, Dilip R. Karnad2 and Snehal Kothari3
(1)
Cardiac Safety Services Quintiles, Durham, North Carolina, USA
(2)
Research Team, Cardiac Safety Services Quintiles, Mumbai, India
(3)
Cardiac Safety Services Global Head, Cardiac Safety Center of Excellence Quintiles, Mumbai, India
It is an unfortunate but immutable fact that no biologically active drug is free from the possibility of causing adverse reactions in certain individuals who are genetically and/or environmentally susceptible.
1.1 Introduction
The word “drug” has various connotations in everyday language. In this book, it refers specifically to pharmaceutical and biopharmaceutical molecules that are used to treat or prevent biological states of clinical concern. The term pharmaceutical drug typically refers to a small-molecule drug (around 20–100 atoms, with a molecular weight of less than 900 daltons), while the term biopharmaceutical drug refers to macromolecules, often a protein produced via the large-scale cultivation of microbial or mammalian cells, which are much larger. For example, the immunoglobulin G (IgG) antibody is around 25,000 atoms and has a molecular weight of approximately 150 kilodaltons (Azbio web site).
For a new drug to receive regulatory approval for marketing, regulators in respective geographical jurisdictions must decide that the drug has a favorable benefit–risk balance. This necessitates that the pharmaceutical or biopharmaceutical company requesting marketing approval for their drug must provide compelling evidence of the drug’s therapeutic benefit and compelling evidence that it is acceptably safe. The term “acceptably safe” may initially seem strange, but it is appropriate. It is an unfortunate but immutable fact that no biologically active drug is free from the possibility of causing adverse reactions in certain individuals who are genetically and/or environmentally susceptible. When making marketing approval decisions, regulators therefore balance the therapeutic benefit provided by a drug with its toxicity. This concept is encapsulated by the term benefit–risk balance. Assessments of benefit are relatively straightforward, but assessments of toxicity are far from it, as will be seen throughout this book.
1.2 Drug Safety
The term drug safety is widely used when discussing the degree and extent of a drug’s toxicity. Upon first examination, this term might also seem strange: what is actually being discussed can be better described as drug harm (Sect. 15.7 provides more detailed discussion on this topic). However, several reasons support our choice of the term drug safety. One of these is the nature of media coverage of reports of purported cardiovascular (and other) side effects of marketed drugs. The perception of a cardiovascular risk being associated with a marketed drug, whether or not the risk has been sufficiently well investigated to regard the risk as real, attracts enormous media attention. As Turner and colleagues (Turner et al. 2010) commented, “In the era of sensationalist, sound-bite coverage, clinical science sadly falls very low on the list of points to be covered in the allotted 30 s of television coverage.” When a cardiovascular safety concern associated with a marketed drug is “identified,” there is frequently a “torrent of recriminations” (Cobert 2007). In this context, the term drug harm would likely be incendiary, attracting even more emotive behavior and sensationalism to an area already fueled by far too much of both.
Such recriminations are almost always directed at the drug’s sponsor (the company that developed and marketed the drug), and the consequences can be considerable, ranging from loss of reputation to a decrease of billions of dollars in market capitalization, even if the “identification” of a risk is subsequently refuted (a Case Study of such refutation is presented in Chap. 12). However, and perhaps increasingly so, recriminations can also be targeted at regulatory agencies. In addition to being in the best interests of patients, which is always the paramount consideration, doing everything possible to identify any degree of cardiovascular risk in preapproval trials is therefore also in the best interests of sponsors and regulators. Both of these stakeholder groups are cognizant of this, and in contemporary drug development, they work very closely together in this regard.
Given that the term drug safety is the one adopted throughout this book, it needs to be defined operationally. One relatively simple but nonetheless meaningful conceptualization of safety is as the inverse of harm: the less a drug’s toxicity, the greater its safety (Durham and Turner 2008). Going a step further, a useful and widely adopted definition of drug safety in terms of benefit–risk assessment was provided by the US Food and Drug Administration’s (FDA’s) Sentinel Initiative (FDA 2008a):
Although marketed medical products are required by federal law to be safe for their intended use, safety does not mean zero risk. A safe product is one that has acceptable risks, given the magnitude of benefit expected in a specific population and within the context of alternatives available.
