Fig. 7.1
Schematic representation of a sarcomere with a scheme showing its main molecular constituents below. Myosin is represented in blue, titin in red and the M-band in purple. Dashed lines indicate that the proteins extend beyond the font. TK titin kinase domain
Over the years the Z-disc has attracted a lot of attention, and a multitude of proteins were identified that localise there. These range from mainly structural components such as alpha-actinin to signalling proteins such as MLP (muscle LIM protein) and phosphatases such as calcineurin (reviewed in Frank and Frey 2011; Luther 2009). The M-band is much less studied and only a handful of bona fide components are known at present (Lange et al. 2005a; see also Fig. 7.1). These comprise proteins with mainly a structural function such as the three members of the myomesin family but also enzymes such as MM-CK (muscle creatine kinase) and proteins containing signalling domains such as obscurin (Young et al. 2001; Fig. 7.1). The aim of this review is to describe the M-band in more detail and to discuss its role in the sarcomere beyond myofibrillogenesis and in particular in cardiomyopathy.
7.2 M-Band Function: Balancing the Forces in the Contracting Sarcomere
The muscle structure is rather elastic, and the original almost crystalline order of the contractile filaments is restored even after substantial deformations caused either by passive force applied from the outside or the forces that arise by active contraction of the muscle. It is achieved due to the smart integration of numerous viscoelastic elements into the sarcomere cytoskeleton. The M-band makes up an important part of this construction, and its elasticity allows for reversible deformations of the myosin filament lattice (Tskhovrebova and Trinick 2012).
However, in addition to acting as an elastic spacer and restoring the order after deformation, the M-band might have a distinct function in the contracting muscle. This role is suggested by the variable appearance and distinct protein composition of the M-band in different muscle types.
The thick filaments are attached to the Z-discs by titin filaments, the elasticity of which keeps them in the central position in the passive sarcomere. However, this central position is intrinsically unstable during sarcomere activation. Indeed, any small force imbalance between the two filament halves, caused, for example, by a different proportion of activated crossbridges or by slightly different overlap with the actin filaments on one side, would lead to the deviation of the myosin filaments from the centre position and accordingly to a higher displacing force. The dramatic changes that occur to the arrangement of the A- and M-bands in an activated muscle were demonstrated in electron micrographs by Horowits and Podolsky in the 1980s (Horowits and Podolsky 1987).
A simple calculation based on single-molecule measurements of titin elasticity shows that the restoring force produced by titin alone might not be sufficient to compensate for the intrinsic instability of individual myosin filaments. However, the cross-links at the M-band might be rather helpful in this respect, as they provide an averaging of the force imbalances between all myosin filaments present in one sarcomere (Agarkova and Perriard 2005). Assuming that force differences are completely random, the total force reduction due to the M-band cross-links is equal to the square root of the thick filament number, giving a factor of 30 (as the standard sarcomere consists of about 1,000 myosin filaments). Thus, theoretically, due to the presence of the M-band, the restoring force generated by titin filaments in the whole sarcomere is substantially amplified by 30 times.
7.3 Structure of the M-Band as Studied by Electron Microscopy
The M-band is apparent as a series of transverse dark lines in electron micrographs of longitudinal sections of striated muscle in the middle between two Z-discs. Three to five M-lines separated by 22 nm intervals are discernible in the central “bare” zone of the myosin filaments that lacks the myosin heads. The numbers of lines and their intensity vary between striated muscle types, which is probably due to distinct molecular composition (Lange et al. 2005a; Schoenauer et al. 2008). In the cardiac muscle the appearance of the M-band depends on the developmental stage and on the functional state. For example, in electron micrographs of the embryonic heart, it is not possible to discern any discrete M-band pattern (Manasek 1968), while in adult cardiac muscle, usually five lines can be distinguished in the M-band region, with slight variation in intensity between different species (Pask et al. 1994). This picture changes in disease, where the ultrastructure of the M-band becomes less distinct again (Ehler et al. 2001).
7.4 The Myomesin Protein Family
The main players in the M-band are the three members of the myomesin protein family. Historically the first discovered family member was M-protein (myomesin-2, MYOM2), originally detected by Masaki and Takaiti (1974) and subsequently characterised more closely by Eppenberger et al. (1981). The “proper” myomesin (myomesin-1, MYOM1) was identified a few years later using monoclonal antibodies against purified M-band fractions and was subsequently shown to be a 185 kDa protein (Grove et al. 1984). These two M-band components were correlated to the appearance of the M1 (M-protein) and M4, M4′ lines (myomesin) on electron micrographs of the sarcomere (Obermann et al. 1996). The additional M6, M6′ lines may be due to the presence of myomesin-3, which was identified recently by comparative sequence analysis of a mouse gene database (Schoenauer et al. 2008).
