood pressure.
- 2.
Nondihydropyridines (divided into two subgroups):
- (a)
Phenylalkylamines (eg, verapamil) act mainly on cardiac cells and have negative inotropic and negative chronotropic effects. They have less vasodilatory action than dihydropyridines and, hence, cause less reflex tachycardia.
- (b)
Benzothiazepines (eg, diltiazem) combine the properties of dihydropyridines and phenylalkylamines. By exerting both cardiac depressant and vasodilatory actions, benzothiazepines are able to reduce arterial pressure without producing as much reflex cardiac stimulation as dihydropyridines.
- (a)
Generic name (Trade name) | Usual Daily Dosage (mg) | Peak Response (hr) | Duration of Response (hr) | Common Side Effects |
---|---|---|---|---|
Dihydropyridines | ||||
Amlodipine (Norvasc) | 5–10 qd | 6–12 | 24 | Headache, flushing, edema |
Felodipine (Plendil ER) | 2.5 qd | 2–5 | ||
Nifedipine GITS (Procardia XL) | 30–90 qd | 4–6 | ||
Nifedipine (Procardia, Adalat) | 10–30 tid | 0.1 | 4–6 | Headache, hypotension, dizziness, flushing, edema |
Nicardipine (Cardene) | 20–40 tid | 0.5–2 | 8 | |
Non-Dihydropyridines | ||||
Diltiazem (Cardizem) | 60–120 tid | 2.5–4 | 8 | Hypotension, bradycardia, dizziness, flushing, edema |
Diltiazem CD (Cardizem CD) | 240–280 qd | 10 | 24 | |
Verapamil (Calan, Isoptin) | 80–120 tid | 6–8 | 8 | |
Verapamil SR (Calan SR, Isoptin SR) | 120–240 bid | 5 | 12–24 |
Mechanism of Action
Transmembrane calcium influx occurs via a voltage-gated calcium channel consisting of four subunits: α 1 , α 2δ , α β , and α γ . The α 1 subunit is the dominant component of calcium channels and constitutes the pore structure for ion conduction. Ten different α 1 subunits have been reported, each with a specific distribution and ion conductance of its channels. These distinct subunits characterize the channel properties of L-, N-, T-, P-, Q-, and R-type calcium channels. Of these channels, the L-type is the main target for CCBs. The L-type voltage-gated calcium channel is responsible for excitation-contraction coupling of skeletal, smooth, and cardiac muscle. L-type voltage-gated calcium channels are also involved in conduction of the pacemaker signal in the heart. T-type calcium channels exhibit properties different from those of the L-type; they are involved in pacemaking and regulation of blood flow, but not in myocardial contraction.
All calcium antagonists cause dilatation of epicardial coronary vessels and arterial resistance vessels. Epicardial coronary vasodilatation is the primary mechanism responsible for the beneficial effect of calcium antagonists in relieving vasospastic angina.
CCBs in Angina
Nondihydropyridine CCBs reduce myocardial oxygen demand via their negative inotropic and chronotropic actions. Dihydropyridine CCBs are also postulated to improve the relative bioavailability of coronary artery nitric oxide and to improve endothelium-dependent vasodilator responses. These actions have led some to postulate that dihydropyridines favorably modify the natural history of atherosclerosis. Whether such an effect would be a direct pharmacologic effect of CCBs or secondary to their blood pressure-lowering effect is not clear.
All calcium-channel antagonists have similar antianginal efficacy. The choice of one agent or another is based primarily on pharmacodynamic characteristics, particularly whether or not a negative chronotropic effect is desired. The nondihydropyridines reduce heart rate, contractility, and blood pressure, thereby decreasing myocardial oxygen demand. The dihydropyridines are coronary vasodilators and also reduce myocardial oxygen demand by peripheral vasodilatation, thus lowering blood pressure and reducing myocardial wall tension.
