Background
Right ventricular (RV) dysfunction in chronic heart failure (HF) is associated with poor prognosis. Cardiac resynchronization therapy (CRT) is an established method of improving prognosis in HF. However, the majority of known indices predictive of response to CRT are based on left ventricular (LV) assessment. The authors hypothesized that baseline RV function and tissue Doppler–derived dyssynchrony may have incremental value over LV dyssynchrony measures for predicting CRT response.
Methods
In this retrospective study, echocardiographic examinations were performed in 90 patients before pacemaker implantation and up to 18 months afterward. CRT results were evaluated using clinical criteria (death, hospitalization for decompensation, change in New York Heart Association class ≥1, and 10% decreases in both peak ventilatory oxygen uptake and 6-min walking distance) and reverse remodeling (>15% reduction in LV end-systolic volume).
Results
Baseline RV dyssynchrony during isovolumic contraction of 26 msec facilitated the segregation of responders from nonresponders with 85% sensitivity and 100% specificity, as well as synchrony in peak deformation of 54 msec, with 89% sensitivity and 67% specificity. The minor axis of the RV inflow tract predicted reverse remodeling after CRT with sensitivity of 73% and specificity of 58% with a cutoff value of 35 mm. According to the clinical criteria, LV indices (end-diastolic and end-systolic volumes) and interventricular delay gave an overall R 2 value of 0.20 (86.2% correctly classified patients; area under the curve, 0.80). The addition of RV dyssynchrony parameters (measured in peak strain and isovolumic contraction peak velocities) significantly increased the power of the model ( R 2 = 0.86; 100% of patients correctly classified; area under the curve, 1; P for change in R 2 < .0001).
Conclusions
The value of baseline RV function analysis is incremental to LV indices for the prediction of clinical response to CRT but not reverse remodeling. RV synchronous longitudinal deformation and RV dyssynchronous isovolumic velocity are independent predictors of clinical response to CRT.
Chronic heart failure (HF) has become the most prevalent cause of mortality, morbidity, and hospitalization in industrialized countries. Cardiac resynchronization therapy (CRT) is one of the treatment options in patients with advanced chronic HF in New York Heart Association class III or IV, who are symptomatic despite optimal medical therapy, and who have reduced left ventricular (LV) ejection fractions (<35%) and QRS prolongation (QRS width ≥ 120 msec). Despite numerous investigations mainly using LV mechanical dyssynchrony parameters, the complete profile of a CRT responder has yet to be fully recognized.
In advanced HF due to LV dysfunction, accompanying right ventricular (RV) impairment is associated with poor exercise capacity and plays an important prognostic role. RV dyssynchrony, as measured by Doppler-derived strain (ϵ), has been documented in patients with HF and has been shown to correlate with both pulmonary hypertension and the degree of RV systolic dysfunction.
Thus, we hypothesized that RV baseline function analysis is incremental to LV evaluation and may have an impact on the results of resynchronization therapy.
Methods
Patient Selection and Study Protocol
In this retrospective investigation, the study group consisted of 90 consecutive patients with chronic HF who fulfilled the recommended criteria : NYHA class III or IV, symptomatic despite optimal medical therapy, reduced LV ejection fraction (≤35%), LV dilatation (LV end-diastolic diameter ≥ 30 mm/height in meters ), and QRS duration > 120 msec. Furthermore, CRT was implemented in patients who fulfilled additional echocardiographic criteria: interventricular dyssynchrony (LV pre-ejection period ≥ 140 msec or difference between LV and RV pre-ejection periods ≥ 40 msec ) or LV intraventricular dyssynchrony (delay between onset and peak [onset to onset or peak to peak] systolic velocities between basal segments of opposite LV walls ≥ 60 msec in ≥1 apical view ). Patients with either interventricular or intraventricular dyssynchrony parameters of left ventricle were eligible for the enrollment. The complex criteria were used as a summary and simplification of published indices (difference of 5 msec in septal-to-lateral delay and multiple apical views ) to increase the response rate.
Patients with classic dual-chamber pacemakers upgraded to CRT, and those without evidence of electromechanical dyssynchrony by echocardiography were excluded.
Ischemic etiology of chronic HF was confirmed by coronary angiography ≥1 year before CRT and/or on the basis of a documented previous myocardial infarction, without new onset of ischemic heart disease. Patients with severe stages of chronic obstructive pulmonary disease were excluded from the study. Pharmacologic treatment was optimal in all cases for ≥90 days before CRT.
