Lung cancer is a heterogeneous disease with abundant genomic alterations. Chronic dysregulated airway inflammation facilitates lung tumorigenesis. In contrast, antitumor host immune responses apply continuous selective pressure on the tumor cells during the evolutionary course of the disease. Unprecedented advances in integrative genomic, epigenomic, and cellular profiling of lung cancer and the tumor microenvironment are enhancing the understanding of pulmonary tumorigenesis. This understanding in turn has led to advancements in lung cancer prevention and early detection strategies, and the development of effective targeted therapies and immunotherapies with survival benefit in selected patients.
Key points
- •
Lung tumorigenesis is a multistage process defined by the progressive accumulation of mutations in oncogenes and tumor suppressor genes, resulting in perturbation of multiple cellular pathways.
- •
Chronic airway inflammation contributes to pathologic alteration of the lung microenvironment and promotes carcinogenesis.
- •
Host immunosurveillance imposes selective pressure on tumor cells during the evolutionary course of lung cancer.
- •
Advances in genomic profiling of lung cancer have facilitated the development of targeted therapies.
- •
Enhanced understanding of critical immunologic pathways within the tumor microenvironment have led to the discovery of immunotherapies that have revolutionized the treatment of lung cancer.
Introduction: the biology of lung cancer
Lung cancer is the leading cause of cancer-related death worldwide. Approximately 15% of lung cancers have the histologic subtype of small cell lung cancer (SCLC) and the remaining 85% are classified as non–small cell lung cancer (NSCLC). The most common subtypes of NSCLC are lung adenocarcinoma (LUAD) and lung squamous cell carcinoma (LUSC). Lung cancer, most often associated with tobacco smoking, is a heterogeneous disease with an abundance of genetic and epigenetic alterations. These genetic changes result in activation of oncogenic pathways and/or inhibition of tumor suppressor genes that are critical for malignant transformation of tumor precursor cells. However, the pathophysiology of lung cancer cannot be fully understood by enumeration of dysregulated pathways in the tumor cells but instead must encompass the contributions of the tumor microenvironment (TME) and host immune responses, which are intimately intertwined during every step of tumorigenesis. Enhanced understanding of the genomic landscape of lung cancer and the biology of the TME and host immune cells has led to the development of novel targeted therapeutics and immunotherapies that have revolutionized the treatment of lung cancer.
Genomic Landscape of Lung Cancer
The multistage process of lung tumorigenesis, from dysplasia and preneoplasia through carcinoma in situ to metastatic cancer, is defined by the progressive accumulation of mutations in oncogenes with dominant gain of function and tumor suppressor genes with recessive loss of function. Genomic alterations can originate from somatic mutations, homozygous gene deletions, gene amplifications and translocations, or epigenetic silencing. These genomic alterations, in turn, result in dysregulated cellular pathways that enable premalignant cells to become tumorigenic. Detailed genomic profiling of lung cancer has revealed significant heterogeneity among patients. Many oncogenes and tumor suppressor genes have been identified, paving the way for the development of novel targeted therapeutics ( Fig. 1 ).

The genomic landscape of lung cancer in an individual is not static but is spatially and temporally heterogeneous. During carcinogenesis, genomic instability within the bulk tumor results in divergent evolution of tumor cell clones with distinct genomic profiles and molecular signatures. This intratumoral heterogeneity has important clinical implications in cancer genome evolution and acquired resistance to therapy. A recent study using whole-exome sequencing (WES) of multiple regions of individual tumors from patients with NSCLC revealed significant intratumoral heterogeneity in somatic mutations and copy-number alterations, which was associated with an increased risk of recurrence and death.
Genetic and molecular alterations of non–small cell lung cancer
Large-scale sequencing studies have revealed distinct genomic differences between LUAD and LUSC. The most commonly mutated oncogenes in LUAD are kristen rat sarcoma ( KRAS ) and epidermal growth factor receptor ( EGFR ), which are implicated in the initiation of tumorigenesis. Although KRAS and EGFR mutations are usually mutually exclusive, when they coexist, the KRAS mutation drives resistance to EGFR inhibitors. Other targetable oncogenes in LUAD include human epidermal growth factor receptor 2 ( HER2 ), MET proto-oncogene ( MET ), and ret proto-oncogene ( RET ). Fusion oncogenes involving anaplastic lymphoma kinase ( ALK ), and reactive oxygen species proto-oncogene 1 ( ROS1 ) are also observed in LUAD. In contrast, actionable driver mutations are not prevalent in LUSC.