Other Actions
Dihydropyridine CCBs, but not phenylalkylamine CCBs, increase endogenous fibrinolytic activity. Given the marked cellular changes associated with loss of normal calcium transport in atherosclerotic vessels, it has been proposed that CCBs slow the progression of CAD in addition to favorably effecting hemodynamics. Angiographic trials have shown significantly reduced formation of lesions in both patients with documented CAD and in animal models. In both in vitro and in vivo studies, amlodipine inhibited oxidative lipid damage.
Smooth-muscle cell proliferation and migration are early hallmarks of atheroma. Amlodipine inhibits smooth muscle cell proliferation following cholesterol enrichment at concentrations several orders of magnitude lower than those needed to inhibit calcium. Thus, amlodipine may interfere with certain adverse effects induced by cholesterol, including atherogenic changes in vessels. In addition, tumor necrosis factor-α (TNF-α), a cytokine that is elevated in atherosclerosis, mediates inflammatory damage to vessel walls. Amlodipine has inhibited TNF-α-induced endothelial apoptosis in a dose-dependent manner.
Clinical Evidence for the Efficacy of CCBs in Angina
Effective Treatment of Vasospastic Angina
CCBs are effective in the treatment of vasospastic angina. In one study, diltiazem (60 mg twice daily) reduced the mean frequency of vasospastic episodes during 72 hours from 43 to 5. In another randomized placebo-controlled study, patients were treated with either 10 mg of amlodipine once daily or placebo once daily for 4 weeks. The rate of vasospastic anginal episodes and the consumption of nitroglycerin tablets decreased significantly with amlodipine treatment.
Cardioprotection
Trials, including ACTION (A Coronary disease Trial Investigating Outcome with Nifedipine) and CAMELOT (Comparison of Amlodipine vs Enalapril to Limit Occurrences of Thrombosis), have documented that CCBs are safe and beneficial for patients with CAD. ACTION did not support a beneficial effect of nifedipine on cardiovascular outcomes. A subset analysis of patients with concurrent angina and hypertension, however, found that an extended-release dosage formulation sustained blood concentrations of nifedipine over 24 hours (nifedipine gastrointestinal therapeutic system), significantly reducing the combined incidence of all-cause mortality, MI, refractory angina, HF, stroke, and peripheral revascularization by 13%.
CAMELOT compared amlodipine or enalapril to placebo in normotensive patients with coronary artery disease ( n = 1991). Although BP reduction was similar in the two active treatment groups, adverse cardiovascular events occurred less frequently in the amlodipine group than in the enalapril group. Compared with atenolol in patients with hypertension and coronary artery disease, verapamil caused less new-onset of diabetes mellitus, fewer angina attacks, and less depression.
The Prospective Randomized Evaluation of the Vascular Effects of Norvasc Trial (PREVENT) was a 3-year multicenter, randomized, placebo-controlled trial that evaluated the effects of amlodipine on the development and progression of atherosclerotic lesions in coronary and carotid arteries among patients with CAD. Amlodipine therapy was associated with statistically significant slowing of carotid atherosclerosis progression, independent of blood pressure changes, and a reduced cardiovascular morbidity.
The Coronary Angioplasty Amlodipine Restenosis Study (CAPARES) was performed in a similar patient population as that of the PREVENT trial. Amlodipine significantly reduced the incidence of repeat PCIs and clinical events after PCI, without reducing luminal loss.
Pharmacokinetics
The pharmacokinetics of first-generation CCBs, including the nondihydropyridines verapamil and diltiazem, are similar. Even though the drugs are nearly completely absorbed after ingestion, their immediate bioavailability is offset by first-pass hepatic metabolism. Their onset of action is between 30 minutes and 2 hours, and their elimination half-lives range from 2 to 7 hours.
On the other hand, the second-generation CCBs, such as the dihydropyridine amlodipine, have a slower onset and longer duration of action, and a longer elimination half-life. These properties reduce the risk of reflex tachycardia and negative inotropy, thus making them relatively safe in patients with left ventricular dysfunction. The t max of amlodipine is 6 to 12 hours, and the elimination half-life is 35 to 50 hours. A slower duration of action can be achieved by using medications with sustained release formulations or by a gastrointestinal therapeutic system, which is available for nifedipine.