Serial Examinations
Echocardiography, 6-min walking test, electrocardiography, and maximum oxygen uptake measurements were performed before CRT and then 1, 6, 12, and 18 months after device implantation. Echocardiographically guided optimization of pacemaker settings, including atrioventricular and interventricular delays, was carried out 3.6 ± 1.2 days after implantation and then at each follow-up visit, if necessary. Atrioventricular dyssynchrony was optimized using an LV diastolic filling profile. Interventricular delay programming was carried out on the basis of the measurements of pre-ejection periods and an online analysis of LV myocardial velocities.
This study complied with the Declaration of Helsinki, and the research protocol was approved by the local ethics committee. Patients gave consent to undergo standard diagnostic and therapeutic procedures, including CRT implantation and echocardiographic studies.
Two-Dimensional Echocardiography
Examinations were performed using commercially available equipment (Vivid 5; GE Vingmed Ultrasound AS, Horten, Norway), with a transthoracic 2.5-MHz phased-array transducer. Three cardiac cycles with simultaneous electrocardiographic recording were collected in passively held end-expiration and stored in cine loop format at a frame rate > 100 frames/sec and sectors of 35° to 75°. During CRT, cine loops were recorded in DDD or VDD mode, according to the dominant pacing mode for data recollection (>50% of time analyzed). Recordings were taken from standard parasternal and apical views. Quantitative offline analysis of color Doppler cine loops was performed using EchoPAC workstation version 6.4.2 (GE Vingmed Ultrasound AS).
The reference points used for electromechanical coupling were the onset of the QRS complex and aortic valve opening and closure.
Two-dimensional echocardiographic parameters, such as LV end-diastolic and LV end-systolic diameter, LV end-diastolic and LV end-systolic volume, tricuspid annular plane systolic excursion, and biplane ejection fraction by Simpson’s method, were retrieved.
RV Chamber
All RV chamber measurements were collected in an apical four-chamber view. RV end-systolic and end-diastolic areas (RV inflow tract, apical four-chamber view) and the fraction of area change were measured. The minor axis of the RV inflow tract was measured within one third of the distance below the tricuspid annulus toward the apical portion of the right ventricle. Peak tricuspid regurgitant velocity and inferior vena cava diameter were used to calculate RV systolic pressure. Pulmonary hypertension (RV systolic pressure ≥ 35 mmHg) was evidenced in 59 patients (55%).
Interventricular Dyssynchrony
Interventricular dyssynchrony is defined as the delay between LV and RV ejection. Using a pulsed-wave spectral velocity profile of the LV and RV outflow tracts, periods from the onset of QRS to the onset of LV and RV ejection (the pre-ejection period) are measured and then subtracted to obtain the value of interventricular dyssynchrony.
Doppler Tissue Imaging
A region of interest (3 × 3 pixels for the right ventricle and 5 × 5 pixels for the left ventricle) was placed within the myocardium and semiautomatically tracked throughout the cardiac cycle to avoid signal collection from the blood pool or the surrounding tissue. For ϵ estimation, a distance of 9.7 mm was used.
Regional Velocity Analysis
Myocardial longitudinal velocity curves were derived from 12 LV segments: basal and medial and two RV segments. Each of the profiles was used to assess systolic synchronicity within the isovolumic and ejection phases ( Figure 1 ). The time delay to the onset and to the peak of myocardial systolic velocity between the opposite walls measured in long-axis apical (basal and medial segments) views was calculated. Similarly, time to maximal velocity was measured during isovolumic contraction. RV intraventricular dyssynchrony was defined as the delay between the RV free wall and the interventricular septum ( Figure 2 A).


Regional ϵ Analysis
On the basis of previous data reporting a greater contribution of longitudinal than circumferential RV shortening to RV stroke volume, deformation parameters were measured in apical views only. For longitudinal deformation, Doppler-derived ϵ was analyzed, and time to peak ϵ was calculated. RV dyssynchrony ( Figure 2 B) and LV delays were defined as the delay between time to peak ϵ between the opposite walls and segments acquired in the apical four-chamber view. The time delay between systolic velocity peaks of opposite walls and segments was measured. The same procedure was performed between peak isovolumic velocity and negative ϵ ( Appendix A ).
