Fig. 8.1
(a) Pathologic specimen of the right ventricle with the free wall removed to demonstrate the 3 anatomic regions. (b) Pathologic specimen of the heart cut transversely demonstrating the crescent shape of the right ventricle (Reproduced with permission from Warnes [1])
The volume of the right ventricle is larger than the volume of the left ventricle, but right ventricular mass is only 1/6 of the left ventricle [7]. The myocardial fibre arrangement is different from the left ventricle with coarse trabeculations. Contraction force relies heavily on longitudinal shortening [8]. The right ventricular free wall (normally 3–5 mm in thickness) is composed of superficial and deep muscle layers. The fibres of the superficial layer are arranged circumferentially parallel to the atrioventricular groove. On the sternocostal aspect the fibres turn obliquely towards the cardiac apex and continue into the superficial myofibres of the left ventricle. In contrast the deep muscle fibres are longitudinally aligned from base to apex. Continuity between muscle fibres of the right and left ventricles lock the ventricles together [9]. This forms the basis for free right ventricular wall traction caused by left ventricular contraction [10].
Right ventricular contraction is sequential [8]. It starts with the inlet and trabeculated myocardium then ends with infundibular contraction 25–50 m/s later. Infundibular contraction is more prolonged than the inflow region. In contrast to the concentric contractility of the left ventricle, right ventricular contraction causes inward movement of the free wall with a bellows effect. Meanwhile, contraction of the longitudinal fibres shortens the long axis of the ventricle drawing the tricuspid annulus towards the apex. There is also traction on the free wall, secondary to left ventricular contraction. Right ventricular pressure tracings show an early peak then rapidly declining pressure which contrasts with the rounded contour of left ventricular pressure tracings (Fig. 8.2) [11]. Right-sided pressures are significantly lower than comparable left-sided pressures because of the low-impedance, highly distensible pulmonary vascular system. Right ventricular-iso-volumic contraction time is short because the systolic pressure rapidly exceeds the low pulmonary artery diastolic pressure.
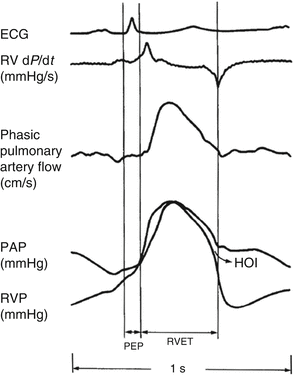
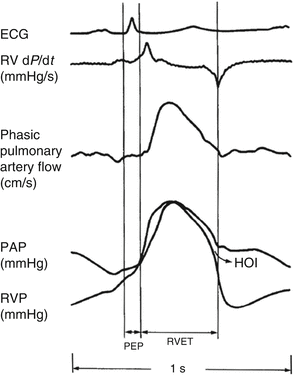
Fig. 8.2
Simultaneously recorded ECG, RV analogue signal of pressure development (dP/dt), phasic pulmonary artery flow, pulmonary artery pressure (PAP) and RV pressure (RVP) in the human subject (Reproduced with permissions from Haddad et al. [4])
Compared with the left ventricle, the right ventricle is more sensitive to afterload change [12]. Under chronic pressure overload there is progressive accumulation of muscle mass and thickness which may exceed that of the left ventricle. In acute right ventricular pressure or volume overload states, dilatation of the right ventricle shifts the intra-ventricular septum towards the left (Fig. 8.3). This alters left ventricular geometry with increased pericardial constraint. Approximately 20–40 % of right ventricular systolic pressure and volume outflow results from left ventricular contraction. Through ventricular interdependence, the interventricular septum is able to maintain right ventricular function in the presence of ischaemia or scarring (as long as the chamber is not dilated) [10]. In turn acute right ventricular dilatation (through pulmonary embolism) causes a decrease in left ventricular elastance [13].