Interactions of CCBs with Other Drug Classes
Nondihydropyridine CCBs interact with other negative chronotropic or inotropic agents, particularly β-blockers. They should generally not be coprescribed with β-blockers, particularly in patients with left ventricular dysfunction or failure.
CCBs inhibit the hepatic cytochrome CYPA4 enzyme and may raise blood levels of statins and numerous other drugs. Cimetidine and grapefruit juice may raise the effective level of CCBs. Because magnesium is a calcium antagonist, magnesium supplements may enhance the actions of CCBs, particularly nifedipine.
Adverse Effects of CCBs
One of the potential adverse effects of using CCBs with a short duration of action, such as short-acting nifedipine (20–40 minutes), is that of increased mortality risk in patients with CAD. Reasons for this effect include a precipitous fall in blood pressure, reflexively triggering increased sympathetic activity and tachyarrhythmias.
A dihydropyridine CCB can be added to optimal doses of β-blockers and nitrates, with an acceptable safety profile. Common side effects of CCBs are peripheral edema, headache, dizziness, and constipation. Ankle edema is not secondary to increased sodium retention but rather from arteriolar dilatation producing an increase in capillary hydrostatic pressure. With nondihydropyridine CCBs, bradycardia and heart block can occur in patients who have significant conduction disorders. For patients with severe systolic dysfunction, nondihydropyridine CCBs can worsen or precipitate congestive HF. CCBs can also suppress lower esophageal sphincter contraction and worsen gastroesophageal reflux disease.
Novel CCBs
CCBs with sustained activity and T/N-type calcium channel-blocking action could provide more beneficial effects than classic CCBs, and may expand the clinical utility of these agents. Among these novel CCBs are benidipine, cilnidipine, and efonidipine.
Benidipine
Benidipine may be more efficacious in vasospastic angina than other CCBs. A meta-analysis compared the actions of benidipine, amlodipine, nifedipine, and diltiazem alone or in combination on major adverse cardiovascular events (MACE) in vasospastic angina patients. The hazard ratio (HR) for the occurrence of MACE was significantly lower for benidipine than other CCBs, even after correcting for patient characteristics that could have affected the occurrence of MACE. Possible explanations for this result with benidipine are greater NO production; preservation of levels of tetrahydrobiopterin, an essential cofactor for NOS; and greater vasoselectivity.
Cilnidipine
Cilnidipine is a dual blocker of L-type voltage-gated calcium channels in vascular smooth muscle and N-type calcium channels in sympathetic nerve terminals that supply blood vessels. Its reno-, neuro-, and cardioprotective effects have been demonstrated in clinical practice and in animal studies. In a study conducted in 2920 hypertensive patients, cilnidipine plus an angiotensin receptor blocker reduced heart rate significantly, particularly in patients with baseline heart rate greater than 75 beats per minute. In another study, cilnidipine relaxed arteries through calcium-channel antagonism and increased NO production by enhancing endothelial NOS in the human internal thoracic artery.
Efonidipine
Efonidipine blocks both L- and T-type calcium channels. In isolated animal myocardial and vascular models, efonidipine exerted potent negative chronotropic and vasodilator effects but only a weak negative inotropic effect. In both animal models and patients, reduction of blood pressure was accompanied by no or minimum reflex tachycardia. The result was improved myocardial oxygen balance and maintenance of cardiac output. Thus, efonidipine, an L- and T-type dual CCB, appears to be promising as an antihypertensive and antianginal drug with organ-protective effects in the heart and kidney.
Angiotensin Converting Enzyme Inhibitors, Angiotensin Receptor Blockers
Introduction
Whereas neither angiotensin converting enzyme (ACE) inhibitors nor angiotensin receptor blockers (ARBs) are usually regarded as antianginal drugs per se, they are included here because their antiarteriosclerotic, antiatherogenic, and vasodilator actions would clearly impact directly the severity and frequency of angina attacks.
