Ventricular Tachyarrhythmias and Sudden Death
Sudden death (death that is instantaneous and completely unexpected) may well be the major public health problem in the United States today. If this statement seems surprising, consider that each year, approximately 400 000 Americans die suddenly, and that sudden death is the most common form of death in the United States. Although most Americans are touched by sudden death, few realize that the majority of these deaths are due to electrical disturbances of the heart and are therefore, at least in theory, preventable.
Consider a typical sudden cardiac death: a 59-year-old man who has suffered a myocardial infarction within the past 12 months has recovered, is now feeling well, and is leading a happy and productive life. He has followed his physician’s advice and has altered his lifestyle in an exemplary fashion—he has stopped smoking, is on a low-fat and low-cholesterol diet, and has joined an exercise program. He is back at work, and his new sense of mortality has resulted in a broader and more tolerant perspective on everyday job-related stresses. In many ways, he feels better than he has in years.
Then one day, while watching television with his wife, he gasps softly and slumps over. An ambulance is called, but within minutes he is dead. The grieving widow is told by the emergency room physician (who has never seen the victim before) that her husband has died of a heart attack. Later, the victim’s personal physician is only too quick (often through the honest desire to allay any feelings of guilt among surviving family members) to corroborate the “inevitability” of the event.
This scene takes place, on average, almost once every minute in the United States. The tragedy is compounded by a lack of understanding by both the lay public and the medical profession as to the reason for these sudden deaths. Most often, sudden death is not caused by acute myocardial infarction or bradycardia, as is commonly claimed. Instead, the vast majority of the 400 000 sudden deaths that occur each year in the United States are due to ventricular tachycardia or ventricular fibrillation. To the extent that ventricular tachyarrhythmias can be adequately treated, most of these sudden cardiac deaths can be prevented.
During the past 2 or 3 decades, remarkable advances have been made in treating ventricular tachycardia and fibrillation, largely thanks to what has been learned in the electrophysiology laboratory. It was the recognition that the great majority of lethal arrhythmias are due to the mechanism of reentry, and that they therefore lend themselves nicely to study in the electrophysiology laboratory, that catalyzed the rapid expansion of electrophysiology centers during the 1980s. The original “Holy Grail” for electrophysiologists was to solve the problem of sudden cardiac death.
This goal was pursued for years through the careful study of ventricular tachyarrhythmias in the electrophysiology laboratory. For almost 20 years, electrophysiologists spent much of their time inducing and terminating ventricular arrhythmias, and most especially, trying to identify effective therapy for those arrhythmias by the serial testing of antiarrhythmic drugs.
Thankfully, serial drug testing for ventricular arrhythmias now has virtually disappeared from the electrophysiologist’s repertoire. So, in contrast to early editions of this book, where much space in this chapter was devoted to the technique of, and rationale for, serial drug testing, the author now offers instead a chilling speculation on how future historians of medicine might view these recent times:
The reader no doubt will be disturbed to learn that, during the last decades of the 20th century, doctors calling themselves “electrophysiologists” submitted thousands of patients to multiple inductions of cardiac arrest, followed by resuscitations, over periods of days to weeks; and further, that this practice was not only legal, but was represented as being therapeutic, and was accepted—and in some instances honored and admired—by their medical colleagues. It is not recorded what these “electrophysiologists” did to sublimate their proclivities once this “serial drug testing” was no longer considered a legitimate medical practice.
In this chapter, we will review the current management of ventricular tachyarrhythmias—and the role that electrophysiologic testing plays in their evaluation and treatment. This role is clearly very different today than it was in the recent past. Nonetheless, the electrophysiology study can still be helpful in managing patients with ventricular tachyarrhythmias, both in diagnosing their problem and in devising optimal treatment. And, despite the smug superiority that (the author fears) may color future historians’ view of the matter, it is what we have learned in the electrophysiology laboratory that enables our treatment of these arrhythmias today and (if we allow ourselves to follow the science) opens a path to substantially reducing the millions of sudden deaths that threaten to occur in the future.
The Evaluation of Ventricular Tachyarrhythmias
The Mechanisms of Ventricular Arrhythmias
The appropriate evaluation and treatment of ventricular arrhythmias largely depends on the mechanism of those arrhythmias. Table 7.1 lists the most common ventricular arrhythmias according to their mechanisms. Whatever the mechanism, however, ventricular arrhythmias have similar appearances on the ECG: premature ventricular complexes (PVCs), ventricular tachycardia, or ventricular fibrillation. Figuring out which mechanism is responsible when faced with any of these arrhythmias depends to a great extent on the clinical setting in which the arrhythmia occurs—and sometimes on its characteristics in the electrophysiology laboratory.