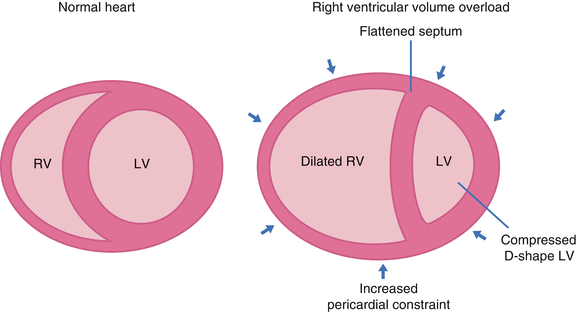
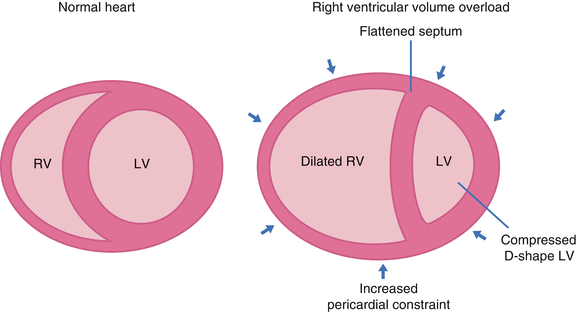
Fig. 8.3
Ventricular interdependence in RV failure. Dilatation of the RV shifts the interventricular septum towards the left, changing LV geometry. Acute RV distension also may lead to an increase in pericardial constraint (arrows). These changes may contribute to low cardiac output state by decreasing LV distensibility, preload and ventricular elastance (Reproduced with permission from Haddad et al. [4])
Right ventricular systolic function is dependent upon contractility, preload and afterload. Pulmonary vascular resistance (PVR) is the most commonly used index of afterload. Right ventricular ejection fraction (RVEF) is the most frequently used index of right contractility but is highly dependent on loading conditions [14]. Because the right ventricular chamber is larger than the left, RVEF under normal conditions is lower than left ventricular ejection fraction (LVEF) [15]. The normal value is around 60 % ranging from 47 to 76 %. Right ventricular myocardial performance index (the ratio of iso-volumic time intervals to ventricular ejection time) is a nongeometric index of global ventricular function [16]. This appears to be independent of preload, afterload and heart rate and is used for assessment of patients with congenital heart disease or pulmonary hypertension [17]. The normal value of this index is 0.28 ± 0.04 and is usually increased in the presence of right ventricular systolic or diastolic dysfunction. Right ventricular filling (preload) is dependent on intravascular volume status, ventricular chamber compliance, ventricular relaxation, heart rate, atrial contraction, left ventricular filling and pericardial constraint. An increase in right ventricular preload improves myocardial contraction through the Frank-Starling mechanism. Excessive right ventricular volume loading can compress the left ventricle and impair function through the ventricular interdependence mechanism [10]. With ageing the pulmonary artery pressure and vascular resistance increase through changes in arterial stiffness of the pulmonary vasculature. Right ventricular ejection fraction remains well preserved.
Right ventricular myocardium is resistant to ischaemic injury through its lower oxygen consumption, ability to increase oxygen extraction and extensive collateral circulation [18]. A dominant right coronary artery is found in 80 % of the population (whereby the vessel supplies most of the right ventricle) [8]. The lateral wall is supplied by the marginal branches, whilst the posterior wall and infero-septal region are perfused by the posterior descending artery. The infundibulum receives its supply from a conal artery which has a separate origin in 30 % of cases. This explains preservation of infundibular contraction when the right coronary is occluded proximally. The left anterior descending branch of the left coronary artery supplies part of the anterior wall and antero-septal part of the right ventricle. A branch of the first septal perforator (from the left anterior descending coronary) may perfuse the right ventricle via the moderator band artery.
8.3 The Right Ventricular Response to Pressure and Volume Overload
Patients with congenital heart disease may present with a pressure or volume overloaded right ventricle in a biventricular or single ventricle circulation (when the morphological right ventricle serves as systemic ventricle) [19, 20]. Right ventricular failure is a complex clinical syndrome triggered by any structural or functional disorder that impairs the ability of the right ventricle to eject blood. Right ventricular dysfunction is the key determinant of outcome in various congenital anomalies including tetralogy of Fallot, transposition of the great arteries (TGA), Ebstein anomaly and Eisenmenger syndrome (Table 8.1). In clinical practice the commonest causes of right ventricular dysfunction are chronic left-sided heart failure and pulmonary hypertension [21–23]. In turn the causes of pulmonary hypertension can be separated into those that effect primarily the pulmonary arterial tree, the pulmonary venous system or the pulmonary micro-vasculature as a result of lung disease or embolisation [23]. The sequelae of right ventricular failure include peripheral oedema, ascites and anasarca. Failure to maintain blood flow through the lungs results in decreased systolic reserve and low cardiac output state. This results in exercise intolerance and fatigue. Right atrial and ventricular distensions predispose to dysrhythmia.