ACE inhibitors are effective in reducing coronary events in high-risk patients and are recommended for consideration in patients with hypertension, diabetes, chronic kidney disease (CKD), and after myocardial ischemia. They have been proven to prevent and improve both ischemic HF and progression of CKD. When combined with thiazide diuretics, ACE inhibitors reduce the incidence of recurrent stroke.
Pharmacology and Pharmacodynamics
Endothelial cells are major mediators of the multistage process of atherosclerosis, and local angiotensin II and bradykinin levels are crucial to the functioning of these cells. ACE converts the hormone angiotensin I to the active vasoconstrictor angiotensin II and also accelerates the metabolic degradation of bradykinin. Four angiotensin II receptors have been identified, AT 1–4 . AT 1 receptors mediate vasoconstriction, aldosterone synthesis and secretion, cardiac hypertrophy, and vascular smooth muscle proliferation. The AT 2 receptor subtype is less well characterized, but there is evidence for its role in fetal tissue development, inhibition of cell differentiation, apoptosis, and possibly vasodilatation. AT 3 and AT 4 receptors are even less well characterized, but do not appear to affect vascular caliber, and in any case have as a ligand angiotensin IV, a metabolite of angiotensin II, and not angiotensin II itself.
Over 90% of ACE is found in tissue, only 10% in soluble form in plasma. Overexpression of tissue ACE in CAD disrupts the angiotensin II/bradykinin balance resulting in endothelial dysfunction. ACE inhibitors reduce the production of angiotensin II, which prevents vasoconstriction, reduces adhesion molecules and growth factors, decreases oxidative stress, and prevents apoptosis. A concomitant decrease in the degradation of bradykinin as a result of ACE inhibition contributes to vasodilation and an antiapoptotic action.
Classic Clinical Trials of ACE Inhibitors in Stable Coronary Artery Disease
ACE inhibitors are not considered to be primary therapy for angina; they do not immediately reduce the frequency or severity of angina attacks. However, several clinical studies have established ACE inhibitors, ARBs, and, in ischemic HF, aldosterone antagonists, as essential adjunctive therapy in many patients with coronary artery disease and angina. Their mode of action is to reduce the adverse impact of angiotensin II or aldosterone on the heart and vasculature, resulting in improved cardiovascular outcomes.
In the Heart Outcomes Prevention Evaluation (HOPE) study, which included 80% of subjects with a history of HF, and one-third with diabetes, treatment with ramipril versus placebo produced a 22% reduction in the composite endpoint of cardiovascular death, myocardial ischemia, and stroke in the ramipril-treated cohort. There was also a significant reduction in worsening angina (23.8% vs. 26.2%, risk ratio 0.89, 95% CI 0.82–0.96, p < 0.004). In the EUROPA trial, 12,218 patients were randomized to the ACE inhibitor perindopril or placebo. Treatment with perindopril (target dose, 8 mg daily) was associated with a 20% relative risk reduction in the composite endpoint of cardiovascular death, myocardial ischemia, or cardiac arrest ( p < 0.003).
The QUinapril Ischemic Event Trial (QUIET) tested the hypothesis that quinapril 20 mg daily would reduce ischemic events (cardiac death, resuscitated cardiac arrest, nonfatal myocardial ischemia, coronary artery bypass grafting, coronary angioplasty, or hospitalization for angina pectoris) and angiographic progression of HF in patients without systolic left ventricular dysfunction. A similar (38%) incidence of ischemic events occurred in the quinapril and placebo groups. Patients in the Prevention of Events with Angiotensin Converting Enzyme inhibition (PEACE) trial had stable CAD, angina (70% of the subjects), and normal or slightly reduced left ventricular function and were randomized to trandolapril or placebo. No difference between the groups was found in the incidence of the primary composite endpoint of cardiovascular death, myocardial ischemia, or coronary artery revascularization. The investigators concluded that ACE inhibitors might not be necessary as routine therapy in patients with low-risk ischemic heart disease with preserved left ventricular function, especially those who have received intensive treatment with revascularization and lipid-lowering agents.