Table 7.1 Mechanisms of ventricular arrhythmias.
Automatic ventricular arrhythmias |
• Premature ventricular complexes |
• Ventricular tachycardia and fibrillation associated with acute medical conditions: |
° Acute myocardial infarction or ischemia |
° Electrolyte and acid–base disturbances, hypoxemia |
° Increased sympathetic tone |
Reentrant ventricular arrhythmias |
• Premature ventricular complexes |
• Ventricular tachycardia and fibrillation associated with chronic heart disease: |
° Previous myocardial infarction |
• Cardiomyopathy (including bundle branch reentry and ventricular arrhythmias associated with arrhythmogenic right ventricular dysplasia) |
Triggered activity |
• Pause-dependent triggered activity |
• Catechol-dependent triggered activity |
Miscellaneous ventricular arrhythmias |
• Idiopathic left ventricular tachycardia |
• Outflow tract ventricular tachycardia (repetitive monomorphic ventricular tachycardia) |
• Brugada syndrome |
• Catecholaminergic polymorphic ventricular tachycardia |
We will discuss these arrhythmias in the order of their clinical frequency, beginning with reentrant ventricular arrhythmias.
Reentrant Ventricular Arrhythmias
Reentry accounts for the majority of ventricular arrhythmias. Reentrant ventricular arrhythmias are most often associated with chronic underlying heart disease. Reentrant circuits within the ventricles usually do not appear until patients develop some form of heart disease which causes scarring in the ventricular myocardium. Such scarring mainly occurs with ischemic heart disease and the various cardiomyopathies. In ischemic heart disease, reentrant circuits arise during the healing and ventricular remodeling that follow an acute myocardial infarction—usually, the reentrant circuits form in the border zone between the scar tissue and the normal myocardium. In contrast to automatic arrhythmias, in which the typical substrate (such as acute ischemia) is temporary in nature and most often reversible, in the case of reentrant arrhythmias the substrate (i.e. the reentrant circuit) is not temporary but fixed. Once a reentrant circuit is formed, it is always present and can generate a reentrant ventricular tachyarrhythmia at any time and without warning. Thus, the “late” sudden deaths that occur in patients with myocardial infarction (i.e. sudden death occurring at a time between roughly 24 hours and months or even years after the acute infarction) are usually due to reentrant arrhythmias. Reentrant arrhythmias, then, are commonly seen in patients who have a history of cardiac disease but are not acutely ill at the time of the arrhythmia. The vast majority of sudden cardiac deaths in the United States are due to reentrant ventricular tachyarrhythmias.
Risk Factors For Reentrant Ventricular Arrhythmias
It is relatively straightforward to predict which patients are at risk for reentrant ventricular tachyarrhythmias (and, therefore, at risk for sudden cardiac death), as long as one has an understanding of the pathophysiology of reentrant arrhythmias. A reentrant arrhythmia requires both an anatomic circuit with electrophysiologic properties appropriate for sustaining a reentrant impulse and an appropriately timed premature impulse to trigger the reentrant arrhythmia.
Because reentrant circuits are common only in the setting of myocardial disease, the major risk factor for ventricular reentry is the presence of underlying cardiac disease. As noted, myocardial infarctions and cardiomyopathic diseases are the most common disorders associated with reentrant ventricular arrhythmias. Nonetheless, any cardiac condition that causes even a small amount of ventricular fibrosis can give rise to reentrant circuits. Such conditions include myocardial trauma, a myocardial infarction that has been “aborted” by thrombolytic therapy, subclinical infarctions associated with bypass surgery, and subclinical viral myocarditis.
In general, the more extensive the myocardial fibrosis, the higher the likelihood of developing a reentrant circuit. With disease states that cause large fibrotic patches (such as a myocardial infarction), reentrant circuits are reasonably likely to develop. In disease states that cause only microscopic fibrosis, however, the likelihood of reentrant arrhythmias is proportional to the degree of myocardial involvement in the underlying disease process.