Definitions of CRT Response
CRT results were evaluated using two sets of criteria. The clinical criteria encompassed patients who were alive, were not hospitalized for HF decompensation, with NYHA class decreases ≥ 1, and with increases of >10% in both peak ventilatory oxygen uptake and 6-min walking distance (all components mandatory-modified from Lecog et al ). According to the response definition based on reverse remodeling, responders demonstrated >15% reductions in LV end-systolic volume. Regarding each set of criteria separately, all components had to be fulfilled for a patient to be considered a responder.
Device Implantation
Standard implantation procedures were performed using the cut-down technique of the cephalic vein. A right atrial lead was inserted into the appendage, an RV lead into the distal segment of the interventricular septum, and one or two LV leads into the posterolateral, lateral, and anterolateral interventricular or middle cardiac veins. If the patient was qualified to receive a CRT defibrillator, an RV defibrillating electrode was positioned first. The LV lead was implanted using the left heart delivery system Attain (Medtronic, Inc., Minneapolis, MN). In 24 patients undergoing dual-site LV stimulation, a second LV lead was positioned in the appropriate branch of the coronary sinus, with the intention to reach as wide separation from the first one as possible. Subsequently, the two bipolar or one unipolar and one bipolar electrodes (depending on vein diameter) were connected by a Y-adaptor (model 2827; Medtronic, Inc.), with the intention to create a system with one bipolar lead. The procedure was finalized with InSync III or InSync Protect (Medtronic, Inc.) pacemaker implantation. The lower rate in DDD mode was set at 60 beats/min, LV pacing polarity on unipolar (CRT) or LV tip to RV coil (CRT defibrillation). The atrioventricular and biventricular pacing configurations were then optimized under echocardiographic guidance.
Statistical Analysis
To compare parametric variables before and after CRT, two-tailed paired-samples t tests were used, while unpaired t tests were used to compare responders and nonresponders. Continuous data are presented as mean ± SD and categorical data as numbers and percentages. To identify independent predictors of response among LV and RV morphologic, isovolumic, and ejection dyssynchrony parameters, stepwise multivariate logistic regression analyses were performed with regard to the clinical and reverse remodeling criteria. The parameters (13 dyssynchrony variables and 12 standard echocardiographic measures) considered in the regression analysis were as follows: LV end-diastolic and end-systolic diameters and volumes (as well as these values indexed to body surface area), RV inflow tract diameter, RV end-diastolic and end-systolic areas, RV fractional area change, interventricular delay, LV pre-ejection period, tricuspid annular plane systolic excursion, and RV systolic pressure; for the right ventricle; isovolumic (in time to peak velocity) and ejection dyssynchrony in velocity (in time to onset and time to peak) and ϵ (in time to peak) between the right ventricle and septum; and for the left ventricle; the standard deviation of assessed segments in six LV walls. LV end-systolic volume and its index were excluded from regression analysis of echocardiographic criteria. A stepwise approach (forward selection with switching to increase the likelihood) was used. Independent predictors are expressed as adjusted odds ratios with 95% confidence intervals. Subsequently, receiver operating characteristic analysis was performed, and areas under the curves (AUCs) were calculated (for each independent response predictor). P values < .05 were considered significant. A statistically significant increase in the global model R 2 and the percentage of patients correctly classified (according to response criteria), after the addition of RV variables, defined incremental prognostic value. For this purpose, NCSS (NCSS, Kaysville, UT) and Statistica version 8 (StatSoft, Inc., Tulsa, OK) were used.
Interobserver and intraobserver variability were studied by means of independent analyses of 15 data sets (seven baseline, six follow-up) by two independent observers blinded to the overall response and by repeated analyses of the same data sets 10 months after the initial analysis by the same reader. Interobserver and intraobserver variability were analyzed using Bland-Altman statistics with MedCalc version 9.0.1.1 (MedCalc Software, Mariakerke, Belgium).
Results
Clinical Outcomes
Within 18 months of follow-up, 13 patients died. The causes of death were cardiovascular in 11 patients (end-stage chronic HF in eight patients, sudden cardiac death in three patients). The remaining two reasons were infectious complication following abdominal surgery and pancreatic carcinoma. Clinical characteristics of patients are shown in Table 1 .