The most important member of the myomesin protein family is the protein myomesin (Fig. 7.2). It is found in all types of vertebrate striated muscles that were investigated (Agarkova et al. 2000) as well as in the first sarcomeres formed in the developing heart (Ehler et al. 1999). Accordingly, it is expressed in a nearly stoichiometrical ratio to myosin in various muscle types (Agarkova et al. 2004; see also Fig. 7.3). In contrast to myomesin, the other two protein family members, M-protein and myomesin-3, show restricted, muscle type-specific expression patterns. M-protein is found in adult heart and fast skeletal muscle (Grove et al. 1985). Myomesin-3 appears in fibres of intermediate speed in skeletal muscle and is differentially expressed in the mouse and human heart (Schoenauer et al. 2008; see also Fig. 7.3).
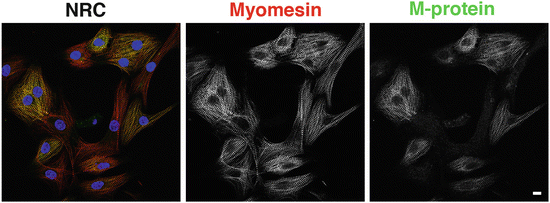
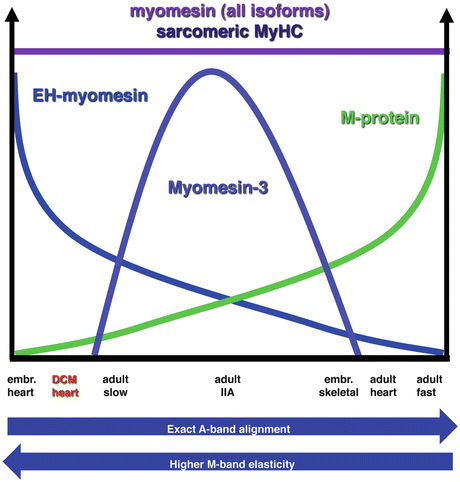
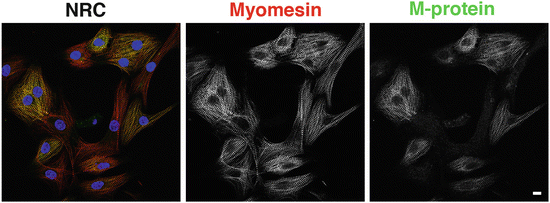
Fig. 7.2
Myomesin and M-protein are differentially expressed in neonatal rat cardiomyocytes (NRC) in culture. Confocal micrographs of NRC stained with antibodies against myomesin (red signal in overlay) and against M-protein (green signal in overlay) show that myomesin is present in every single M-band in every NRC; some of the NRC have not yet switched on M-protein expression and thus lack a yellow signal in the overlay due to colocalisation. Nuclei were stained with DAPI and are depicted in blue. Scale bar equals 10 μm
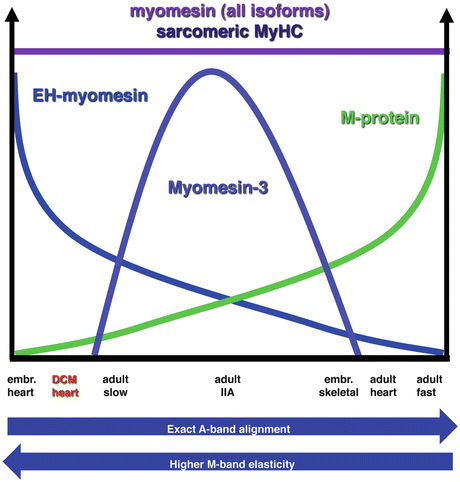
Fig. 7.3
Chart showing the expression of different myomesin family members in different muscle types. While the expression levels of myomesin and sarcomeric myosin are always tightly correlated, the adult heart expresses high levels of M-protein, while the embryonic heart expresses high levels of the splice variant EH-myomesin. Myomesin-3 is expressed in intermediate contraction speed fibres. The combination of myomesin family members expressed affects the elasticity and also the alignment of the A-bands and hence the clear visibility of M-lines in the electron microscope
7.5 Myomesin Protein Family: Dimers That Cross-Link Myosin Filaments
The genes that encode the three members of the myomesin family are closely related, suggesting a common ancestor. The published genome of the non-vertebrate Ciona intestinalis contains only one myomesin gene. This indicates that the myomesin protein family may have arisen as a result of two genome-wide duplications and subsequent functional diversification, which occurred in the vertebrate lineage after separation from the common ancestor of all chordates (Putnam et al. 2008). In addition to a close relation on the gene level, the three members of the myomesin family share an identical domain arrangement. The N-terminal domain of myomesin, My1, binds to myosin and is predicted to have an intrinsically disordered/unstructured conformation (Obermann et al. 1995). Such unstructured protein fragments are often associated with