When making a marketing approval decision, regulators make a benefit–risk decision at the public health level. That is, they have to answer this question: On balance, is this drug likely to do more good than harm in the entire population in the region under our jurisdiction with the condition of clinical concern? The drug must be considered to have a favorable benefit–risk balance to receive marketing approval. A similar decision process occurs at the level of the individual patient once a relevant drug is approved: the physician and the patient must decide together that a particular course of pharmacotherapy has a favorable benefit–risk balance for the drug to be prescribed.
The process of benefit–risk estimation can be represented as follows (Turner 2010):
In addition to considering harm, this representation makes clear that benefit must be considered as well. Therefore, while not the primary focus of this book, examples of statistical methodologies that facilitate assessment of benefit are presented in Chap. 4.

1.3 Notable Events Driving Assessments of Drug Safety and Efficacy
At the start of the twentieth century, there were no federal regulations in the USA to protect the public from dangerous drugs. Manufacturers were not required to provide evidence of a drug’s safety, quality, or effectiveness before selling it. A series of Acts has transformed that environment into one in which compelling evidence of all three characteristics must be provided before a drug is approved for marketing. The US Pure Food and Drug Act of 1906, the first of several major consumer protection Acts, was intended to prevent the manufacture, transportation, or sale of adulterated, misbranded, or poisonous foods, drinks, and drugs. Governance of the Act was assigned to a division of the US Department of Agriculture that became the FDA in 1930.
The US Federal Food, Drug, and Cosmetics Act of 1938 (FFDCA) took consumer protection to the next level. While it had been under congressional discussion for several years, it was galvanized by the elixir sulfanilamide tragedy in which more than 100 people were fatally poisoned after ingestion of the elixir diethylene glycol, an ingredient used to dissolve sulfanilamide (see Schep et al 2009; Conrad et al. 2016). The fundamental requirement introduced by the FFDCA was provision of compelling evidence of a drug’s safety at the time of application for marketing approval.
In Europe, the thalidomide tragedy spurred events that led to the United Kingdom’s (UK’s) Medical Act of 1968. Thalidomide was first marketed in 1956 in Germany for the treatment of insomnia and vomiting in early pregnancy. In 1961, a sizeable increase in the incidence of congenital birth defects was noted. These defects were typically an absence or reduction of the long bones of the limbs in the presence of normal or rudimentary hands and feet. The association of these conditions with thalidomide was not recognized for several years after the drug was marketed, and several thousand babies in Europe were born with this congenital condition.
While public awareness of the events in Europe was a powerful motivator for congressional action for further drug law reform, as discussed in the next paragraph, the USA did not experience the same tragedy from the use of thalidomide. Having seen the reports from Europe, Dr Frances Kelsey, a newly appointed reviewer at the FDA, undertook considerable research and, as a result, took a firm stance against the drug’s approval. In recognition of her diligence, she was awarded the President’s Award for Distinguished Federal Civilian Service 2 months before further amendments to the FFDCA were signed in 1962.
Perhaps the most salient event in the regulatory process for new drug development was the passage of the Kefauver–Harris Amendments to the FFDCA, which were signed into law by President Kennedy in 1962 (see Turner 2012). Until these Amendments were signed, drug manufacturers were only required to provide compelling evidence that new products could be safely used before putting them on the market. The central novel requirements of the Amendments concerned the additional provision of compelling evidence of efficacy at the time of application for marketing approval. The Amendments were a powerful influence in the continued development of the randomized clinical trial, which is now considered to be the gold standard in providing compelling evidence of efficacy of a new drug. The more accurate term for this study design is the randomized concurrently controlled clinical trial, because a concurrent control treatment arm is a fundamental aspect of the trial’s comparative nature: however, we will use the traditional nomenclature. The randomized clinical trial, discussed in more detail in Chap. 4, became the key methodology for providing the compelling evidence of efficacy required by the Amendments.