Table 8.1
Congenital causes of RV failure
Pressure overload |
Left-sided heart failure |
RV outflow tract obstruction |
Peripheral pulmonary stenosis |
Double-chambered RV |
Systemic RV |
Volume overload |
Tricuspid regurgitation |
Pulmonary regurgitation |
Atrial septal defect |
Anomalous pulmonary venous return |
Sinus of Valsalva rupture into the RA |
Coronary artery fistula to RA or RV |
Myocardial ischaemia |
Intrinsic myocardial process |
Cardiomyopathy |
Arrhythmogenic RV dysplasia |
Sepsis |
Inflow limitation |
Tricuspid stenosis |
Superior vena cava stenosis |
Complex congenital defect |
Ebstein anomaly |
Tetralogy of Fallot |
Transposition of the great arteries |
Double-outlet RV with mitral atresia |
Adaptation to pressure or volume overload is a complex process. It depends upon the type and severity of myocardial injury, the time course of the disease (acute or chronic) and the time of onset (whether in the newborn, paediatric or adult years) [23]. The right ventricle adapts better to volume overload than to pressure overload. For example, with atrial septal defect or tricuspid regurgitation, the ventricle will tolerate volume overload for many years without a significant decrease in systolic function [24, 25]. Nevertheless, volume overload will eventually contribute to morbidity and mortality. Through the ventricular interdependence mechanism, right ventricular dilatation also leads to left ventricular dysfunction. After repair of tetralogy of Fallot, severe pulmonary regurgitation will cause right ventricular dilatation and dysfunction at an earlier stage [26]. Right ventricular dilatation predisposes to arrhythmias, heart failure and sudden cardiac death during the second and third decades [27]. As a result many centres now recommend early pulmonary valve replacement before the first symptoms of heart failure develop [28]. When right ventricular end diastolic volume exceeds 170 ml/m2 or end systolic volume exceeds 85 ml/m2, right ventricular dilatation and dysfunction persist even after pulmonary valve replacement [29]. Declining contractile function is associated with altered expression of genes which regulate mitochondrial and G protein-coupled receptor signalling [23, 30].
The morphology of the right ventricle enables early adaptation to high pressure [23]. As long as sinus rhythm persists and there is no volume overload, the hypertrophied pressure-loaded right ventricle functions well into the fourth or fifth decades [12, 31]. Mechanical stress induces intracellular signalling to stimulate synthesis of contractile proteins [32]. Cardiomyocyte protein synthesis is directly introduced by stretch and reinforced through autocrine, paracrine and neurohumeral factors (the renin-angiotensin system) [33]. The increase in afterload is sensed by integrins and stretch activated ion channels in myocytes, fibroblasts and endothelial cells [34]. The integrins are firmly attached to the extracellular matrix and cytoskeleton allowing transduction of mechanical stress into intracellular chemical signals relevant for the synthesis of contractile proteins. They also induce changes at the transcriptional level including an increase of protein synthesis and alterations of foetal genes [35]. Wall thickness increases by accumulating muscle mass and assuming a more rounded shape.
During hypertrophy a mismatch between the numbers of capillaries versus the increased size of the cardiomyocytes may eventually result in hypoxia, apoptosis, fibrosis and contractile dysfunction [36]. In this way pressure overload can eventually lead to systolic and diastolic right ventricular dysfunction. Compared with volume overload, there is more pronounced histological change in pressure overloaded states [37]. This is confirmed by the increased density of myocardial connective tissue (fibrosis) in the extracellular matrix. Pulmonary hypertension and obstructive pressure overload both cause right ventricular dilatation and failure, but some patients deteriorate earlier than others with the same degree of increased afterload [38, 39]. Genetic and neurohormonal responses determine this response. Patients with idiopathic pulmonary hypertension show re-expression of the myocardial foetal gene programme. This results in decreased expression of the alpha-myosin heavy chain gene and enhanced expression of the foetal beta-myosin heavy chain [32].
In the presence of systemic afterload, the right ventricle changes its myofibre architecture to resemble the circumferential “sandwich” pattern of the left ventricle. This results in predominant circumferential rather than longitudinal free wall shortening. Whilst the prognosis for a systemic right ventricle depends largely upon the severity of associated lesions, patients with congenitally corrected TGA may survive until the seventh or eighth decade [40]. The propensity for heart failure increases with age with one third of patients experiencing symptoms by the fifth decade [41]. Emergence of tricuspid regurgitation or right ventricular dysfunction portend worse prognosis. Progression in systemic right ventricular dysfunction is then more rapid than for left ventricular dysfunction in the presence of mitral regurgitation. This is probably the result of increased tricuspid annular dilatation. Right coronary artery perfusion may also prove inadequate to sustain severely hypertrophied myocardium resulting in ischaemia and fibrosis [42].