Thus, two large studies in high-cardiovascular-risk patients (HOPE and EUROPA) showed cardiovascular protective effects by ACE inhibitors, and two studies in lower-cardiovascular-risk patients (QUIET and PEACE) did not. In a 2009 meta-analysis of ACE inhibition versus angiotensin receptor blockade, Baker et al reviewed nine trials and concluded that adding an ACE inhibitor to a standard regimen in patients with ischemic heart disease and preserved left ventricular function reduced total mortality and nonfatal myocardial ischemia at the expense of slightly increased syncope and cough. All but two ACE inhibitor trials found significantly fewer recurrent cardiac events using various agents. Most trials included few women (11–43%) and elderly patients (mean age 57 to 67 years). Whether the benefit associated with ACE inhibitors was a class effect was not clear. Despite these trial limitations, wider use of ACE inhibitors was supported in patients with ischemic heart disease and preserved left ventricular function.
One suggested mechanism for the beneficial action on cardiovascular outcomes of ACE inhibitors in patients with stable CAD and angina is blood pressure reduction. However, the reduction of blood pressure was quite modest in HOPE (3/2 mm Hg) and EUROPA (5/2 mm Hg). There is now some evidence from a EUROPA substudy for an alternative mode of action of ACE inhibitors. Serum from HF patients was found to significantly downregulate eNOS protein expression and activity significantly versus that of healthy controls ( p < 0.01), most probably as a result of upregulation of tissue ACE. One year of treatment with perindopril upregulated eNOS protein expression and activity. In addition, von Willebrand factor, endothelial apoptosis, tissue angiotensin II, and tumor necrosis factor were all elevated in patients with HF and reversed by perindopril. Thus, perindopril normalizes the angiotensin II/bradykinin balance, reduces inflammation, and prevents endothelial apoptosis. We do not know whether this activity is unique to perindopril or is a class effect of ACE inhibitors, and whether this is unique to patients with ischemic HF, or generalizable to all patients with CAD and stable angina.
Yet no study sought to assess whether or not an ACE inhibitor lessened the frequency and severity of angina as a primary outcome, until The Quinapril Anti-ischemia and Symptoms of Angina Reduction (QUASAR) trial, a double-blind, randomized, placebo-controlled, parallel-group study. Subjects ( n = 336), with stable angina but no left ventricular dysfunction or recent MI, were randomized to receive quinapril or placebo for a total of 16 weeks. Exercise-induced electrocardiographic changes, the Seattle Angina Questionnaire, and ambulatory ECG monitoring were the measured variables. There were no differences between the subjects treated with quinapril versus placebo in the time to induce a 1-mm ST-segment depression during an exercise treadmill test, in the mean value for the five scores of the Seattle Angina Questionnaire, or in the number of ischemic episodes seen on the ambulatory ECG.
Thus, ACE inhibitors should not be regarded as primary therapy for angina per se. However, there is abundant evidence to support their use to reduce adverse cardiovascular outcomes in patients with ischemic heart disease, particularly if the patients have hypertension, left ventricular dysfunction, or HF, have had a prior MI, or have diabetes or CKD.
Angiotensin Receptor Blockers in Stable Coronary Artery Disease
Several ARBs have been shown to reduce the incidence or severity of ischemic heart disease events, progression of renal disease in type 2 diabetes mellitus, and cerebrovascular events. ARBs are often considered to be an alternative therapy in individuals with cardiovascular disease who are intolerant of ACE inhibitors.