Variable | Value |
---|---|
Age (years) | 57 ± 9 |
Women | 34 (38%) |
Ischemic etiology of chronic HF | 37 (41.1%) |
BMI (kg/m 2 ) | 26.5 ± 4.3 |
Medications | |
β-blockers | 90 (100%) |
Spironolactone | 76 (84.4%) |
Angiotensin-converting enzyme inhibitors | 79 (87.8%) |
Angiotensin receptor antagonists | 16 (17.8%) |
Loop diuretics | 80 (88.9%) |
Comorbidities | |
Diabetes | 20 (22.2%) |
Arterial hypertension | 41 (45.6%) |
Dyslipidemia | 47 (52.2%) |
LBBB | 79 (88%) |
NYHA class | |
Baseline | 3.26 ± 0.39 (class IV, 32 patients) |
Follow-up | 1.93 ± 0.47 (class IV, 6 patients) ∗ |
QRS duration (msec) | |
Baseline | 176 ± 29 |
Follow-up | 154 ± 45 ∗ |
6-min walking distance (m) | |
Baseline | 313 ± 95 |
Follow-up | 385 ± 103 ∗ |
Peak ventilatory oxygen uptake (mL/kg/min) | |
Baseline | 13.1 ± 3.9 |
Follow-up | 14.6 ± 4.8 ∗ |
NT-proBNP (pg/mL) | |
Baseline | 2,493.5 ± 2,446 |
Follow-up | 1,158 ± 1,235 ∗ |
Echocardiographic Changes During CRT
After 18 months, a significant reduction in interventricular dyssynchrony was noted. Reductions in LV volumes and diameters as well as an improvement in systolic function were observed during follow-up. CRT resulted in a decrease in intra-RV systolic delay and significant improvements in RV fractional area change and tricuspid annular plane systolic excursion ( Table 2 ).
Variable | Baseline | 6 months | 18 months | P (ANOVA) |
---|---|---|---|---|
IVD | ||||
LV PEP (msec) | 183 ± 40 | 189 ± 48 | 190 ± 58 | .78 |
IVD (msec) | 64 ± 22 | 25 ± 21 | 20 ± 15 | <.0001 |
LV remodeling | ||||
Biplane LV EDV (mL) | 261 ± 82 | 230 ± 93 | 228 ± 90 | .13 |
Index (mL/m 2 ) | 140 ± 45 | 117.9 ± 46.3 | 112.7 ± 49 | <.05 |
Biplane LV ESV (mL) | 199 ± 71 | 145 ± 71 | 155 ± 97 | <.05 |
Index (mL/m 2 ) | 106 ± 39 | 76 ± 39 | 81 ± 47 | <.001 |
Biplane EF (%) | 24.6 ± 7.6 | 37.5 ± 12.6 | 31.4 ± 11.5 | <.0001 |
RV changes | ||||
RVIT A4C (cm) | 3.28 ± 0.89 | 3.1 ± 0.82 | 3.32 ± 0.73 | .69 |
RV ED area (cm 2 ) | 21.3 ± 7.8 | 20.8 ± 10.6 | 18.6 ± 5.5 | .73 |
RV ES area (cm 2 ) | 14.8 ± 6.7 | 12.1 ± 5.5 | 11.6 ± 5.4 | .26 |
FAC | 0.31 ± 0.13 | 0.41 ± 0.13 | 0.39 ± 0.15 | <.05 |
TAPSE (cm) | 1.31 ± 0.46 | 1.54 ± 0.47 | 1.61 ± 0.64 | <.05 |
RVSP (mm Hg) | 43.2 ± 20.1 | 40.4 ± 24 | 40 ± 7.6 | .23 |
RV dyssynchrony changes | ||||
RV-S peak IVC VEL (msec) | 18.9 ± 17.9 | 16.3 ± 13.8 | 13.9 ± 9.9 | .60 |
RV-S peak VEL (msec) | 38.2 ± 44.4 | 11.3 ± 15.7 | 13.1 ± 15 | <.0001 |
RV-S peak ϵ (msec) | 175.5 ± 168.6 | 111.1 ± 104.7 | 120 ± 109 | .009 |
Analysis of Response to CRT
At the end of follow-up, patients were classified as being responders or nonresponders according to the aforementioned criteria. If a patient was alive, the data used to determine clinical response data were taken at the 18-month follow-up visit. If a patient died during follow-up, the data used to define echocardiographic response were retrieved from the last available follow-up visit. In terms of the clinical criteria, there were 69 responders (76.6%), and the criterion of reverse remodeling was fulfilled by 64 (71.1%).