Two conditions where chronic pressure overload is well tolerated are Eisenmenger syndrome and congenital pulmonary stenosis [43]. The right ventricle fails late in Eisenmenger syndrome despite long-standing supra-systemic pressure in the pulmonary artery [31, 44]. Survival is 80 % at 10 years, 77 % at 15 years and 42 % at 25 years. Preserved right ventricular contractility is associated with persistence of the foetal phenotype. There is similar right and left ventricular wall thickness throughout life and a right to left shunt to the systemic circuit. This balances the pulmonary and systemic vascular resistance changes during exercise. Similarly in pulmonary valve stenosis, the degree of right ventricular hypertrophy adapts so that symptomatic right ventricular failure is unusual before adult life. The eventual onset of tricuspid regurgitation will precipitate right ventricular failure [45].
The autonomic nervous system, the renin-angiotensin-aldosterone system, natriuretic peptides, endothelin and cytokines all play a role in the development of right heart failure [23]. In pulmonary hypertensive patients elevated catecholamine levels increase pulmonary vascular resistance and lower cardiac index and exercise capacity. Right ventricular pressure overload can also decrease responsiveness to the inotropic effects of angiotensin II [46]. Excessive sympathetic adrenergic stimulation adversely affects ventricular remodelling and survival. As in the failing left ventricle, decreased beta-adrenergic receptor density occurs in the stressed right ventricle. In patients with pulmonary hypertension and some forms of congenital heart disease, elevated endothelin-I levels are associated with decreased exercise capacity and impaired ventricular contractility [47, 48]. Beta-type natriuretic peptide levels increase in both pressure and volume overload states and are associated with increased risk of mortality.
8.4 Symptoms and Prognosis
In patients with congenital heart disease decreased exercise tolerance associated with pulmonary hypertension is an important prognostic factor for death or hospitalisation [23]. Equally for patients with left heart failure, RVEF is a strong independent predictor of mortality [49, 50]. Factors including right ventricular systolic dysfunction, tricuspid regurgitation, ventricular interdependence, suboptimal preload and dysrhythmia may all contribute to low cardiac output state. Right ventricular diastolic dysfunction impairs filling and increases diastolic right atrial and ventricular pressure [51]. In turn significant tricuspid regurgitation will aggravate right ventricular volume overload and decrease cardiac output. These patients with elevated systemic venous pressure develop abdominal discomfort (through hepatic distension), ascites (through hepatic venous hypotension) and leg swelling. They may eventually develop cirrhotic change and venous ulceration. In the presence of an atrial septal defect or patent foramen ovale, diastolic dysfunction and tricuspid regurgitation promote right to left shunting and systemic hypoxaemia. They also predispose to paradoxical embolism and stroke. In the presence of right ventricular dilatation with increasing pericardial constraint, the left ventricle becomes compressed into a D shape with impaired filling (Fig. 8.3) [23].
The prognosis of right ventricular failure is dependent upon the underlying cause. Those with volume overload, pulmonary stenosis or Eisenmenger physiology often have the best long-term prognosis [43, 52]. Other prognostic factors include the severity of right ventricular systolic dysfunction, right ventricular diastolic dysfunction, the degree of neurohormonal activation and the presence of left ventricular systolic dysfunction (Table 8.2) [23]. Right ventricular ejection fraction constitutes an independent predictor of mortality in left heart failure [8]. Although the ventricle tolerates chronic volume overload, some patients with an isolated large atrial septal defect will develop Eisenmenger syndrome in later life. Pulmonary regurgitation in repaired tetralogy of Fallot is the commonest cause of right ventricular dilatation and dysfunction. For these patients pulmonary valve replacement should be considered before symptomatic onset and risk of sudden death [28]. In Ebstein anomaly right ventricular failure stems primarily from volume overload and a hypoplastic ventricular chamber which is incapable of handing systemic venous return [53]. An associated atrial septal defect allows bidirectional shunting and cyanosis. Again surgical correction is indicated in symptomatic patients [54]. The methods depend upon tricuspid valve morphology and the size of the functional ventricle [55].