The Ongoing Telmisartan Alone and In Combination with Ramipril Global Endpoint Trial (ONTARGET) randomized 25,620 patients with vascular disease or high-risk diabetes (of whom 74% had a history of CAD) to the ACE inhibitor ramipril (10 mg/d), the ARB telmisartan (80 mg/d), or a combination of these two drugs. Although not the prespecified primary or secondary outcome, the authors recorded worsening or new angina. There was no difference between ramipril, telmisartan, and the combination in the incidence of this outcome measure.
In the Telmisartan Randomised Assessment Study in ACE Intolerant Subjects with Cardiovascular Disease (TRANSCEND), patients with cardiovascular disease or diabetes with end-organ damage were randomized to telmisartan (80 mg daily) or placebo. HF patients were excluded from TRANSCEND. The composite of cardiovascular death, nonfatal myocardial ischemia, and stroke occurred in 13% of patients on telmisartan versus 14.8% of the placebo group ( p = 0.048), and fewer patients in the telmisartan group had a cardiovascular hospitalization (30.3% vs 33%; p = 0.025). The investigators concluded that telmisartan exerted modest benefits on the composite endpoint of cardiovascular death, myocardial ischemia, and stroke.
In the “Valsartan” in Acute Myocardial Infarction Trial (VALIANT), the ARB valsartan had effects similar to those of the ACE inhibitor captopril in reducing cardiovascular event endpoints. The combination of the ARB with the ACE inhibitor yielded an increase in adverse events with no incremental benefit for cardiovascular events. Valsartan Antihypertensive Long-term Use Evaluation (VALUE) was a study of patients with hypertension and high cardiovascular risk. The rate of a composite outcome that included myocardial ischemia and HF in patients treated with valsartan was similar to that observed for the CCB amlodipine However, there were important differences in blood pressure control in the early stages of the VALUE trial (a significant blood pressure difference in favor of amlodipine) that may have confounded outcomes for myocardial ischemia and especially stroke.
There have also been some ARB trials with negative results. In one, the OPtimal Trial In Myocardial infarction with the Angiotensin II Antagonist Losartan (OPTIMAAL), beneficial cardiovascular outcomes were not shown. The lack of benefit in patients with complicated MI may have been attributable to inadequate doses of losartan.
Though ARBs can be substituted in patients intolerant to ACE inhibitors, more studies of ARBs in patients with ischemic heart disease are needed.
Guideline Recommendations for ACE Inhibitors and ARBs
The most recent guidelines for the management of patients with chronic stable angina have the following recommendations for the role of ACE inhibitors and ARBs in patients with chronic stable angina:
class I:
- 1.
ACE inhibitors should be prescribed in all patients with stable ischemic heart disease who also have hypertension, diabetes mellitus, left ventricular ejection fraction 40% or less, or CKD, unless contraindicated.(Level of Evidence: A)
- 2.
ARBs are recommended for patients with SIHD who have hypertension, diabetes mellitus, LV systolic dysfunction, or CKD and have indications for, but are intolerant of, ACE inhibitors. (Level of Evidence: A)
- 1.
class IIa:
- 1.
Treatment with an ACE inhibitor is reasonable in patients with both SIHD and other vascular disease. (Level of Evidence: B)
- 2.
It is reasonable to use ARBs in other patients who are ACE inhibitor intolerant. (Level of Evidence: C)
- 1.