1.4 Cardiovascular Safety
Drugs that are indicated for cardiovascular conditions are expected to influence the appropriate component(s) of the cardiovascular system to generate their desired therapeutic benefit. For example, antiarrhythmic drugs are intended to influence the aberrant pattern of the heart’s beating to return it to the normal pattern. Similarly, antihypertensive medications are expected to lower blood pressure. In contrast, drugs for noncardiovascular conditions, referred to in this book as noncardiovascular drugs, are not expected to influence the cardiovascular system. However, as noted previously, no biologically active drug is free from the possibility of causing adverse reactions. While some chapters focus on evaluations conducted during preapproval drug development to ensure to the greatest degree possible that a new drug does not carry an unacceptable risk of precipitating cardiovascular adverse reactions, discussions concerning postmarketing evaluations and discussions of the therapeutic use of marketed drugs are also provided.
Cardiovascular adverse drug reactions carry both short-term and long-term risks. For example, certain alterations in the heart’s natural pattern of electrical activity can precipitate sudden cardiac death, while increases in blood pressure can play a role in the development of various cardiovascular conditions over long periods. The domain of cardiovascular safety is therefore of central importance in contemporary drug development. Four examples of such drug safety investigations, introduced here in turn, are discussed in subsequent chapters.
1.4.1 Proarrhythmic Cardiac Safety
The first goal of the field of proarrhythmic cardiac safety (often referred to simply as cardiac safety: see Turner and Durham 2009) is to determine whether a noncardiac drug has an unacceptable propensity to lead to the polymorphic ventricular dysrhythmia called torsades de pointes (torsades) in patients who may be prescribed the drug should it subsequently be approved for marketing. Such arrhythmogenesis can result in nonfatal dysrhythmias causing syncope (fainting), but on rare occasions it can prove fatal. The second goal is to remain alert to unexpected cardiac adverse drug reactions during its therapeutic use.
Proarrhythmic cardiac safety concerns led to the marketing withdrawal of various drugs in the UK and the USA from the late 1980s to the early 2000s. These included terodiline (indicated for urinary incontinence, withdrawn from UK and US markets in 1991), antihistamine terfenadine (withdrawn from the US market in 1998), and levacetylmethadol (indicated for opiate addiction, withdrawn from the UK market in 2003). Regulatory, scientific, and clinical interest concerning these marketing withdrawals led to the release in 2005 of two guidelines from the International Conference on Harmonisation of Technical Requirements for Registration of Pharmaceuticals for Human Use (ICH): ICH S7B, which focuses on nonclinical assessments of relevance to the proarrhythmic liability of noncardiac drugs (ICH 2005a), and ICH E14, which addresses preapproval clinical assessments (ICH 2005b). ICH E14 introduced the Thorough QT/QTc (TQT) Study. An ICH E14 “Questions & Answers” document containing questions and associated answers was released in June 2008, a revised version containing additional questions and answers was released in April 2012, and a second revised version was released in March 2014. These documents provided additional guidance as experience was gained over time in the execution of the TQT study, a topic discussed in detail in Chap. 7.
A third revision, referred to in this book as ICH E14 Q&A R3, was released in December 2015 (ICH E14 Implementation Working Group 2015). ICH E14 Q&A R3 is likely to have considerable ramifications in the domain of preapproval clinical assessment of a drug’s proarrhythmic liability. It makes it clear that a second form of assessment of a drug’s proarrhythmic liability, exposure–response modeling, is an acceptable alternative to the TQT study. Chapter 8 discusses this topic. Chapter 9 then discusses the Comprehensive in vitro Proarrhythmia Assay (CiPA), an initiative investigating more sophisticated approaches to the nonclinical assessment of a drug’s proarrhythmic liability (see Sager et al 2014).