Table 8.2
Markers of RV dysfunction associated with prognosis
Systolic performance indexes |
RVEF |
RV fractional area change |
TAPSE |
RV myocardial performance index |
Haemodynamics |
RA pressure |
Cardiac index |
Diastolic filling profiles |
Tissue Doppler indexes |
Iso-volumic acceleration |
Systolic and diastolic myocardial velocities |
Right-sided dilatation |
RV dilatation |
RA dilatation |
Tricuspid regurgitation |
Electrophysiological characteristics |
Arrhythmias |
Inducibility of ventricular tachycardia |
QRS duration |
Neurohormones and cytokines |
B-type natriuretic peptide |
Norepinephrine |
Endothelin |
Tumour necrosis factor |
Right ventricular outflow tract obstruction with pressure overload occurs in pulmonary valve stenosis, double-chambered right ventricle and unoperated tetralogy of Fallot. The hypertrophic response can compensate for right ventricular outflow obstruction for many years even when severe [23]. In adult life symptoms of fatigue and dyspnoea ensue through inability to elevate cardiac output during exercise. Progressive tricuspid regurgitation will eventually lead to symptomatic right ventricular failure.
Perhaps the greatest therapeutic challenge is in the management of the morphological right ventricle which supports the systemic circulation. The right ventricle is the systemic ventricle in congenitally corrected TGA, in patients with D-TGA following atrial repair with the Senning or Mustard techniques and in others with univentricular heart of right ventricular predominance [56, 57]. These include patients following Fontan-type total cavopulmonary connection. For these patients, right ventricular function is the major determinant of morbidity and mortality with worse outlook than for the morphological left ventricle.
8.5 Investigation and Management of the Pressure Overloaded Right Heart
8.5.1 Pulmonary Valve Stenosis
Isolated congenital pulmonary valve stenosis accounts for between 80 and 90 % of patients with a pressure-loaded right ventricle (Fig. 8.4) [58]. The commonest type is dome shaped with no separation into valve cusps. The valve is relatively thin with a narrow central opening. In contrast the dysplastic pulmonary valves found in 10–15 % of patients are trileaflet with thickened, immobile myxomatous cusps often associated with a hypoplastic ventriculoarterial junction. This morphology is common in Noonan’s syndrome [59]. Long-standing pulmonary stenosis causes post-stenotic dilatation of the pulmonary artery together with right ventricular hypertrophy which may cause sub-valvar stenosis. The hypertrophied right ventricle functions well for many years with near systemic intra-cavity pressure as long as sinus rhythm is preserved. Even in the presence of severe pulmonary stenosis, most patients remain asymptomatic. The condition is often detected through a systolic murmur on physical examination followed by transthoracic echocardiography. On auscultation the mobile pulmonary valve creates an ejection click which decreases with inspiration. The ejection systolic murmur is maximal at the upper left sternal border. If a third heart sound is detected, an atrial septal defect should be considered. Symptoms may develop on exercise in the fourth or fifth decade of life. These include chest pain, syncope and even sudden cardiac death through ischaemia or ventricular arrhythmias. When a murmur is detected, the electrocardiogram may show evidence of right atrial enlargement, right axis deviation and right ventricular hypertrophy. In severe pulmonary stenosis, the R wave is usually greater than 20 mm. Echocardiography is then the diagnostic method of choice, colour and pulsed wave Doppler are used to define the site of obstruction and distinguish between valvular, sub-valvar and supra-valvar obstruction. Echocardiography will also define right ventricular size and function and the presence of tricuspid regurgitation or post-stenotic dilatation in the pulmonary artery. Cardiac magnetic resonance imaging will define the same features but with improved definition of ventricular morphology and function [60].