The most commonly mutated tumor suppressor genes in LUAD include tumor protein p53 ( TP53 ), kelch-like ECH-associated protein 1 ( KEAP1 ), liver kinase B1 ( LKB1 ), and neurofibromin 1 ( NF1 ). TP53 mutation is also present in more than 90% of LUSC. TP53, which is an integral sensor of genomic damage and suppressor of proliferation, is implicated in tumor progression. Cyclin-dependent kinase inhibitor 2a (CDKN2A), a tumor suppressor that regulates the cell cycle, is also inactivated in 70% of LUSC.
Although smoking is implicated in the pathogenesis of NSCLC, approximately 10% of cases occur in never-smokers. Studies reveal that the genomic landscape in NSCLC is distinct between smokers and nonsmokers. NSCLCs from smokers have among the highest tumor mutational burdens (TMBs) of all malignancies, and frequently contain the tobacco exposure signature of cytosine to adenine (C>A) transversions. , , , In contrast, NSCLCs in never-smokers generally have a 10-fold lower number of point mutations compared with smokers, and predominantly contain transition of cytosine to thymine (C>T). Furthermore, the prevalence of oncogene-addicted NSCLC with EGFR mutations and/or ALK and ROS1 translocations is higher in never-smokers, whereas KRAS and TP53 mutations are more commonly found in smokers.
Genetic and molecular alterations of small cell lung cancer
SCLC is a high-grade neuroendocrine (NE) tumor. Approximately 75% of SCLCs express the transcription factor achaete-scute complex–like 1 (ASCL1) and 15% express neurogenic differentiation factor 1 (NEUROD1), which are master regulators of neuronal and NE differentiation. However, approximately 15% of SCLC tumors lack these transcription factors and do not express the NE program. Although SCLC has been regarded as a homogeneous disease, recent studies reveal considerable intertumoral heterogeneity in the genomic landscape of SCLC. ,
The dominant genetic alteration in more than 90% of SCLCs is the loss of function of TP53 and retinoblastoma protein ( RB1 ), which are essential for tumor initiation. These two canonical tumor suppressors are critical sensors that integrate intracellular and extracellular signals to regulate cell proliferation and apoptosis. The MYC family of oncogenes are also frequently overexpressed or amplified in SCLC, and are associated with genomic instability and shortened survival. , Notch signaling, which can have oncogenic and tumor-suppressive effects, is also altered in SCLC. As a tumor suppressor in SCLC, Notch pathway negatively regulates NE differentiation. This pathway is inactivated in most SCLCs by either inactivating mutations or overexpression of nonfunctional Notch ligand delta–like protein 3 (DLL3).
Similar to NSCLC in smokers, SCLCs possess high TMB with the tobacco exposure signature of C>A transversions. , , In contrast with NSCLC, mutations in kinase genes in SCLCs are modest, and mutations in EGFR or KRAS are extremely rare. There is currently no US Food and Drug Administration (FDA)–approved targeted therapy for SCLC.
The Tumor Microenvironment
The TME contains extracellular matrix, vascular and lymphatic networks, cancer-associated fibroblasts, and infiltrating host immune cells, which are intimately intertwined with tumorigenesis. Studies reveal that intrinsic genomic alterations within premalignant or neoplastic cells can reprogram the composition of the TME to facilitate carcinogenesis. In contrast, chronic inflammation caused by extrinsic factors, such as unresolved infections or exposure to carcinogens, can orchestrate the lung TME to promote cancer progression.
Host immune cells are a critical component of the TME, and studies reveal that immune cells are present within the TME of nearly all premalignant and neoplastic lung lesions. The composition and function of these infiltrating immune cells have been a subject of intense investigation in the past decade. It is now understood that host immune cells play a dichotomous role throughout the evolution of lung cancer, and can either facilitate the eradication of malignancy or promote an immunosuppressive TME favoring tumor progression.
The immune tumor microenvironment: tumor-promoting chronic inflammation
Evidence suggests that chronic airway inflammation contributes to pathologic alterations of the lung microenvironment to facilitate cancer initiation and progression. Epidemiologic studies reveal that patients with chronic microbial colonization have an increased risk of lung cancer. Patients with chronic obstructive pulmonary disease, which is a chronic inflammatory disorder, also possess increased risk of lung cancer even after correction for the risk associated with cigarette exposure.
Chronic inflammation may contribute to tumorigenesis via several mechanisms. Inflammation-induced leukocyte infiltration into the lung can induce DNA damage through the generation of reactive oxygen species (ROS). Dysregulated chronic inflammation can also foster aberrant expression of growth factors and cytokines, such as transforming growth factor beta (TGF-β), interleukin (IL)-1β, IL-4, IL-6, IL-8, IL-10, and IL-22, which activate multiple tumorigenic pathways, such as nuclear factor kappa B subunit (NF-κB) and cyclooxygenase 2 (COX-2) to promote tumorigenesis.