Once an anatomic circuit exists whose electrophysiologic properties are appropriate for sustaining reentry, an appropriately timed premature impulse is required to trigger the reentrant arrhythmia. Thus, another risk factor for developing reentrant ventricular arrhythmias is ventricular ectopy. Complex ventricular ectopy is generally considered to be present if, on 24-hour Holter monitoring, there are more than 10 PVC complexes per hour, or repetitive forms such as couplets, triplets, or runs of nonsustained ventricular tachycardia. Patients with underlying cardiac disease who have complex ventricular ectopy have a higher risk of sudden death than patients who have the same underlying disease without complex ectopy. Frequent ectopic beats are not, however, a requirement for developing reentrant arrhythmias, since a single PVC (or even a premature atrial complex (PAC)) has the potential to trigger a reentrant ventricular tachyarrhythmia, given the right reentrant circuit. Indeed, a substantial proportion of patients resuscitated from lethal arrhythmias have only negligible ventricular ectopy. It should also be noted that complex ventricular ectopy in patients with normal heart muscles (who are therefore extremely unlikely to have a reentrant circuit within their ventricles) has never not been shown to increase the risk of sudden death.
An individual’s risk for sudden cardiac death from a reentrant tachycardia, therefore, is most directly dependent on the presence or absence of underlying myocardial disease and on the extent of that disease. In general, since lower ejection fractions imply more extensive scarring and therefore indicate a higher likelihood that a reentrant circuit is present, the lower the ejection fraction, the higher the probability of sudden death. Most fatal arrhythmias occur in patients who have left ventricular ejection fractions of less than 40%. As we have noted, some degree of ectopy must also be present to trigger the reentrant arrhythmia, but that ectopy does not necessarily have to be very frequent for an arrhythmia to occur. The probability that a reentrant circuit will generate an arrhythmia is much more dependent on the characteristics of the circuit itself (e.g. the tachycardia zone of the circuit) than on the frequency of ectopic beats.
Many studies have been done to attempt to quantify an individual’s risk of sudden death, and most have focused on three risk factors: the presence of a prior myocardial infarction, the presence of a depressed left ventricular ejection fraction (arbitrarily, less than 40%), and the presence of complex ventricular ectopy. At the risk of greatly oversimplifying the vast body of literature examining this issue, let us make the following generalizations. First, the presence of underlying heart disease is more important in determining risk than the presence of complex ectopy, because underlying heart disease alone increases one’s risk for sudden death, whereas complex ectopy alone does not. Second, having one of these risk factors alone (except for complex ectopy) yields a 1-year risk of sudden death that can be grossly estimated at approximately 5%. Third, the risk entailed by the presence of more than one of these risk factors appears to be roughly additive (Table 7.2). Thus, the presence of either a previous myocardial infarction or a depressed ejection fraction gives a 1-year risk of about 5%. The presence of any two risk factors gives a risk of about 10%, and the presence of all three factors gives a risk of about 15%. Obviously, these values represent only a gross estimate derived from the available literature. The severity of each risk factor is also important. For instance, a large myocardial infarction yields a higher risk than a small infarction, an ejection fraction of 15% yields a higher risk than an ejection fraction of 35%, and the presence of nonsustained ventricular tachycardia yields a higher risk than a single PVC. Thus, a patient with a previous large myocardial infarction with severely depressed ventricular function and long runs of nonsustained ventricular tachycardia will have a 1-year risk substantially higher than 15%.
Table 7.2 Risk factors and probabilities of sudden cardiac death.
Moderate-risk group | |
Risk factors | 1-year risk of sudden death |
Previous MI or LV EF < 40% | 50% |
Previous MI + LV EF < 40% or previous MI + complex ectopy or LV EF < 40% + complex ectopy | 10% |
Previous MI + LV EF < 40% + complex ectopy | 15% |
High-risk group | |
Risk factors | 1-year risk of sudden death |
Sudden-death survivor | 30–50% |
VT with syncope | 30–50% |
VT with minimal symptoms | 20–30% |
Values on this table are loosely derived from data reported by the Multicenter Post-infarction Research Group (Bigger JT et al., Circulation 1984; 69:250) and the Multicenter Investigation of the Limitation of Infarction Size (Mukharji J et al., Am J Cardiol 1984; 54:31). MI, myocardial infarction; LV EF, left ventricular ejection fraction; VT, ventricular tachycardia. |
Another indicator that is sometimes used to estimate risk of reentrant ventricular arrhythmias is the signal-averaged surface ECG. The signal-averaging process digitizes and processes a series of QRS complexes (usually several hundred) recorded from the body surface. The result is a clean, high-fidelity average QRS complex in which small (low-amplitude) details can be seen which are not visible on a normal ECG. In many patients at risk for lethal arrhythmias, low-amplitude afterpotentials can be seen immediately following the QRS complex (Figure 7.1). In theory, these afterpotentials may represent electrical activity caused by localized slow conduction in one or more reentrant circuits. If so, the signal- averaged ECG may be a method of detecting the presence of reentrant circuits in patients who have underlying cardiac disease known to predispose to reentry. When considered with other risk factors, as listed in Table 7.2, the prognostic value of the signal-averaged ECG appears to be roughly additive. Thus, for instance, a patient with a depressed ejection fraction, a prior myocardial infarction, complex ectopy, and a positive signal-averaged study would have a 1-year risk of sudden death of 20–30%. The signal-averaged ECG should always be interpreted along with these other risk factors.