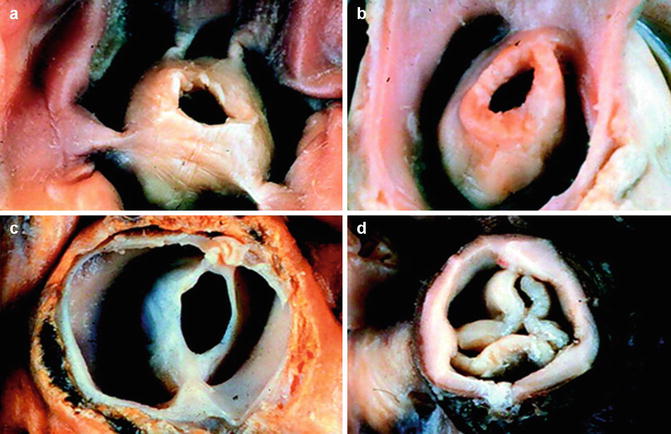
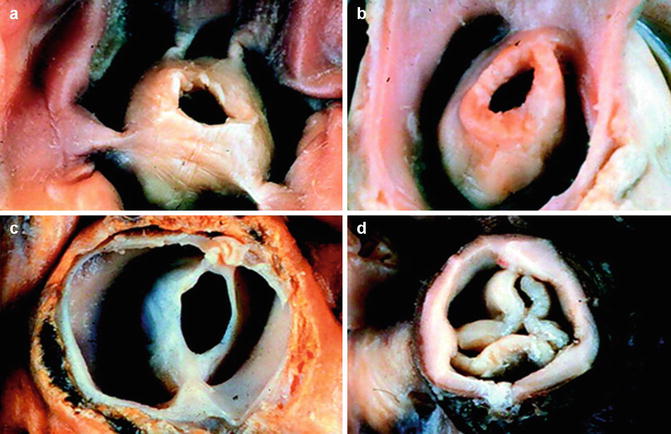
Fig. 8.4
Pulmonary stenosis: The abnormal PV may be classified as acommisural (a) with prominent systolic doming of the valve cusps and an eccentric orifice, unicommissural (b), bicuspid with fused commissures (c) or dysplastic (d) with severely thickened and deformed valve cusps (Reproduced with permission from Bruce and Connolly [93])
In general surgical intervention is offered to patients who are symptomatic with dyspnoea, fatigue or dysrhythmia and to those with a peak gradient exceeding 50 mmHg. Of those operated in infancy or childhood, one third will develop significant pulmonary regurgitation requiring late pulmonary valve replacement (around 30 years later) [43]. Whilst a dysplastic valve requires excision, percutaneous balloon valvotomy has become the treatment of choice for domed valvular pulmonary stenosis [61]. Long-term outcome is then excellent with a low rate of re-stenosis or significant pulmonary regurgitation (5 %).
8.5.2 Double-Chambered Right Ventricle
Doubled-chambered right ventricle is characterised by hypertrophied muscle bands that separate the right ventricular cavity into a proximal high pressure and a distal low pressure chamber [62]. There is usually an associated ventricular septal defect which may dominate the clinical signs. Echocardiography is diagnostic, defining the location and degree of the obstructive bands and the site of the ventricular septal defect. However, whilst echo is the imaging modality of choice, for the ellipsoid left ventricle quantitative two-dimensional echocardiographic measurement of left ventricular performance is based on geometric assumptions that do not apply in the right ventricle. The right ventricle must be imaged in multiple planes though the apical four chamber view gives best information on the right atrium, tricuspid annulus and right ventricular morphology. Echocardiography provides accurate information with regard to the presence of inter-atrial or ventricular septal defects together with situs and atrioventricular-ventriculoarterial connections. Identification of right ventricular morphology is best determined by examining the atrioventricular valves. The tricuspid valve is always closer to the apex compared to the mitral valve, and its septal leaflet has chordal attachments to the inlet septum.
Surgical correction involves excision of the obstructing muscle bands either through the tricuspid valve or via right ventriculotomy. Associated lesions, predominantly VSD, are dealt with simultaneously [62].
8.5.3 Congenitally Corrected Transposition
Congenitally corrected TGA is rare accounting for <1 % of all cases of congenital heart disease [57]. Through atrioventricular and ventriculoarterial discordance, the right atrium is connected to the morphological left ventricle (Fig. 8.5). This gives rise to the pulmonary artery. In contrast the left atrium is connected to the morphological right ventricle which gives rise to the aorta. The systemic ventricle shows coarse trabeculation, a moderator band and a morphological tricuspid valve. There is mitral valve in the right heart. Usually the aorta is anterior and to the left, or the great arteries may be side by side. The condition is commonly associated with dextrocardia and additional lesions are present in 95 % of patients [63]. These include Ebstein anomaly (90 %), ventricular septal defect (70 %) and pulmonary stenosis (40 %). These patients often present with dyspnoea on exertion or palpitations in the third or fourth decade of life. Cyanosis may occur in those with ventricular septal defect and pulmonary stenosis. Again echocardiography is the diagnostic method of choice. Assessment of systemic right ventricular dysfunction and valve regurgitation is important together with identification of a perimembranous ventricular septal defect or left ventricular outflow tract obstruction. The prognosis depends on the presence and severity of these associated lesions. When the systemic (tricuspid) atrioventricular valve becomes regurgitant, ventricular function deteriorates progressively with symptomatic onset within 5 years. This is the main determinant of prognosis. Tricuspid valve replacement should then be considered to prevent progressive overload and irreversible systemic right ventricular dysfunction. Coronary flow reserve is decreased in these patients and coronary anomalies are common with perfusion defects at rest [42]. There is a propensity to develop complete heart block and sudden dysrhythmic death.