The transcription factor NF-κB operates as a lynchpin by exerting oncogenic effects within the tumor cells through suppression of apoptosis and stimulation of proliferation, and concomitantly activating protumorigenic inflammatory genes in the TME. In addition, NF-κB regulates the expression of various inducible protumorigenic enzymes, such as matrix metalloproteinases (MMPs), inducible nitric oxide synthase (iNOS), and COX-2, which generate mutagenic molecules and growth factors that promote lung cancer progression.
COX-2 has been implicated in the pathogenesis of inflammation-associated lung cancer, and its increased expression is associated with poor outcomes. COX-2 is the rate-limiting enzyme in the conversion of arachidonic acid into prostaglandin H2 (PGH2), which is a substrate for prostaglandin and thromboxane synthases. Induction of COX-2 activity by inflammatory mediators, such as TGF-β and IL-1β, or by cigarette smoke results in increased production of prostaglandin E2 (PGE2). PGE2 is a potent growth-promoting and inflammatory metabolite that activates multiple downstream signaling pathways associated with proliferation, epithelial-to-mesenchymal transition, and apoptosis resistance.
The immune tumor microenvironment: antitumor immunity
Immune surveillance
Immunosurveillance by host immune effectors imposes continuous selective pressure on tumor cells throughout the evolution of lung cancer. Although both innate and adaptive immune responses are involved in antitumor immunity, host T cell recognition of tumor antigens represents the central tenet of immunosurveillance and immunoediting.
Specificity is the hallmark of adaptive T-cell immunity against tumor. Genomic mutations in lung cancer can give rise to mutant proteins that, when processed, result in the generation of neoantigens. Appropriate presentation of tumor antigens by professional antigen-presenting cells (APCs), namely dendritic cells (DCs), within the major histocompatibility complex (MHC) molecules (signal 1), along with positive costimulatory signal cluster of differentiation 80 (CD80) or CD86 (signal 2) and proinflammatory cytokines (signal 3) primes naive host T cells against specific tumor antigens. Following priming and activation, effector T cells no longer require costimulatory signals and can eliminate tumor cells after recognition of MHC-bound cancer antigens. Indeed, a recent study in patients with early-stage NSCLC identified CD8 + tumor-infiltrating lymphocytes (TILs) that are reactive to tumor clonal neoantigens. Furthermore, the presence of mature DCs, tertiary lymphoid structures, and cytolytic CD8 + T cells within the TME has been implicated as a positive prognostic indicator in patients with lung cancer.
Immune escape
Most lung cancers escape host immune surveillance through a variety of mechanisms. Tumors can directly suppress host immune responses by activating negative regulatory pathways known as immune checkpoints. Two checkpoints, cytotoxic T-lymphocyte protein 4 (CTLA-4) and programmed cell death-1 (PD-1) have generated much attention recently. The coinhibitory receptor CTLA-4 is upregulated on T cells following activation and initiates negative regulation by competing with the CD28 receptor for binding of costimulatory CD80 and CD86 molecules expressed by APCs ( Fig. 2 A). In contrast, PD-1 is primarily expressed by exhausted T effector cells during long-term antigen exposure, such as chronic viral infection or malignancy. Adaptive expression of programmed cell death ligand-1 (PD-L1) within the TME by tumor cells or the myeloid compartment, in response to inflammatory signals such as interferon (IFN)-γ, can significantly attenuate the antitumor activity of PD-1–expressing TILs that recognize tumor-specific antigens ( Fig. 2 B). Immune checkpoint blockade with antibodies targeting PD-1 or PD-L1 results in preferential activation of exhausted tumor-specific T cells and is generally associated with lower toxicity profile compared with CTLA-4 inhibitors.

Lung tumors also restrict host immunosurveillance through suppression of functional antigen presentation. Loss of heterozygosity in human leukocyte antigen (HLA) was found to occur in 40% of patients with NSCLC, which was associated with a high burden of subclonal neoantigens. Genomic variation within MHC class I has also been implicated in hindering host immune responses against certain epitopes. Recently, a study revealed that tumors evade immune recognition by epigenetic repression of neoantigen transcription through promoter hypermethylation.
Lung cancers can also alter the composition of the TME to establish an immunosuppressive milieu characterized by an abundance of inhibitory molecules, such as TGF-β, IL-6, PGE2, and vascular endothelial growth factor (VEGF), and an accumulation of immunosuppressive cells, such as regulatory T cell (T reg ) and myeloid-derived suppressor cells (MDSCs) ( Fig. 2 C). T reg and MDSCs limit tumor-specific T cell responses via multiple mechanisms. Tumor-infiltrating T reg in NSCLC correlates with COX-2 expression within the TME and portends a poor prognosis. MDSC infiltration into the TME is also associated with negative clinical outcomes.