The multivariate stepwise regression analysis ( Table 3 , Figure 3 A) identified RV deformation synchrony, RV isovolumic contraction dyssynchrony, interventricular dyssynchrony, and lower Simpson’s biplane end-systolic volume as independent predictors of clinical response. Intra-deformation delay < 54 msec predicted a favorable clinical response with 89% sensitivity and 67% specificity (AUC, 0.82; P < .05; Figure 4 A). Isovolumic contraction dyssynchrony > 26 msec differentiated clinical responders from nonresponders with 85% sensitivity and 100% specificity (AUC, 0.89; P < .05; Figure 4 B).
Variable | β | P | OR (95% CI) | AUC (95% CI) | P |
---|---|---|---|---|---|
Clinical criterion | |||||
Biplane LV EDV (per mL) | 0.158 | .001 | 1.17 (1.06–1.29) | 0.57 (0.41–0.72) | NS |
Biplane LV ESV (per mL) | −0.176 | .001 | 0.84 (0.76–0.93) | 0.65 (0.51–0.78) | <.05 |
RV-S peak IVC VEL (per msec) | −0.82 | .069 | 0.92 (0.85–1.01) | 0.89 (0.79–0.99) | <.05 |
RV-S peak ϵ (per msec) | 0.005 | .011 | 1.01 (0.99–1.02) | 0.82 (0.64–0.99) | <.05 |
IVD (per msec) | −0.061 | .033 | 0.94 (0.89–0.99) | 0.56 (0.41–0.72) | NS |
Reverse remodeling | |||||
Biplane LV EDV (per mL) | 0.054 | .002 | 1.06 (1.02–1.09) | 0.59 (0.45–0.73) | NS |
LV EDD index (per mm/m 2 ) | 0.292 | .002 | 1.34 (1.11–1.61) | 0.69 (0.57–0.81) | <.05 |
RV-S peak VEL (per msec) | 0.035 | .018 | 1.04 (1.01–1.07) | 0.53 (0.38–0.67) | NS |
SD peak ϵ (per msec) | −0.025 | .038 | 0.98 (0.95–0.99) | 0.74 (0.57–0.91) | <.05 |
RVIT A4C (per mm) | 0.360 | .020 | 1.44 (1.06–1.94) | 0.65 (0.48–0.82) | NS |
RV ED area (per cm 2 ) | 0.448 | .012 | 1.56 (1.1–2.2) | 0.52 (0.33–0.71) | NS |


A similar analysis for reverse remodeling revealed that systolic RV synchrony (the shorter the delay, the better) measured by peak velocity delay was an independent predictor of CRT response. However, RV peak velocity synchrony alone was of poor predictive value (AUC, 0.53; P = NS; Table 3 , Figure 3 B).
Two RV morphologic parameters were independent predictors of reverse remodeling: low RV inflow tract diameter (minor RV axis; for 35 mm, sensitivity of 73% and specificity of 58%; AUC, 0.65; P = NS) and low RV end-diastolic area (for 20.9 cm 2 , sensitivity of 62% and specificity of 50%; AUC, 0.52; P = NS).
The LV indices predictive of reverse remodeling were LV end-diastolic index (for 40.4 mm/m 2 , sensitivity of 67% and specificity of 68%; AUC, 0.69; P < .05), the standard deviation of time to peak ϵ in six mid-LV segments (for 98 msec, sensitivity of 75% and specificity of 75%; AUC, 0.74; P < .05). LV end-diastolic volume was a predictor of response according to both criteria, but its value was poor.
Regression and receiver operating characteristic analyses of the predictive value for nonresponse to CRT are presented in Table 3 .
Incremental Value of RV Variables for the Prediction of Response to CRT
According to the clinical criteria, LV indices (end-diastolic and end-systolic volumes) and interventricular delay gave an overall R 2 value of 0.20 (86.2% of patients correctly classified; AUC, 0.80). The addition of RV delay measured in peak ϵ and isovolumic contraction peak velocities significantly increased the power of the model ( R 2 = 0.86; 100% of patients correctly classified; AUC, 1; P for change in R 2 < .001; Figure 5 A).