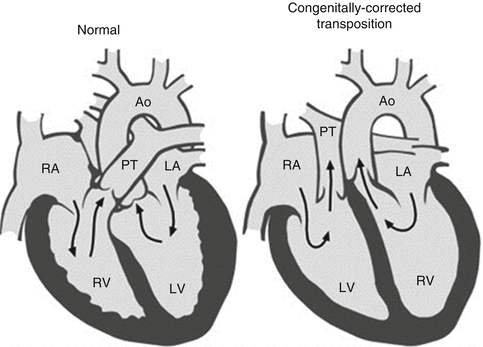
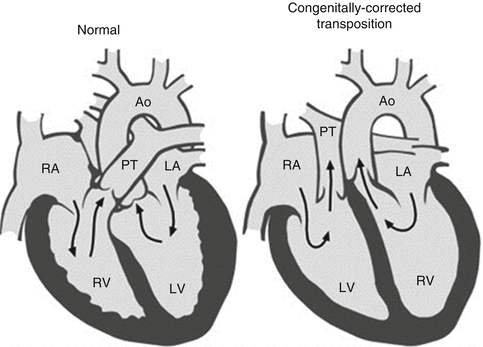
Fig. 8.5
Congenitally corrected transposition. Schematic of congenitally corrected transposition (right) in which there is both atrioventricular and ventriculoarterial discordance (Reproduced with permission from Warnes [1]). RA right atrium, RV right ventricle, PT pulmonary trunk, Ao aorta, LA left atrium, LV left ventricle
8.5.4 Complete Transposition of the Great Arteries
This condition accounts for 5 % of newborns with a congenital cardiac anomaly. In TGA the aorta arises from the morphological right ventricle and the pulmonary artery from the morphological left ventricle. The great vessels are parallel with the aorta anterior in front of the pulmonary artery. Associated lesions, commonly ventricular septal defect, are present in one third. Whilst the contemporary treatment is the arterial switch procedure, a number of adult patients with TGA underwent an atrial switch procedure (Mustard or Senning operation) which resulted in the morphological right ventricle supporting the systemic circulation. Late after an atrial switch operation, uncoordinated myocardial contraction, myocardial perfusion defects and failure of the morphologically tricuspid systemic atrioventricular valve contribute to deterioration [64, 65]. Dysrhythmia (atrial flutter and sick sinus syndrome) compromises quality of life and survival. The decline in right ventricular function together with residual lesions such as baffle obstruction or leak, residual ventricular septal defect or pulmonary valve stenosis also contributes to reduced exercise capacity and late mortality. Deficiencies in myocardial perfusion are not through classic coronary artery disease [66]. They represent a supply/demand ischaemia in the context of severe right ventricular hypertrophic response to systemic pressure loading. Incoordinated myocardial contraction in the right ventricle is highly sensitive to ischaemia, and regions of abnormal myocardium with fibrosis are apparent on magnetic resonance imaging with late gadolinium enhancement [67]. These fibrotic areas correlate with increased right ventricular mass and dilatation indicating that ischemic fibrosis develops within the severely hypertrophied myocardium with a resulting impairment of right ventricular systolic performance [68]. Fibrosis is also associated with prolonged QRS duration and arrhythmia as markers of adverse outcome. Atrial scarring following the Mustard or Senning operation creates a substrate for atrial arrhythmias which may become life threatening in the presence of baffle obstruction [56]. Superior vena cava obstruction is common though the azygos system will compensate for this. Sinus node dysfunction is common so that 10–15 % of patients will require pacemaker implantation. Insertion of a transvenous pacing system can be complicated by superior vena caval or baffle obstruction. Pulmonary hypertension develops in around 7 % of patients after atrial switch procedures often secondary to pulmonary venous pathway obstruction. Baffle leaks may allow shunting with cyanosis or paradoxical emboli.