1.4.2 Cardiovascular Safety Considerations in Oncology Drug Development and Therapeutic Use
It has long been known that many oncologic agents are cardiotoxic. However, when the best hoped-for outcome was keeping patients alive for a few extra months or years, the risk of cardiovascular disease at some indeterminate point in the future did not seem problematic to many patients from a benefit–risk perspective. The excellent news is that many individuals now live cancer-free for decades following cessation of treatment, but the corollary is that we need to pay very close attention to cardiotoxicity considerations.
Several considerations are pertinent here. First, during drug development, it is necessary to adapt proarrhythmic cardiac safety investigational methodologies because the cytotoxic nature of many oncologics precludes their being administered to healthy clinical trial participants in typical Phase I trials and a TQT study. Second, given the benefit these drugs provide in life-threatening illnesses, a greater degree of risk may be acceptable when granting marketing authorization than is acceptable for drugs indicated for less severe conditions. Third, heightened clinical awareness and patient care is needed. Although such therapy has proved very successful in many cases, with disease states going into remission and patients living for many years after cessation of treatment, cardiotoxicities can manifest themselves in different time scales: some appear relatively soon, while others can appear more than a decade later. Cardiovascular events of concern include cardiomyopathy leading to heart failure, cardiac dysrhythmias, thromboembolic events, and hypertension (Turner et al 2014). This topic is discussed in Chap. 10.
1.4.3 Blood Pressure Responses to Noncardiovascular Drugs
It has become clear in recent years that noncardiovascular drugs can affect blood pressure in an off-target manner by raising or lowering pressure or by negating the beneficial hypotensive effect of concomitantly prescribed antihypertensive drugs (Sudano et al 2010; Grossman and Messerli 2012; Sager et al 2013). In most cases, the increases in blood pressure are mediated by known mechanisms, including salt and water retention, activation of the sympathetic nervous system, inhibition of prostacyclin, and inhibition of vascular endothelial growth factor. In most instances drug-induced off-target blood pressure increases are relatively small, and the clinical relevance of these small and transient effects to symptomatology, morbidity, or mortality is not known because the subject has not been systematically studied (O’Brien and Turner 2013). However, effects of more immediate concern have certainly been documented: Grossman and Messerli (2012) observed that “severe hypertension involving encephalopathy, stroke, and irreversible renal failure have been reported.” Off-target blood pressure responses have therefore garnered increasing scientific and regulatory interest in recent years. This topic is discussed in Chap. 11.
1.4.4 Cardiovascular Safety of Antidiabetic Drugs for Type 2 Diabetes
Formalization of this domain of cardiovascular safety can be traced to an FDA Guidance for Industry released in final format in December 2008 (FDA 2008b) and a subsequent Guideline released in final format by the European Medicines Agency (EMA) in May 2012 (EMA 2012). Both of these documents address the prospective exclusion of unacceptable cardiovascular risk associated with new antidiabetic drugs for the treatment of type 2 diabetes. The existence of an FDA general diabetes drug development guideline (FDA 2008c) allowed the FDA’s new document to focus exclusively on cardiovascular safety, while the EMA’s document addresses this topic as one component of an updated comprehensive guidance on multiple facets of diabetes drug development.
While the precise details of the FDA and EMA documents differ, the consequences are the same: in the vast majority of cases, a large cardiovascular safety outcome trial will need to be conducted to prospectively exclude an unacceptable cardiovascular risk, or liability, associated with a new drug. The name cardiovascular safety outcome trial conveys that the endpoints of interest are clinical outcomes as opposed to surrogate endpoints, such as blood pressure increases, which are taken to be indicative of increased cardiovascular risk but are not in themselves a clinical outcome. The outcomes most typically considered are the events comprising the major adverse cardiovascular event (MACE) composite endpoint, i.e., nonfatal myocardial infarction, nonfatal stroke, and cardiovascular death. The number of outcomes in the drug treatment group is compared with those in the control treatment group, and a statistical analysis is conducted to determine whether or not the number of outcomes in the drug treatment group is unacceptably greater than the number in the control treatment group.