molecular recognition and target-induced binding. According to amino acid comparison, the N-terminal domains of the three myomesin family members are the most diverse part of the molecule. It is suggested that they may serve to target each family member to a certain position in the bare zone of the thick filament (Schoenauer et al. 2008). The remainder of the molecule is comprised of identically arranged immunoglobulin-like (Ig) and fibronectin type III (Fn) domains (Schoenauer et al. 2008; Steiner et al. 1999; see Fig. 7.4). The C-terminal domains of myomesin, My13, are able to dimerise (Lange et al. 2005b; see Fig. 7.4). The crystal structure of this immunoglobulin-like domain revealed an antiparallel dimerisation mediated by intermolecular beta-sheets (Pinotsis et al. 2008). The same molecular mechanism is observed in assembly complexes of titin and also of filamin (Pinotsis et al. 2009). Despite the high sequence identity, the corresponding C-terminal domains of all three myomesin family members interact only in a homotypic manner, i.e. My13 only interacts with itself but not with the corresponding domains of M-protein or myomesin-3 (Schoenauer et al. 2008). This suggests that they all form antiparallel dimers which act as a structural bridge between neighbouring myosin molecules, while the dimer of each myomesin family member has a specific lateral position along the central zone of the myosin filament, which is defined by the sequence of its N-terminal domain.
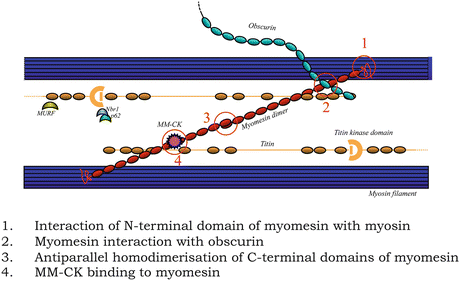
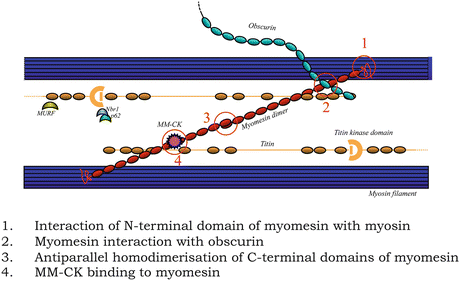
Fig. 7.4
Schematic representation of the molecular domain interactions of the three M-band components from which structural data is available, myomesin (red), titin (brown) and obscurin (turquoise). The antiparallel myosin tails are depicted as a single blue bar
7.6 Alternative Splicing of Myomesin
In contrast to M-protein and myomesin-3, myomesin has splice variants. In the embryonic heart, a splice variant of myomesin is predominant that contains an insert of about 100-amino acid length between domains My6 and My7 (Agarkova et al. 2000). This myomesin isoform is found also in slow and extraocular muscles (Agarkova et al. 2004; Wiesen et al. 2007). Single-molecule experiments showed that the alternatively spliced EH (embryonic heart) insert has a disordered conformation and functions like an entropic spring, similar to the PEVK domain in titin (Schoenauer et al. 2005). EH-myomesin was proposed to confer more elasticity to the M-bands in myofibrils working under eccentric contraction conditions (Agarkova and Perriard 2005). In a healthy adult heart, expression of this splice variant is restricted to the conduction tissue (Meysen et al. 2007). However, in dilated cardiomyopathy, which among other subcellular defects is characterised by less well-aligned myofibrils (Ehler et al. 2001), an upregulation of the EH-myomesin isoform is correlated to the functional status of the failing heart (Schoenauer et al. 2011).