Figure 7.1 Signal-averaged ECG. The first panel shows a normal signal-averaged ECG, in which no late potentials are present. The second panel shows an abnormal signal-averaged ECG. The late potentials may indicate areas of slow conduction within the ventricular myocardium, suggesting the presence of a reentrant circuit.
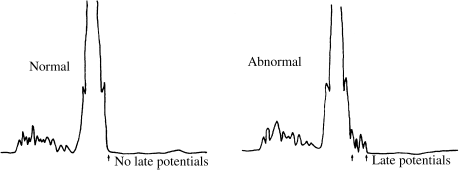
In summary, patients who have survived a myocardial infarction or who have a depressed left ventricular ejection fraction from any cause are at increased risk for sudden death from reentrant ventricular tachyarrhythmias. The risk increases when the underlying cardiac disease is accompanied by complex ventricular ectopy or a positive signal-averaged ECG. Considering only the fact that each year between 500 000 and 1 000 000 people suffer myocardial infarctions, the pool of patients at risk for sudden cardiac death is seen to be huge. It is from this very large pool of individuals that most of the 400 000 sudden-death victims each year are drawn.
Clinical Characteristics of Reentrant Ventricular Tachyarrhythmias
The reentrant ventricular tachyarrhythmias take two major forms—ventricular tachycardia and ventricular fibrillation—and result in three major symptom complexes: sudden cardiac death, syncope, or minimal symptoms such as dizziness and palpitations.
Ventricular tachycardia (Figure 7.2) is a relatively organized tachyarrhythmia with discrete QRS complexes. It can be either sustained or nonsustained and can be monomorphic or polymorphic— polymorphic ventricular tachycardias tend to be faster and less stable than monomorphic tachycardias. The rate of ventricular tachycardia can be from 100 to more than 300 beats/min. At slower rates, ventricular tachycardia often does not cause significant hemodynamic compromise and may be relatively asymptomatic. The symptoms produced by ventricular tachycardia also depend on the morphology of the tachycardia, the severity of the underlying heart disease, the vascular tone, the geometry of ventricular contraction during the tachycardia, and whether the patient is upright or supine. At faster rates (usually 220 beats/min or faster), the tachycardia is so rapid that it may be impossible to distinguish the QRS complex from the T waves. This type of ventricular tachycardia is often referred to as ventricular flutter.
Figure 7.2 Ventricular tachycardia. In unimorphic (or monomorphic) ventricular tachycardia, all QRS complexes are similar in morphology. In polymorphic ventricular tachycardia, the QRS complexes have constantly changing morphologies. In ventricular flutter, the ventricular rate is so rapid that the QRS complexes cannot be readily distinguished from T waves.
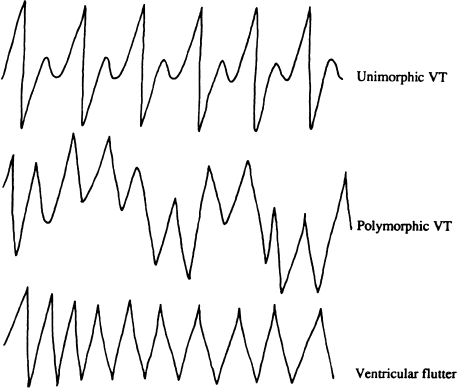
Ventricular fibrillation (Figure 7.3) is a completely disorganized tachyarrhythmia without discrete QRS complexes. This arrhythmia causes instant hemodynamic collapse and rapid loss of consciousness, because the heart immediately ceases to contract meaningfully. When ventricular fibrillation begins, it is associated with a coarse electrical pattern. As the heart becomes less viable (over a period of a few minutes), the amplitude of fibrillation waves seen on the ECG becomes finer and finer. Finally, all electrical activity ceases (flatline).
Figure 7.3 Ventricular fibrillation. Ventricular fibrillation is a completely chaotic ventricular rhythm without discernible QRS complexes.