Development of more effective methods for prevention, diagnosis, and treatment
Targeting Inflammation for Lung Cancer Prevention
Although the prevalence of smoking has decreased, it remains the most important risk factor for the development of lung cancer. Lung cancer prevention strategies have largely focused on tobacco cessation and are reviewed elsewhere in this issue. Significant efforts are also directed at developing chemoprevention strategies, which encompass the use of agents to prevent, delay, or reverse carcinogenic progression in high-risk populations.
Given the strong association between chronic inflammation and carcinogenesis, studies have evaluated the role of antiinflammatory drugs in cancer prevention. Evidence suggests that long-term use of aspirin is associated with reduction in cancer death. However, the chemopreventive efficacy of aspirin to mitigate lung cancer risk is equivocal according to recent meta-analyses. , Recently, a nationwide retrospective study evaluating 13 million patients revealed that low-dose aspirin use for more than 5 years was associated with risk reduction of lung cancer. Although the overall effect is modest, a subgroup analysis revealed enhanced efficacy in patients older than 65 years. Future studies are needed to identify patient populations that would derive the greatest benefit. An ongoing phase II trial is evaluating chemoprevention with aspirin compared with placebo in high-risk patients with subsolid lung nodules.
Selective inhibition of COX-2 with celecoxib has also been evaluated for lung cancer prevention. An early-phase study in active smokers revealed that treatment with celecoxib for 6 months significantly reduced the expression of the proliferation maker Ki-67 in serial bronchial biopsies and inhibited secretion of IL-10 and PGE2 in the lung microenvironment. In 2 subsequent randomized trials, treatment of current and former smokers with celecoxib also resulted in significant reduction of Ki-67 in post-treatment biopsies compared with placebo. , Similarly, a chemoprevention trial in lung cancer evaluating the effect of inhaled iloprost, an analogue of prostaglandin I2 (PGI2), revealed significant improvement of endobronchial histology in former smokers, but not current smokers, which highlights the importance of smoking cessation in lung cancer prevention.
Recently, IL-1β, a critical mediator of inflammation-driven carcinogenesis, has emerged as a potential target for lung cancer prevention. In the CANTOS trial, canakinumab, a monoclonal antibody targeting IL-1β, was associated with a significantly lower rate of recurrent cardiovascular events than placebo in patients with prior myocardial infarction. Remarkably Canakinumab resulted in significant dose-dependent reduction of lung cancer incidence and mortality compared with placebo. Further analysis revealed that patients with the greatest reductions in biomarkers of inflammation, namely high-sensitivity C-reactive protein and IL-6, benefited the most from canakinumab, highlighting the antiinflammatory role of canakinumab in lung cancer prevention. Ongoing trials are evaluating canakinumab as monotherapy or in combination with checkpoint inhibitors as adjuvant or neoadjuvant therapy in early-stage NSCLC.
Strategies designed to enhance host immune responses against premalignant lesions are also being used for lung cancer immunoprevention. A phase I study is evaluating the potential of vaccination with Mucin 1, a common cancer-associated antigen, in cancer prevention in current and former smokers with high risk of lung cancer. An ongoing phase II study is evaluating the checkpoint inhibitor, pembrolizumab, a PD-1 antibody, in patients with high-risk pulmonary nodules.
Early Detection of Lung Cancer
Most lung cancers are diagnosed at an advanced stage, when the prognosis remains poor. In the National Lung Screening Trial (NSLT), annual low-dose computed tomography (LDCT) screening in high-risk populations resulted in 20% relative reduction in lung cancer–related mortality and 6.7% decrease in all-cause mortality. Lung cancer risk prediction scores, such as PLCO m2012 , that better define individuals at risk of lung cancer and improve the receiver operating characteristics of this screening tool have been developed, and are discussed elsewhere in this issue.
Although LDCT screening has led to progress in early lung cancer detection, improvement in patient outcomes has been incremental. Recently, a transformative cancer interception strategy has been proposed that aims to shift the paradigm of diagnosis and treatment to the earliest phase of the disease in order to block the progression of premalignancy to invasive cancer. These efforts have been facilitated by the PreCancer Atlas (PCA) initiative, introduced by the National Cancer Institute (NCI), which uses a comprehensive multiomic strategy to establish detailed molecular and cellular characteristics of premalignant lesions and their evolution to invasive cancer. Technological advances such as autofluorescence bronchoscopy (AFB), which allows serial sampling of preinvasive lesions within the proximal airways, as well as laser capture microscopy and multiplex immunofluorescence (MIF), which permit in-depth genomic interrogation and immunophenotyping of paraffin-imbedded tissue, have expedited these efforts in early detection and characterization of pulmonary premalignancy. Recent advances in the development of pulmonary PCA are highlighted below.