7.7 Correlation of M-Band Appearance and Molecular Composition
How are all these different components arranged in three dimensions, and is there any correlation between molecular composition and M-band appearance in the electron microscope? The problem is that the electron dense appearance of the M-lines on electron micrographs is rather due to the decoration with the muscle isoform of creatine kinase (MM-CK) which sits on the myomesin molecule than the myomesin molecules themselves (Hornemann et al. 2003). The chains of immunoglobulin- and fibronectin-like domains alone are not visible on electron micrographs. However, some parallels are established. The most prominent M4/M4′ lines may represent the MM-CK molecules attached to myomesin which is found in all muscles. Expression of M-protein correlates to the presence of the central M1 line (Obermann et al. 1996), while the M6/M6′ lines are linked to the expression of myomesin-3 in intermediate speed fibres (Schoenauer et al. 2008). In addition, the M-lines are not discernible in electron micrographs of embryonic heart muscle, which is currently explained by a more extended and flexible M-band structure of this muscle leading to tiny misalignments and thus a more diffuse distribution of the MM-CK dots that decorate the M-band proteins (Agarkova et al. 2000). In transverse sections, the M-band shows a hexagonal arrangement that can be very well correlated with models that include myomesin dimers (Lange et al. 2005a). Hopefully, a three-dimensional single-particle analysis of electron micrographs of negatively stained muscle, as beautifully shown by Al-Khayat for the myosin head-containing zone of the A-band, will be available soon to shed more light on the M-band structure as well (Al-Khayat et al. 2013).
7.8 Other M-Band Components
Apart from these structural components of the M-band, two obvious additional components must be considered, the C-termini of the titin molecules, which stretch all the way from the Z-disc, and the myosin tails that constitute the bare zone of the A-band. As far as titin is concerned, it is assumed that C-termini from opposite Z-discs overlap in an antiparallel fashion (Obermann et al. 1996). At the edge of the M-band region, the titin molecule contains a kinase domain, which can be activated by mechanical stretch (Gräter et al. 2005). While there is some information on the organisation of myosin in the rest of the A-band (Al-Khayat et al. 2013), how the very tails of this molecule are arranged in the M-band is still a mystery.
Until the beginning of this century the above-mentioned proteins were the only known structural components of the M-band. However, in the last decade a number of novel players were identified that move the M-band from a merely structural connection site to a potential node for signalling (Fig. 7.1). The first of these was the finding that obscurin, another huge modular protein, mainly composed of immunoglobulin-like domains, is found predominantly at the M-band in the adult heart (Young et al. 2001). Obscurin has a molecular weight of 800 kDa and possesses a calmodulin-binding IQ motif and a Rho guanine nucleotide exchange factor domain at its C-terminus. Splice variants exist that replace this stretch with one or two kinase domains (Small et al. 2004). So far a signalling role of obscurin has remained elusive, and obscurin knockout mice do not show any M-band phenotype, but have a defect in their sarcoplasmic reticulum organisation (Lange et al. 2009). Recently a molecular link of obscurin to the utmost C-terminal domain of titin, M10 and domains My4 and My5 in myomesin was demonstrated (Fukuzawa et al. 2008). Reducing myomesin expression levels in cardiomyocytes leads to a loss of obscurin at the M-band (Fukuzawa et al. 2008). A smaller relative of obscurin, ObsL-1 shows the same molecular interactions, and it is assumed that while obscurin is the main interaction partner at the periphery of the myofibrils, ObsL-1 is the main link within the myofibrils between titin M10 and myomesin. However, the titin M10 domain appears to be a busy site for interactions, since it was also shown recently to bind to myospryn (Sarparanta et al. 2010). This is especially interesting, since myospryn can also bind a regulatory subunit of protein kinase A (PKA), which in turn is known to phosphorylate the linker between My4 and My5 in myomesin that binds to obscurin (Obermann et al. 1997). Currently the significance of this web of interactions and how it is correlated to different states of M-band activity and function is unclear.
Apart from these rather large proteins, a number of smaller molecules were found to target to the M-band region, often due to their association with the stretch of titin that spans from its kinase domain to its C-terminus. Among these is DRAL/FHL2, which binds to the is2 region of M-band titin and seems to act as a hub for the association of metabolic enzymes such as creatine kinase, adenylate kinase and phosphofructokinase (Lange et al. 2002). The E3 ubiquitin ligase MURF1 associates with a domain in titin close to the titin kinase domain, suggesting a possible link to titin protein turnover (Centner et al. 2001). More recently, Myomasp, which is a leucine-rich protein, was found to locate to the M-band, and a direct interaction with the C-terminus of the myosin heavy chain was shown (Will et al. 2010).