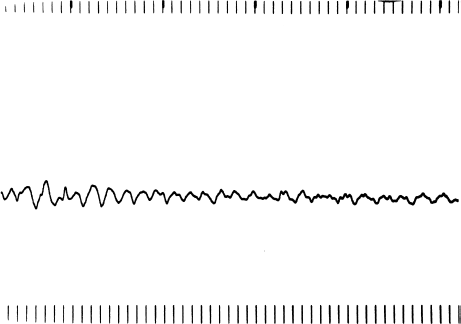
Recordings from patients who wore Holter monitors at the time of sudden cardiac death have often displayed the following progression of arrhythmias (Figure 7.4): an acute increase in ventricular ectopy is followed within a few seconds to minutes by ventricular tachycardia, which in turn degenerates (again in seconds to minutes) to coarse and then fine ventricular fibrillation. After another 5–7 minutes, there is no electrical activity. Patients who are successfully resuscitated during such an episode (electrophysiologists have called them, oxymoronically, “sudden-death survivors”) are often labeled as having had primary ventricular fibrillation, because that is the rhythm commonly seen by the time rescuers arrive. If the rescuers are a little too late, they see the flatline pattern of the dead heart. This has led to the popular misconception that sudden bradycardia is responsible for a large proportion of sudden deaths. The 1-year risk of arrhythmia recurrence for sudden death survivors is between 30 and 50%. Most recurrences are fatal.
Figure 7.4 A typical sequence of events in sudden death. This figure shows tracings from a Holter recording made in a patient who experienced sudden death. At 12:01 pm the patient is in sinus rhythm with PVCs. At 12:03 pm ventricular tachycardia occurs, which degenerates to ventricular fibrillation at 12:05 pm. By 12:08 pm, fine ventricular fibrillation is present. All electrical activity has ceased by 12:10 pm.
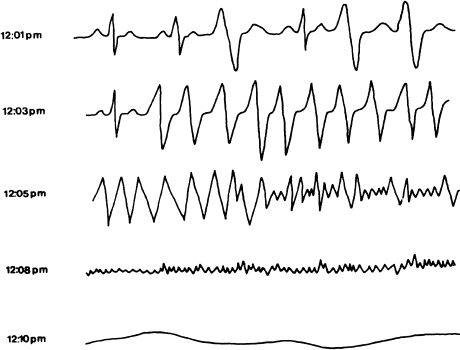
When syncope occurs in a patient who has a history of previous myocardial infarction or cardiomyopathy (especially if complex ventricular ectopy is present), a spontaneously terminating ventricular tachyarrhythmia must be high on the list of differential diagnoses. Such patients have up to a 50% chance of having inducible ventricular tachycardia during electrophysiologic testing, and those who have inducible arrhythmias subsequently have a high risk of sudden death (nearly as high as in sudden-death survivors). It is unlikely that a patient will survive multiple episodes of hemodynamically unstable (i.e. syncope-producing) ventricular arrhythmias. Indeed, patients with underlying heart disease who present with a single syncopal episode actually have a worse prognosis than patients who present with multiple syncopal episodes (in these latter patients, there is likely to be some other cause for the syncope).
Despite the fact that reentrant ventricular tachyarrhythmias are responsible for hundreds of thousands of sudden deaths each year in the United States, it is not unusual for sustained reentrant ventricular tachycardia to present with relatively minimal symptoms (minimal symptoms being palpitations, dizziness, lightheadedness, and other minor symptoms that are less severe than loss of consciousness). Sustained ventricular tachycardia that produces only minimal symptoms almost invariably presents as a monomorphic wide-complex tachycardia whose rate is less than 200 beats/min. Patients who present with these minimally symptomatic arrhythmias have a lower risk of sudden death than patients who have lost consciousness with their presenting arrhythmias—but their risk is still far higher than normal.
Owing to the lack of severe symptoms, a major problem that occurs with these patients is that physicians often mistake their relatively well-tolerated ventricular tachycardia for supraventricular tachycardia with aberrancy. At some time in their careers, all physicians memorize a list of clues that helps them to distinguish ventricular tachycardia from supraventricular tachycardia with aberrancy in patients presenting with wide-complex tachycardia. Such a list of clues is presented in Table 7.3.
Table 7.3 Clues for distinguishing ventricular tachycardia from supraventricular tachycardia with aberrancy.
Ventricular tachycardia | Supraventricular tachycardia with aberrancy | |
AV dissociation present | 50% | Never |
QRS duration | Often >0.14 seconds | Often <0.14 seconds |
Precordial concordance | Often present | Usually not present |
If RBBB configuration: | ||
lead V1 | Initial R taller (Rsr) | Second R taller (rsR) |
lead V6 | Monophasic QRS common | Triphasic QRS common |
Axis commonly <−30̊ | Axis usually >−30̊ | |
If LBBB configuration: | ||
lead V1 | Wide R wave, >0.04 seconds | Narrow R wave |
Concordance, either all QRS complexes are positive or all are negative. |
Unfortunately, within 15 minutes of taking the examination for which they memorized those clues, most physicians forget all of them. Instead, they often substitute the following simple rule (because it is easy to remember): “Ventricular tachycardia always causes loss of consciousness.” This tenet is patently false and often leads to mistaking sustained ventricular tachycardia for paroxysmal atrial tachycardia (PAT) with aberrancy. Patients thus misdiagnosed are then sent out of the emergency room without appropriate treatment. Because their reentrant ventricular tachycardia is not recognized, the opportunity to intervene to reduce their long-term risk of sudden death is missed.