Studies in lung cancer have highlighted a field cancerization phenomenon, in which histologically normal-appearing tissues adjacent to lung cancer share common genomic abnormalities with the tumor. These molecular changes likely represent early alterations in normal tissue, which undergo positive selection and clonal expansion during progression to NSCLC. Consistently, a study utilizing targeted deep DNA and RNA sequencing of LUAD and tumor-adjacent atypical adenomatous hyperplasia (AAH) lesions, which are known precursors of LUAD, revealed presence of common driver mutations, such as BRAF and KRAS , in AAH lesions.
A clearer picture of the immune contexture associated with pulmonary premalignancy has also emerged recently. A study evaluated paraffin-imbedded lung cancer specimens at various stages, including premalignant AAH, adenocarcinomas in situ, and invasive LUAD, by WES and MIF, and identified somatic mutations in both premalignancy and the associated tumor as progression-associated mutations. Predicted neoantigens from these shared mutations were highly correlated with T cell infiltration and PD-L1 upregulation in premalignant lesions, suggesting an adaptive immune response to these neoantigens. These results provide evidence for mutational heterogeneity and immune recognition in pulmonary premalignancy.
Researchers are beginning to define the genomic evolution of premalignancy throughout the course of tumorigenesis. Although histologically identical, half of lung carcinoma in situ (CIS) lesions, which are precursors of LUSC, progress to invasive cancer, whereas the other half regress or remain static. Recently, an elegant study used multiple approaches to decipher the genomic, transcriptomic, and epigenomic landscape of CIS from serial samples obtained with the guidance of AFB in a longitudinally monitored patient cohort, and identified predictive models of progressive lesions with remarkable accuracy. Moreover, characteristic mutations, copy-number changes, and epigenomic modifications associated with lung cancer were observed in premalignant lesions, offering a window into early carcinogenesis.
A similar study using RNA sequencing to determine the transcriptome profile of serial endobronchial biopsies from high-risk smokers identified 4 molecular subtypes associated with clinical phenotypes in bronchial premalignant lesions (PMLs), which are precursors of LUSC. A proliferative gene signature was identified with specificity for clinical progression of PMLs. Notably, this proliferative gene signature was present even in uninvolved large airway brushings of subjects with progressive PMLs. Furthermore, the investigators observed decreased gene expression in IFN signaling, antigen processing and presentation, and T cell–mediated immunity among progressive and persistent proliferative lesions compared with regressive lesions, and these pathways correlated with decreases in immune cell types.
These studies have significantly enhanced our understanding of the early molecular, cellular, and immunologic properties that fuel the progression of PMLs to invasive cancer. Further studies are ongoing to improve early detection and risk stratification strategies, and develop personalized preventive therapies.
Lung Cancer Treatment
Identification of driver mutations in lung cancer, along with characterization of critical molecular, cellular, and immunologic pathways within the TME, has led to the discovery of multiple targeted therapeutics and immunotherapies that have revolutionized lung cancer treatment. The seminal trials that led to the approval of these drugs, and ongoing efforts to develop novel therapies, are discussed next.
Targeted therapy
Non–small cell lung cancer
Screening for driver mutations is a critical part of diagnostic work-up of lung cancer. In a recent French study, oncogenic drivers were observed in 50% of patients with NSCLC, and targeted therapy was associated with improved overall survival (OS). Similarly, the multicenter Lung Cancer Mutation Consortium in the United States identified targetable driver mutations in 64% of patients with LUAD. The patients with an oncogenic driver who received genotype-directed therapy had improved survival compared with those without targeted therapy.