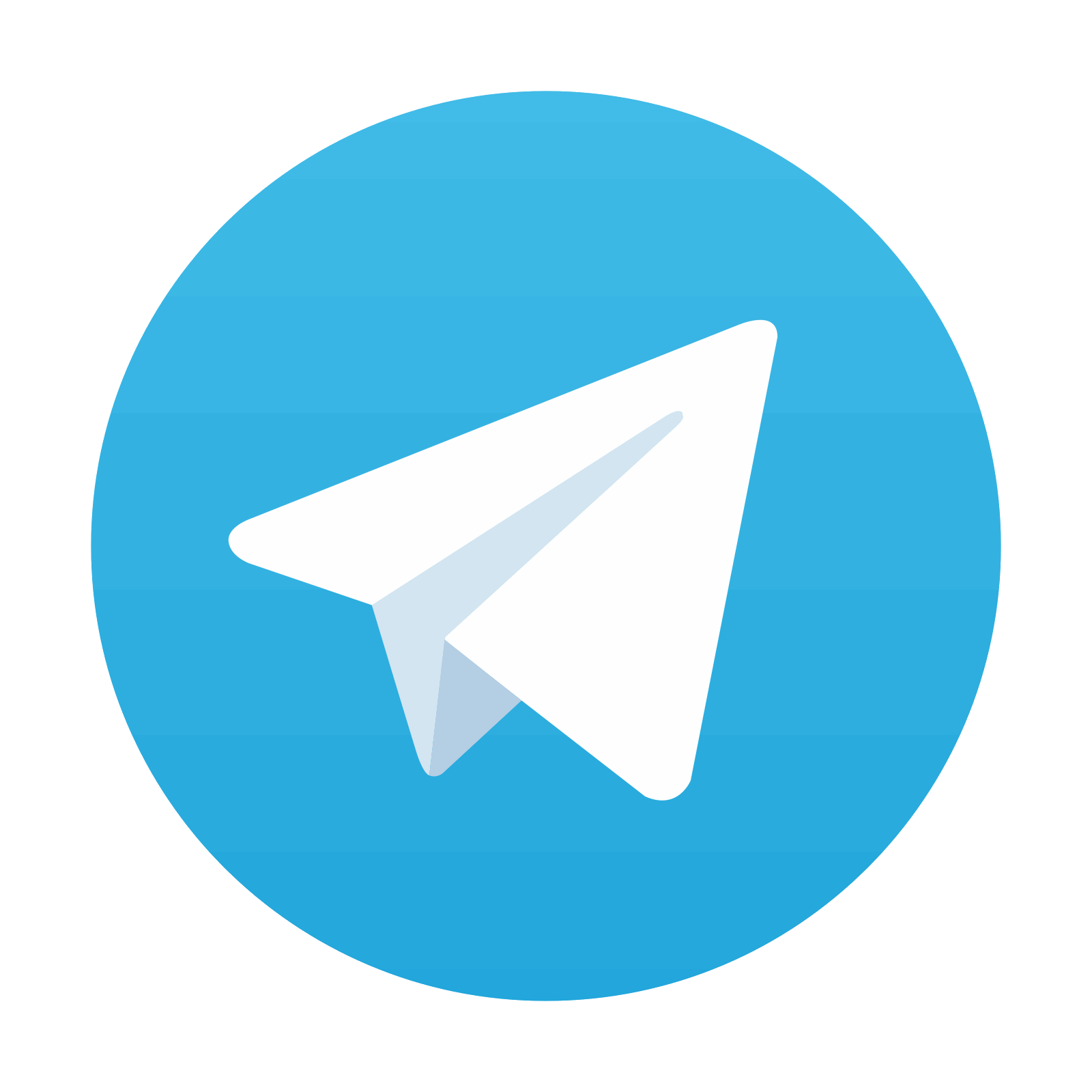
Stay updated, free articles. Join our Telegram channel

Full access? Get Clinical Tree
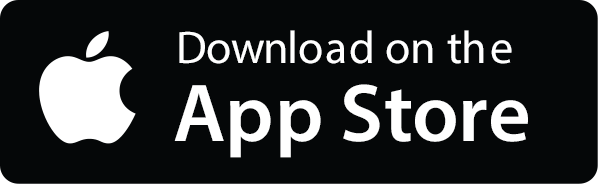
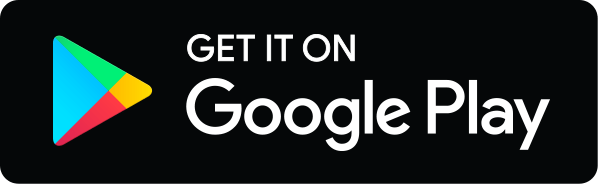