Intravenous adenosine has come into common usage for termination of supraventricular tachycardias and thus is often administered to patients with wide-QRS-complex tachycardia. When adenosine is administered, useful clues can often be seen that can lead one to a diagnosis of ventricular tachycardia. When supraventricular tachycardia of any type is treated with adenosine, careful observation usually reveals some transient change in the rhythm, even if the supraventricular tachycardia is not terminated. For instance, patients with atrial flutter or atrial tachycardia most commonly will have transient second- or third-degree AV block following IV adenosine, thus revealing the underlying mechanism of the arrhythmia. In contrast (unless the patient has one of the rare forms of ventricular tachycardia that responds to verapamil and adenosine), when adenosine is administered to a patient with ventricular tachycardia, nothing happens. Thus, when a patient with wide- complex tachycardia has no response at all to adenosine, ventricular tachycardia should immediately become the leading diagnosis.
Because the clues listed in Table 7.3 are difficult to remember, the author proposes an alternative simple rule:
When patients with previous myocardial infarction or cardiomyopathy present with wide-complex tachycardia, it is ALWAYS ventricular tachycardia, regardless of symptoms.
This rule will lead to the correct diagnosis in more than 95% of patients with underlying cardiac disease who present with wide-complex tachycardia. If such patients are referred for a more aggressive evaluation, the few who do have supraventricular arrhythmias will be diagnosed during electrophysiologic testing.
The Electrophysiologic Study in the Evaluation of Reentrant Ventricular Tachyarrhythmias
The electrophysiology study has revolutionized our understanding and management of lethal ventricular arrhythmias. Through the electrophysiology study, we have come to learn that most patients with ventricular tachyarrhythmias have reentrant foci as the source of their arrhythmias, that the arrhythmias can be reproduced safely in the laboratory, that some reentrant circuits can be successfully ablated using transcatheter techniques, and that no matter how thorough and aggressive one may be in testing antiarrhythmic drugs, these drugs cannot be fully relied upon to protect against recurrent ventricular arrhythmias.
The reasons for performing electrophysiology studies in patients known or suspected to have sustained ventricular tachyarrhythmias are: (1) to diagnose the presence of a reentrant circuit that can cause a ventricular tachyarrhythmia; (2) to test the effect of antiarrhythmic drugs on the inducible arrhythmias; (3) to assess the feasibility of mapping and ablating a reentrant circuit; and (4) to optimize the programming of an antitachycardia device.
Inducing Ventricular Arrhythmias
In considering the techniques used for inducing ventricular arrhythmias, we should begin by reviewing the prerequisites for reentry. First, an appropriate anatomic circuit should be present (see Figure 2.6). Second, the electrophysiologic characteristics of the circuit should be such that an appropriately timed premature impulse can block down one pathway (pathway B) and conduct down the other (pathway A) to establish a continuously circulating impulse. Third, the initiating premature impulse must reach the circuit at a critical instant in time (i.e. when pathway B is refractory and pathway A has recovered).
The successful induction of ventricular tachycardia in the electrophysiology laboratory depends on the first two prerequisites being in place: an anatomic circuit with appropriate electrophysiologic characteristics must be present. The only prerequisite for reentry supplied by the electrophysiologist is the critically timed premature impulse. By programmed stimulation, the electrophysiologist attempts to deliver a premature impulse to the reentrant circuit at just the right moment to induce ventricular tachycardia.
Delivering a premature impulse to a reentrant circuit at just the right moment is sometimes not easy. As noted in Chapter 4, the distance between the pacing electrode and the reentrant circuit, as well as the refractory characteristics and conduction velocity (i.e. the functional refractory period (FRP)) of the intervening tissue, determine whether it is possible for a paced impulse to reach the reentrant circuit early enough to initiate an arrhythmia. Unfortunately, the precise location of the suspected reentrant circuit is almost always unknown, and the FRP of the tissue between the pacing electrode and the reentrant circuit cannot be measured. Thus, the measures used to optimize conditions to allow premature paced impulses to arrive at the reentrant circuit early enough to initiate reentry are necessarily empiric.