Multiple tyrosine kinase inhibitors (TKIs) are FDA approved for NSCLC treatment, and these have been reviewed extensively elsewhere. EGFR and ALK alterations are the most prevalent targetable mutations in NSCLC and occur more frequently in nonsmokers and women. Osimertinib is a third-generation EGFR TKI with activity against the T780M mutation that is the most common cause of acquired resistance to the first-generation TKIs. In a randomized phase III trial comparing first-line osimertinib with either erlotinib or gefitinib in patients with EGFR -mutated advanced-stage NSCLC, osimertinib was associated with significantly improved progression-free survival (PFS), establishing osimertinib as the standard of care. The second-generation ALK inhibitor, alectinib, is currently the first-line TKI for patients with ALK -positive NSCLC because of its enhanced efficacy compared with first-generation inhibitors in 2 randomized trials. ,
ROS1 gene rearrangements are observed in approximately 2% of LUADs. Because of a high degree of homology between the tyrosine kinase domains of ROS1 and ALK, many TKIs approved for ALK -positive NSCLC have shown activity in ROS1 -positive tumors. Crizotinib is currently the standard of care for the treatment of ROS1 -positive NSCLC. Mutations in v-raf murine sarcoma viral oncogene homolog B1 ( BRAF ) gene are observed in approximately 7% of LUADs and 4% of LUSCs. BRAF belongs to the mitogen-activated protein kinase (MAPK) pathway, which includes RAS-RAF-MEK-ERK. Although NSCLC patients with BRAF -V600E mutation respond to single-agent BRAF TKIs, combination therapy with BRAF and MEK inhibitors is preferred because of the enhanced efficacy.
Significant efforts have been devoted to extending the scope of targeted therapies in NSCLC. Alterations in MET signaling are present in 7% of LUADs and are associated with acquired resistance to EGFR TKI. Several ongoing trials are evaluating the efficacy of selective inhibitors as single agents in advanced NSCLC with MET mutation, and in combination with EGFR TKIs in EGFR TKI-resistant NSCLC with acquired MET amplification. Targeted therapy with anti–c-MET antibody-drug conjugate, telisotuzumab vedotin, has also shown promising results in early-phase clinical trials for patients with MET -amplified NSCLC.
Other potentially targetable alterations in NSCLC include rearrangement of the proto-oncogene RET , which is present in 1% to 2% of patients. Treatment of patients with RET -rearranged lung cancer with multikinase inhibitors has shown limited efficacy. Ongoing early clinical trials are evaluating the efficacy of highly selective inhibitors of RET, BLU-667, and LOXO-299 in RET -altered NSCLC. Mutations in HER2 , which is an EGFR family receptor tyrosine kinase (RTK), are detected in 3% of LUADs. However, targeting HER2 in lung cancer has resulted in modest efficacy. ,
Small cell lung cancer
There is currently no FDA-approved targeted therapy for SCLC. In 2012, the US Congress recognized SCLC as a recalcitrant cancer based on its 5-year survival rate of less than 7%. In the past several years, renewed effort has led to the identification of multiple therapeutic targets.
Genomic abnormalities in the MYC family of transcription factors are observed in 20% of SCLCs. In a phase II clinical trial in patients with progressive or recurrent SCLC, alisertib, a selective inhibitor of aurora kinase A that is regulated by MYC, showed a 21% objective response rate (ORR), although the genomic status of MYC was unknown in these patients.
Notch ligand DLL3 has been a target for the development of novel strategies in SCLC with promising initial results. However, a phase III study of rovalpituzumab tesirine (ROVA-T), a DLL3-targeting antibody-drug conjugate, as a second-line treatment of patients with advanced SCLC was recently placed on hold because of shortened OS. An ongoing phase III trial is evaluating ROVA-T as a maintenance therapy following frontline treatment in patients with extensive-stage SCLC (ES-SCLC).
Immunotherapy
Advances in immunotherapy have changed the treatment landscape of lung cancer. To date, 4 immune checkpoint inhibitors (ICIs) have FDA approval for NSCLC treatment: nivolumab and pembrolizumab targeting PD-1, as well as atezolizumab and durvalumab targeting PD-L1. Four immunohistochemistry (IHC)-based diagnostic assays measuring PD-L1 expression within the TME have also been approved by the FDA to aid with patient selection. Recently, atezolizumab, nivolumab, and pembrolizumab obtained FDA approval, and durvalumab was granted orphan drug designation (ODD) for the treatment of ES-SCLC.
Non-small cell lung cancer
Immune checkpoint inhibitors for metastatic non–small cell lung cancer
Three ICIs, namely nivolumab, pembrolizumab, and atezolizumab, are approved as single agents for the treatment of advanced NSCLC with disease progression on chemotherapy. Pembrolizumab is the only ICI with FDA approval as single-agent first-line treatment of advanced NSCLC. This approval is based on the results of the KEYNOTE-024 trial, which revealed superior OS with pembrolizumab compared with platinum-based chemotherapy in patients with NSCLC with PD-L1 expression greater than or equal to 50% and without EGFR/ALK alterations. Prolonged follow-up data showed persistent median OS (30.0 vs 14.2 months) benefit with pembrolizumab compared with chemotherapy.