Cardiovascular safety outcome trials require very large numbers of participants. As an example, the SAVOR-TIMI 53 trial for saxagliptin employed 16,500 participants (Scirica et al. 2013). Such trials can take 5–7 years to conduct and can cost several hundred millions of US dollars. We previously expressed our concern that the new regulatory landscape generated by the FDA and EMA documents may prove to be a deterrent for some sponsors considering continued involvement in developing new drugs for type 2 diabetes (Caveney and Turner 2010): such an occurrence would likely have proved detrimental to patients, who on average need a new drug added to their treatment regimen every 3–5 years to maintain adequate control of blood glucose (EMA 2012). Fortunately, despite the added burden of bringing these drugs to market, new drugs continue to be developed. For example, at the time of writing this chapter, the following drugs have been approved in the USA since issuance of the FDA’s December 2008 guidance: albiglutide, alogliptin, canagliflozin, dapagliflozin, exenatide extended-release, linagliptin, liraglutide, and saxagliptin.
In a 2014 paper published in the journal Circulation, Menon and Lincoff (2014) detailed ongoing cardiovascular outcome trials at the time of their paper’s preparation. After excluding the previously mentioned SAVOR-TIMI 53 trial that has now been completed, the total number of participants required for the remaining trials was around 122,500, a staggeringly large total. While access to the precise cost of each trial is not possible, it can safely be assumed that the total cost is several billion US dollars.
It is therefore of considerable interest to many stakeholders to determine the most expedient methodologies for satisfying the FDA and EMA requirements. An Expert Perspectives paper from the Cardiac Safety Research Consortium (CSRC: see Sect. 1.8) discussed clinical development strategies, operational issues, and statistical methodological issues in this regard (Geiger et al 2015). Going one step further, various professional organizations, including the CSRC, are exploring alternate ways of providing equally compelling evidence of the cardiovascular safety of new drugs for type 2 diabetes that may become components of regulatory landscapes in this area as they evolve in the future (Sager et al 2015). This topic is discussed in Chaps. 12 and 13.
1.5 Postmarketing Surveillance
In Part VI of the book, attention turns to postmarketing safety assessments. The importance of these assessments is captured succinctly yet powerfully by a quote from a report by the Institute of Medicine (IOM) of the National Academies entitled “The Future of Drug Safety: Promoting and Protecting the Health of the Public,” which reads as follows (IOM 2007):
The approval decision does not represent a singular moment of clarity about the risks and benefits associated with a drug – preapproval clinical trials do not obviate continuing formal evaluations after approval.
Chapter 14 therefore discusses a variety of topics, including clinical trials that are performed following a drug’s marketing approval, various postmarketing safety surveillance strategies, and cardiovascular safety in clinical practice. Chapter 15 then provides discussion of a sample of other domains of drug safety: cardiovascular safety is a very important domain, but by no means the only safety domain. Topics include adherence to prescribed medication regimens, abuse of opioid medications, and safety aspects of precision medicine.
1.6 Clinical Research Methodology
This book discusses experiments involving human participants, also known as clinical trials. It is incumbent on all individuals conducting such research to gain a solid understanding of all aspects of clinical research methodology. This term is used as an overarching term to encapsulate study design, experimental methodology, operational execution, and statistical analysis. Not everyone needs to be an expert in all of these components, but all clinical researchers should be well versed enough in areas outside their immediate expertise to appreciate all aspects of such research and, accordingly, to be able to communicate effectively with all collaborators.