7.9 What Happens if Myomesin Is Stretched
The force-buffering function of the M-band relies on the robustness of the cross-links between myosin filaments. In the relaxed sarcomere, the myomesin dimers are in a compact zero-force conformation and keep the myosin filaments in register. During sarcomere activation the myomesin dimers may work as force-transmitting bridges to balance the shifting forces between the neighbouring myosin filaments. A series of brilliant single-molecule studies revealed the smart molecular mechanisms, which allow the myomesin dimers to establish that tough but yet extensible link. It became clear that the stability of the M-band bridges in all possible working regiments of the muscles is achieved by a remarkable mechanical hierarchy of unfolding—refolding processes of the various structural modules that make up the myomesin molecule (Xiao and Grater 2014). The first study from 2005 used AFM to characterise the “mechanical fingerprints” of the typical modules that constitute the myomesin molecule, including one Fn-like domain (My6), one Ig-like domain (My10) and the alternatively spliced EH-fragment (Schoenauer et al. 2005). The EH-insert was found to be largely unfolded and was considered to function as an entropic spring, analogous to the PEVK domain of titin. According to the single-molecule measurements, inclusion of two EH-inserts in the myomesin bridge allows for about 70 nm elastic extension, which nearly doubles the predicted length of the whole dimer. This considerably increases the working range of the M-band bridges without the need to unfold the Fn-/Ig-like domains. However, in muscles lacking the EH-myomesin isoform, the elongation of the myomesin molecule is achieved by other mechanisms. After the initial finding that myomesin forms antiparallel dimers via its My13 domain (Lange et al. 2005b), a structural analysis was carried out, and it was found that the dimerised domains make up a beta-sheet complex similar to filamin dimerisation and obscurin/titin interaction domains (Pernigo et al. 2010; Pinotsis et al. 2008).
When the structural analysis was extended towards the N-terminus spanning My9–My13, it was revealed that unlike many other linkers between modular domains, the linkers between domains My9 and My13 of the myomesin molecule attain an alpha-helical conformation (Pinotsis et al. 2012). Using a unique approach, this study has identified the superhelical coil arrangement of this dimer, composed of the C-terminal portion of myomesin, My9–My13. The unfolding of the alpha-helical linkers allows for an extension of up to 2.5-fold of the original length (Pinotsis et al. 2012).
Myomesin is a molecular spring: in the relaxed state it is in a coiled conformation. Its behaviour during extension might be modelled as a series of elastic springs with different stiffness together with the viscous elements, corresponding to the unfolding of individual domains. According to the mechanical hierarchy, the stretching would first unravel the whole Fn/Ig domains chain, followed by the extension of the EH-segment. In those muscles, which do not have EH-myomesin, a stretching force of about 30 pN (lacking the EH-segment) will lead to the sequential unfolding of the alpha-helical linkers in the C-terminal part of the molecule. In contrast to the extension of the elastic regions, this process will constantly absorb the energy. The Fn and Ig domains demonstrate substantially higher rupture forces and might be used as “shock absorbers” of the last bastion, because the refolding of these domains might need a longer relaxation time. The mechanical stability of the Ig domains increases towards the C-terminal part of the myomesin, while the dimerising My13 domain is the most robust one (Berkemeier et al. 2011). Molecular dynamics simulations have shown that the stability of the antiparallel dimer outperforms all other Ig domains of myomesin, ensuring the intactness of the myomesin cross-link even when all intermediate domains are unfolded (Xiao and Grater 2014). Thus, myomesin acts as a serial link of the various structural modules, whose complex viscoelastic properties allow for the reversible extension, and thus safeguards the integrity of the M-band bridges during muscle contraction.