Several trials have evaluated combinations of ICIs with platinum chemotherapy as first-line regimens in NSCLC irrespective of PD-L1 expression. In the phase III KEYNOTE-189 study, first-line pembrolizumab plus pemetrexed and platinum in patients with nonsquamous metastatic NSCLC showed significant improvement in 12-month OS (69.2% vs 49.4%), with similar toxicity profile, compared with placebo plus chemotherapy irrespective of PD-L1 tumor expression. Similarly, in the phase III KEYNOTE-407, first-line pembrolizumab in combination with carboplatin and taxane in patients with metastatic LUSC irrespective of PD-L1 tumor expression resulted in improved median OS (15.9 vs 11.3 months) compared with placebo plus chemotherapy. As a result, pembrolizumab in combination with platinum chemotherapy is now an FDA-approved first-line treatment regimen for all patients with advanced NSCLC without EGFR/ALK alterations, irrespective of PD-L1 tumor expression.
In the phase III Impower 150 trial, atezolizumab and anti-VEGF antibody bevacizumab combined with carboplatin and paclitaxel in patients with metastatic nonsquamous NSCLC without EGFR/ALK alterations improved median OS (19.2 vs 14.7 months) compared with bevacizumab, carboplatin, and paclitaxel combination, regardless of PD-L1 expression. These data led to the FDA approval of atezolizumab, bevacizumab, carboplatin, and paclitaxel as first-line treatment of this patient population. An ongoing trial is evaluating this first-line regimen in metastatic LUSC.
Immune checkpoint inhibitors for locally advanced and early-stage non–small cell lung cancer
Until recently, the standard of care for unresectable stage III NSCLC consisted of concurrent chemoradiation, which was associated with poor long-term outcome, with 5-year survival of approximately 15%. In the PACIFIC trial, which compared durvalumab (anti–PD-L1) with placebo in patients with stage III NSCLC who had completed definitive chemoradiation, durvalumab significantly improved PFS, establishing durvalumab as the standard of care in this setting. Follow-up survival results revealed that durvalumab significantly improved 24-month OS (66.3% vs 55.6%) compared with placebo. Ongoing phase III trials are evaluating the efficacy of PD-1/PD-L1 blockade following surgical resection and adjuvant chemotherapy in patients with locally advanced NSCLC.
Neoadjuvant trials of ICIs in early-stage (stage I–IIIA) NSCLC also hold promise. Although surgical resection with curative intent is the standard of care for early-stage NSCLC, relapse rates are high despite adjuvant and neoadjuvant platinum-based chemotherapy for selected patients. In a pilot study, neoadjuvant nivolumab in patients with surgically resectable early NSCLC induced a major pathologic response in 45% of resected tumors. Importantly, there were no treatment-related delays to definitive surgery. Several ongoing trials are evaluating the combination of neoadjuvant chemotherapy or radiation therapy with PD-1/PD-L1 blockade in early-stage NSCLC with promising initial and interim results.
Primary and acquired resistance to immune checkpoint inhibitors
Despite advances in cancer immunotherapy, many patients with NSCLC do not respond to ICIs (primary resistance), and others develop resistance after an initial response (acquired resistance). Significant effort has been devoted to identifying the biomarkers of response and the underlying molecular mechanisms of resistance.
PD-L1 expression by IHC is the only FDA-approved biomarker for patient selection. However, predictive potential of this test is limited, because many patients with PD-L1 expression do not respond to ICIs, whereas others with low PD-L1 levels benefit from ICIs. Increased tumor T cell infiltration before treatment is also associated with favorable responses to ICIs, consistent with the proposed mechanism that the PD-1/PD-L1 checkpoint primarily constrains the effector function of the exhausted tumor-specific TILs within the TME. Increased TMB has also been implicated in improved likelihood of response to ICIs, which supports the hypothesis that tumors with high somatic mutations likely possess neoepitopes that can be recognized by TILs. , Recent studies have identified tumor clonal neoantigen burden as a factor associated with improved responses to ICIs. , In contrast, LKB1 inactivating mutations have been implicated in driving primary resistance to anti–PD-1 therapy in KRAS -mutant LUADs.
Although mechanisms of acquired resistance in NSCLC remain obscure, several immune escape mechanisms discussed earlier, such as defective antigen presentation, immunosuppressive TME, and upregulation of other checkpoint inhibitors, may be involved. Mutations in the IFN pathways, such as JAK1/2 , have also been implicated in acquired resistance to ICIs in melanoma. Efforts are ongoing to develop synergistic combination therapies to enhance the efficacy of ICIs in patients with unfavorable genomic and immune profiles that derive primary or acquired resistance to immunotherapy.