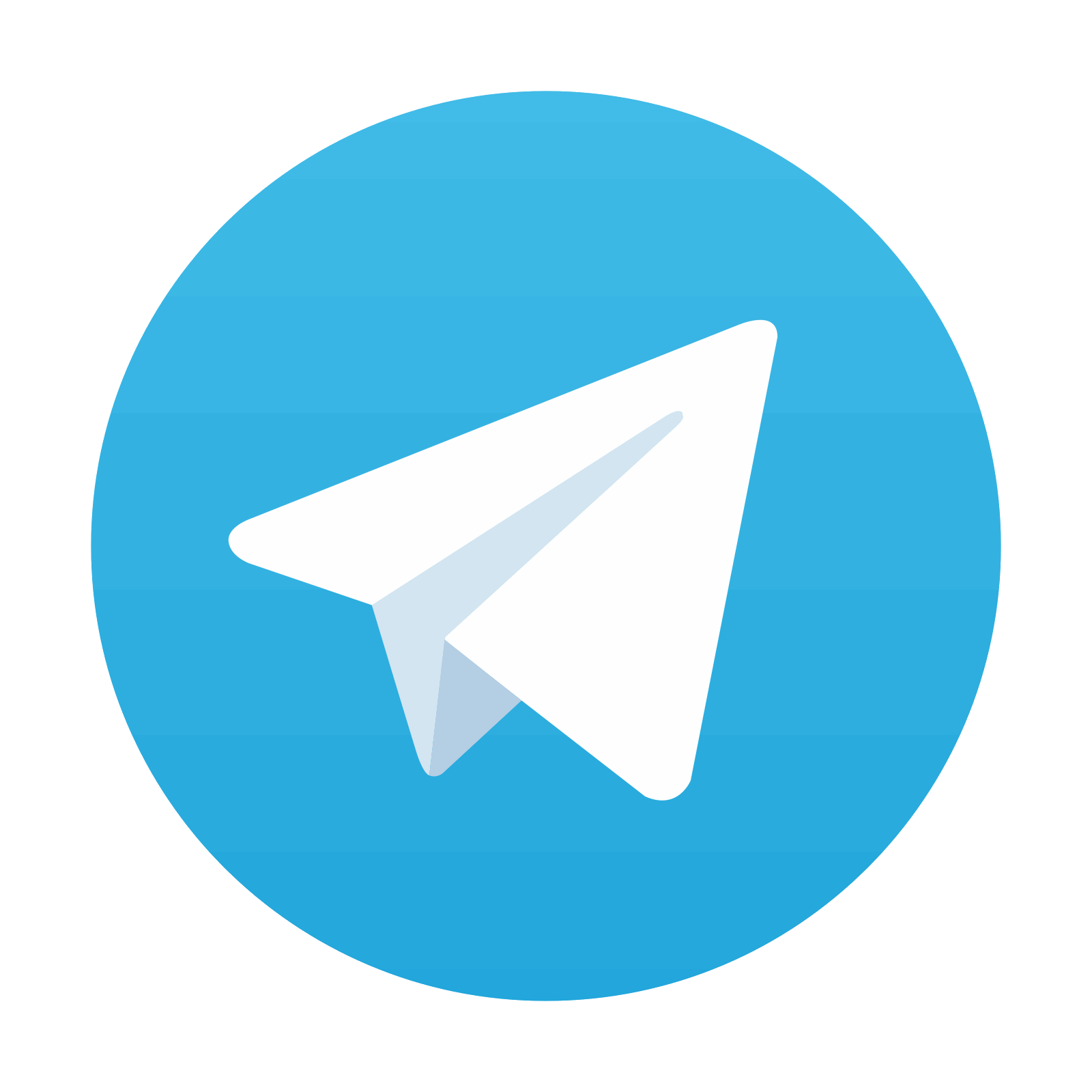
Stay updated, free articles. Join our Telegram channel

Full access? Get Clinical Tree
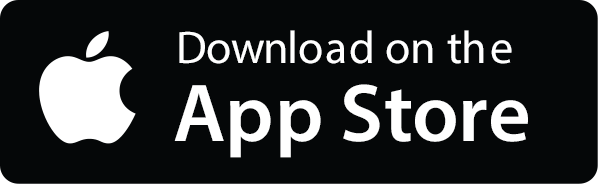
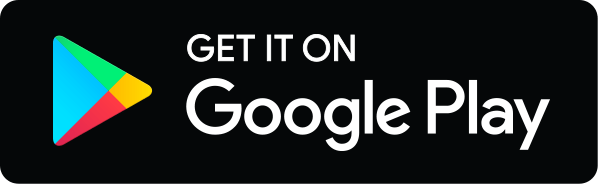