As we have seen, pacing at faster rates (i.e. at shorter cycle lengths) decreases the refractory periods and increases the conduction velocity in ventricular myocardium, thus decreasing the FRP. Shorter FRPs will allow premature impulses to arrive at the reentrant circuit earlier and will increase the chances of initiating reentry. Stimulation protocols use two basic techniques to minimize the FRP of the ventricular myocardium: coupling multiple premature impulses together (typically up to three programmed extrastimuli) and pacing incrementally at rapid rates.
In addition, most stimulation protocols call for pacing from more than one catheter location, on the simple premise that one location may be closer to a reentrant circuit than another. Typically, pacing is performed initially from the right ventricular apex, and if no arrhythmias are induced, the electrode catheter is moved to the right ventricular outflow tract and pacing is repeated. Some electrophysiologists will also pace from the left ventricle. The experience of most, however, is that left ventricular stimulation is unlikely to induce an arrhythmia if no arrhythmias have been inducible from two right ventricular sites.
Finally, the inducibility of a reentrant ventricular arrhythmia can occasionally be improved by infusing isoproterenol. Presumably, in some patients, catecholamines act on potentially reentrant circuits to optimize the electrophysiologic characteristics for reentry.
Programmed stimulation protocols vary somewhat from institution to institution. The basic organization of stimulation protocols is to use progressively more aggressive pacing techniques, until either the desired arrhythmia is induced or the protocol is finished (in which case the patient is declared to be noninducible).
A typical stimulation protocol is outlined in Table 7.4. The electrode catheter is initially positioned in the right ventricular apex. Following a drive train of eight incrementally paced beats (S1 beats) at a cycle length of 600 msec, a single programmed extrastimulus (S2) is introduced at a coupling interval of 500 msec (Figure 7.5a). If no arrhythmia is induced, the pacing sequence is repeated. With each pacing sequence, the coupling interval between the last S1 stimulus and the S2 stimulus is decreased by 10–20 msec, until the S2 no longer captures (i.e. the effective refractory period (ERP) for the S2 is reached). This procedure is then repeated, with S1 drive-train cycle lengths of 500 then 400 msec. If no ventricular tachycardia is induced with single extrastimuli at any of the three drive cycle lengths, the S2 stimulus is “parked” at a coupling interval approximately 20 msec longer than its ERP and a second extrastimulus (an S3) is added (Figure 7.5b). At drive cycle lengths of 600, 500, and 400 msec, the S1–S2–S3 coupling intervals are brought in as closely as possible. If double extrastimuli also fail to induce the arrhythmia, a third extra stimulus is added (S4; Figure 7.5c). At drive trains of 600, 500, and 400 msec, the S1–S2–S3–S4 intervals are brought in as tightly as possible. If no ventricular tachycardia is induced with single, double, or triple extrastimuli at any of the three drive cycle lengths, incremental pacing is performed. Incremental trains consisting of 8–12 stimuli are introduced with progressively shorter cycle lengths, beginning at a cycle length of 350 msec and decreasing to ventricular refractoriness (Figure 7.5d). If no ventricular tachycardia is induced from the right ventricular apex, the electrode catheter is repositioned to the right ventricular outflow tract, and the entire stimulation sequence is repeated. If ventricular tachycardia is not induced, an isoproterenol infusion is begun (to produce sinus tachycardia of 110–140 beats/min), and the entire stimulation sequence is repeated. If no ventricular tachycardia has been induced with any of these measures, the patient is deemed to be “noninducible.”
Figure 7.5 Typical stimulation protocol for inducing ventricular tachycardia. Right ventricular pacing is illustrated. (a) Pacing begins with the introduction of a single extrastimulus (S2) following drive trains of incrementally paced beats (S1). (b) If ventricular tachycardia is not induced, two extrastimuli are used (S3). (c) If ventricular tachycardia is not induced with two extrastimuli, three extrastimuli are used (S4). (d) If ventricular tachycardia is still not induced, incremental bursts are used.