< div class='tao-gold-member'>
Only gold members can continue reading. Log In or Register a > to continue
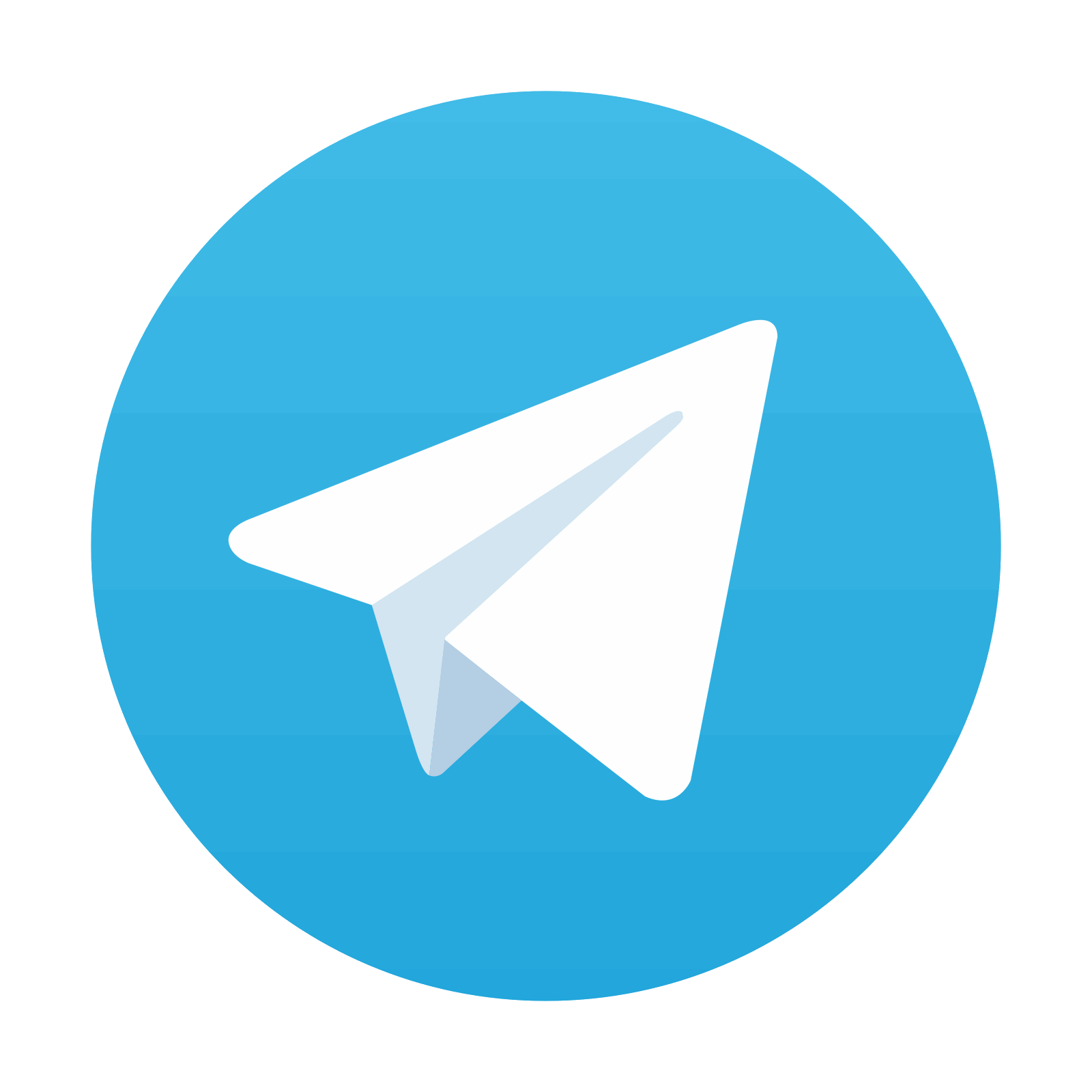
Stay updated, free articles. Join our Telegram channel

Full access? Get Clinical Tree
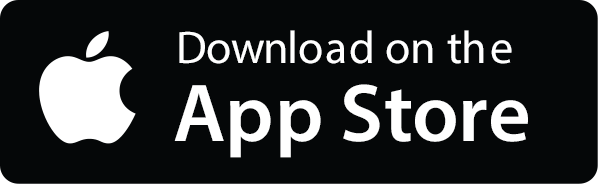
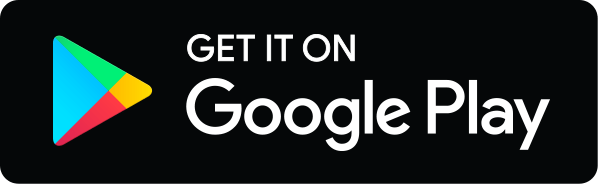