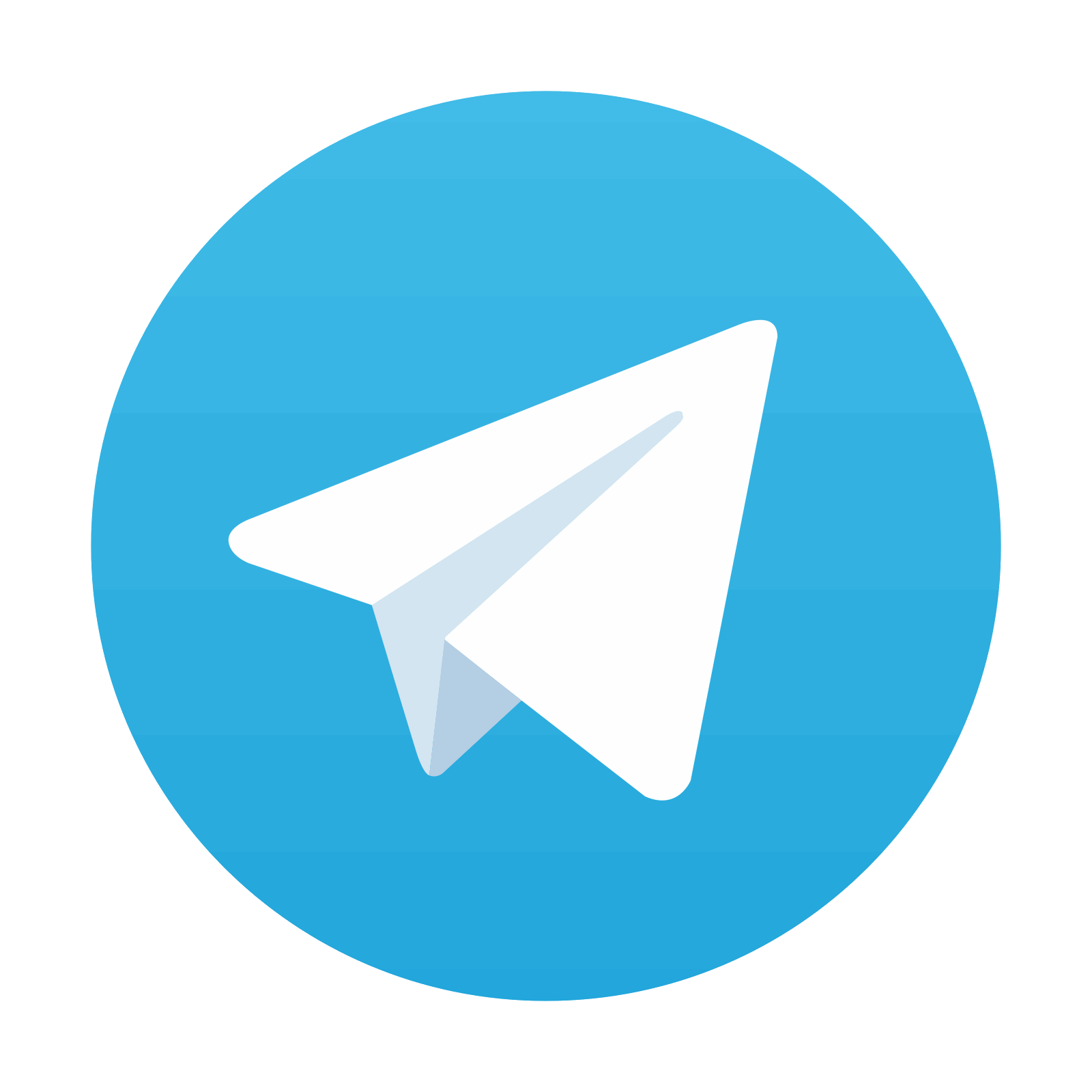
Stay updated, free articles. Join our Telegram channel

Full access? Get Clinical Tree
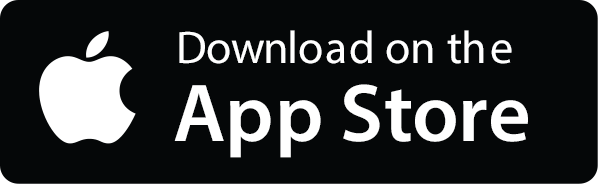
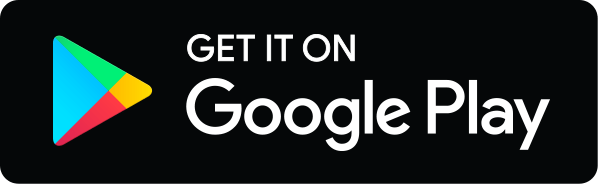