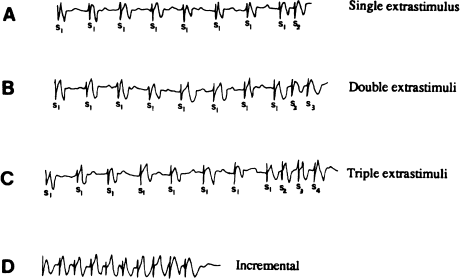
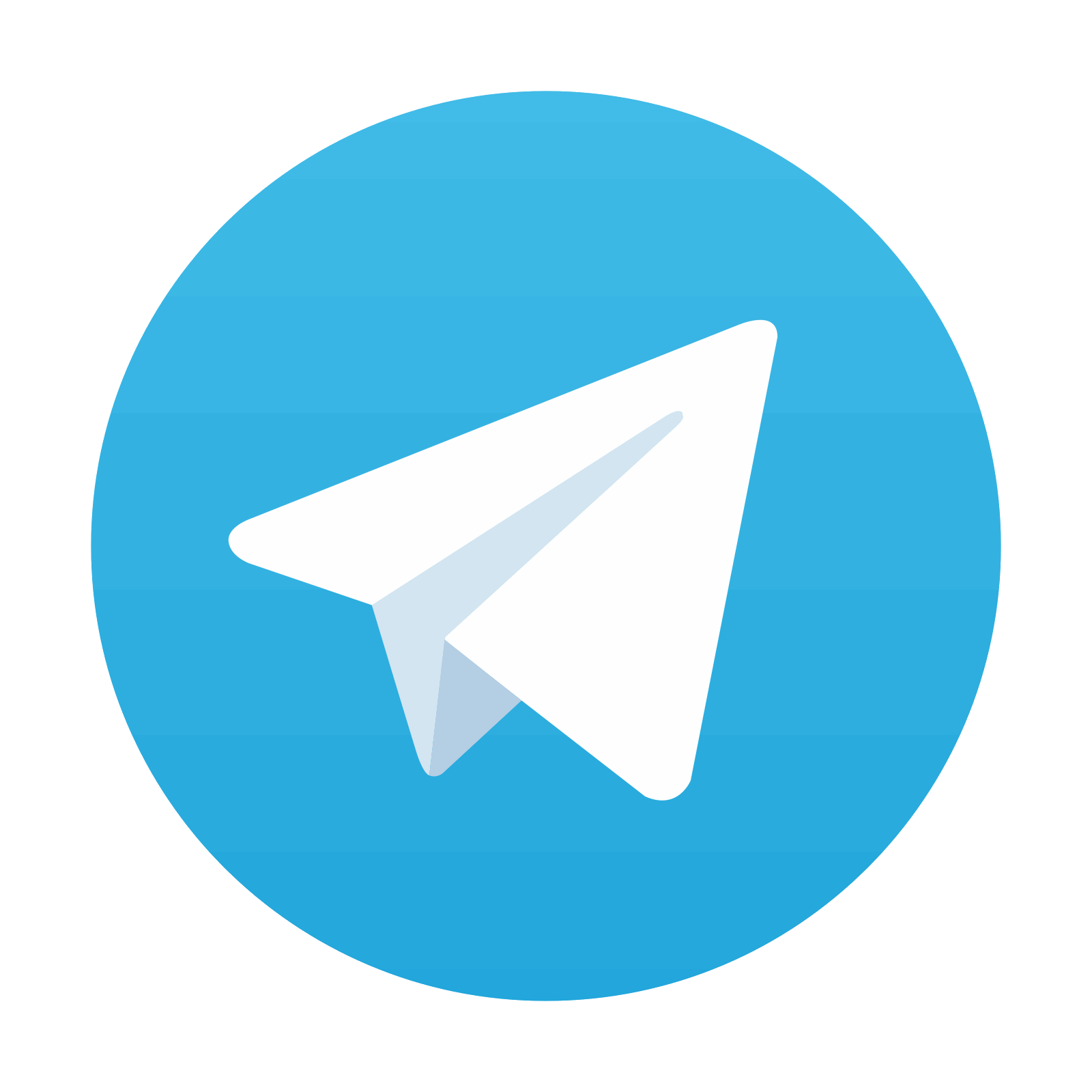
Stay updated, free articles. Join our Telegram channel

Full access? Get Clinical Tree
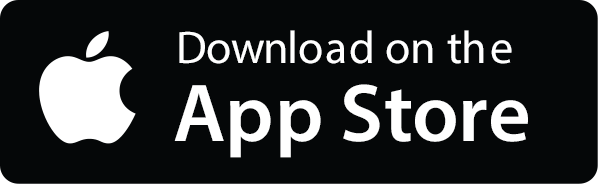
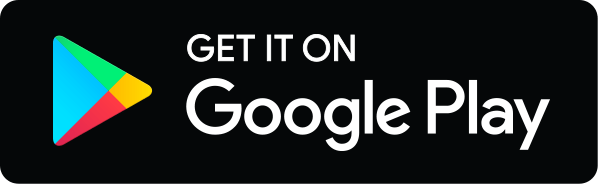