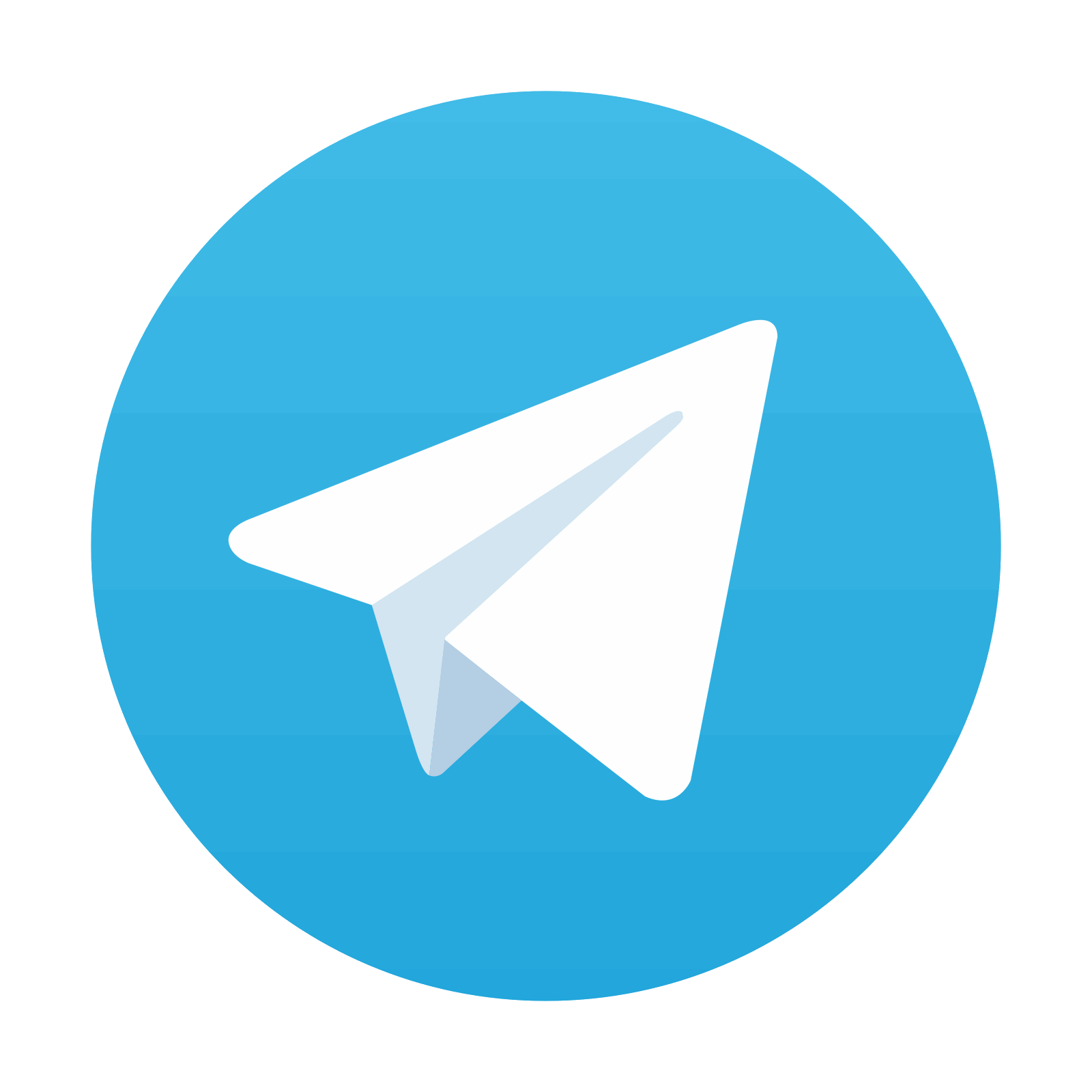
Stay updated, free articles. Join our Telegram channel

Full access? Get Clinical Tree
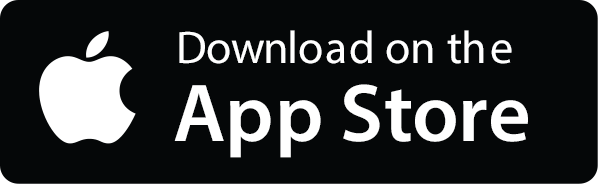
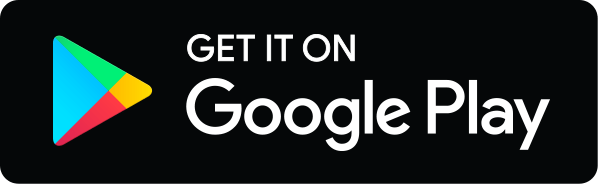