Overcoming resistance to immune checkpoint inhibitors
One approach to overcome resistance is to enhance immune responses by combining ICIs that target nonredundant pathways of T cell inhibition. Preliminary results from the phase III CheckMate 227 trial in treatment-naive patients with stage IV or recurrent NSCLC reveals that combination of nivolumab and anti–CTLA-4 antibody ipilimumab is associated with higher PFS compared with chemotherapy in patients with high TMB, regardless of PD-L1 expression. Other synergistic checkpoints, such as LAG-3, B7-H4, and B7-H3, are also currently being evaluated in combination with ICIs targeting the PD-1 axis.
Another promising strategy involves combining ICIs with tumor vaccines, which can potentially restore tumor antigen presentation and enhance tumor-specific T cell responses. In a phase I trial in previously treated patients with advanced NSCLC, intratumoral injection of autologous DCs expressing chemoattractant CCL21 (CCL21-DC) showed local and systemic immune responses, including enhanced CD8 + T cell infiltration and PD-L1 upregulation in the TME, as well as systemic recognition of autologous tumor antigens. Expanding on these results, an ongoing trial is evaluating the combination therapy for intratumoral CCL21-DC plus pembrolizumab in advanced NSCLC. Other early-phase studies are evaluating the use of personalized neoantigen cancer vaccines in combination with ICIs.
Radiotherapy is also being evaluated in combination with ICIs to enhance immune responses. A phase II trial, PEMBRO-RT, recently evaluated whether stereotactic body radiotherapy to a single tumor site preceding pembrolizumab could enhance responses to ICI in patients with metastatic NSCLC. A doubling of ORR in the pembrolizumab plus radiotherapy group was observed compared with pembrolizumab alone. The largest clinical benefit was observed in patients with no baseline PD-L1 expression, suggesting that radiotherapy may enhance immune responses in immunosuppressed tumors.
Combination of ICIs with epigenetic modulators such as inhibitors of histone deacetylase and DNA methyltransferases (DNMTs), which may increase the tumor immunogenicity, are also being evaluated in early-phase trials.
Small cell lung cancer
Multiple ICIs have been evaluated in SCLC with promising results over the past year. Based on the results of early-phase studies, nivolumab and pembrolizumab have recently been granted accelerated FDA approval for the treatment of metastatic SCLC with disease progression after platinum-based chemotherapy and at least 1 other line of therapy.
ICIs have also been evaluated as frontline therapy for SCLC. In the phase III Impower133 trial, frontline regimen with atezolizumab in combination with carboplatin and etoposide in patients with ES-SCLC improved median OS (12.3 vs 10.3 months) compared with chemotherapy plus placebo, leading to FDA approval of this frontline regimen in ES-SCLC. Similarly, according to the results of a planned interim analysis of the phase III CASPIAN trial, frontline therapy with durvalumab in combination with etoposide and platinum-based chemotherapy in patients with ES-SCLC led to significant improvement in OS and PFS. Based on these results, durvalumab was recently granted ODD by the FDA for the treatment of ES-SCLC. Multiple ongoing trials are evaluating the efficacy of frontline pembrolizumab in combination with platinum/etoposide for patients with ES-SCLC.
Several other synergistic strategies are being investigated for their potential to enhance the efficacy of ICIs in SCLC. A phase I/II study is evaluating ROVA-T in combination with nivolumab or nivolumab and ipilimumab in patients with ES-SCLC. An early-phase trial is exploring tumor vaccination with TP53-DC vaccine in combination with nivolumab and ipilimumab in SCLC. Other strategies designed to increase tumor immunogenicity with targeted therapies, such as DNMT or PARP (poly-ADP ribose polymerase) inhibitors, in combination with ICIs are also being evaluated in early-phase studies.
Summary
In the past 2 decades, unprecedented advances in the understanding of the biology of lung cancer have revolutionized the diagnosis and treatment landscape of the disease, and ushered an exciting era of personalized medicine. TKIs and ICIs have changed the paradigm of treatment of advanced lung cancer with improved survival for a subset of patients. However, many patients do not respond and others develop resistance after an initial response. Comprehensive data including genomic, epigenomic, and cellular profiles of PMLs, primary and metastatic tumors, along with composition of the TME and host immune responses are being gathered throughout the course of the disease. Integration of this information will provide further insight into the mechanisms of tumorigenesis and resistance to therapy and establish a foundation for rational selection of personalized therapeutics. Furthermore, it is anticipated that the application of this comprehensive and integrative immunogenomic approach in pulmonary premalignancy will lead to the development of interventions that can potentially intercept cancer progression at the earliest stage of the disease.
Disclosure
S.M. Dubinett reports receiving a commercial research grant from Johnson & Johnson and is a consultant/advisory board member for LungLifeAI, Early Dx, Inc, the Johnson & Johnson Lung Cancer Initiative, and T-Cure Biosciences, Inc.
References
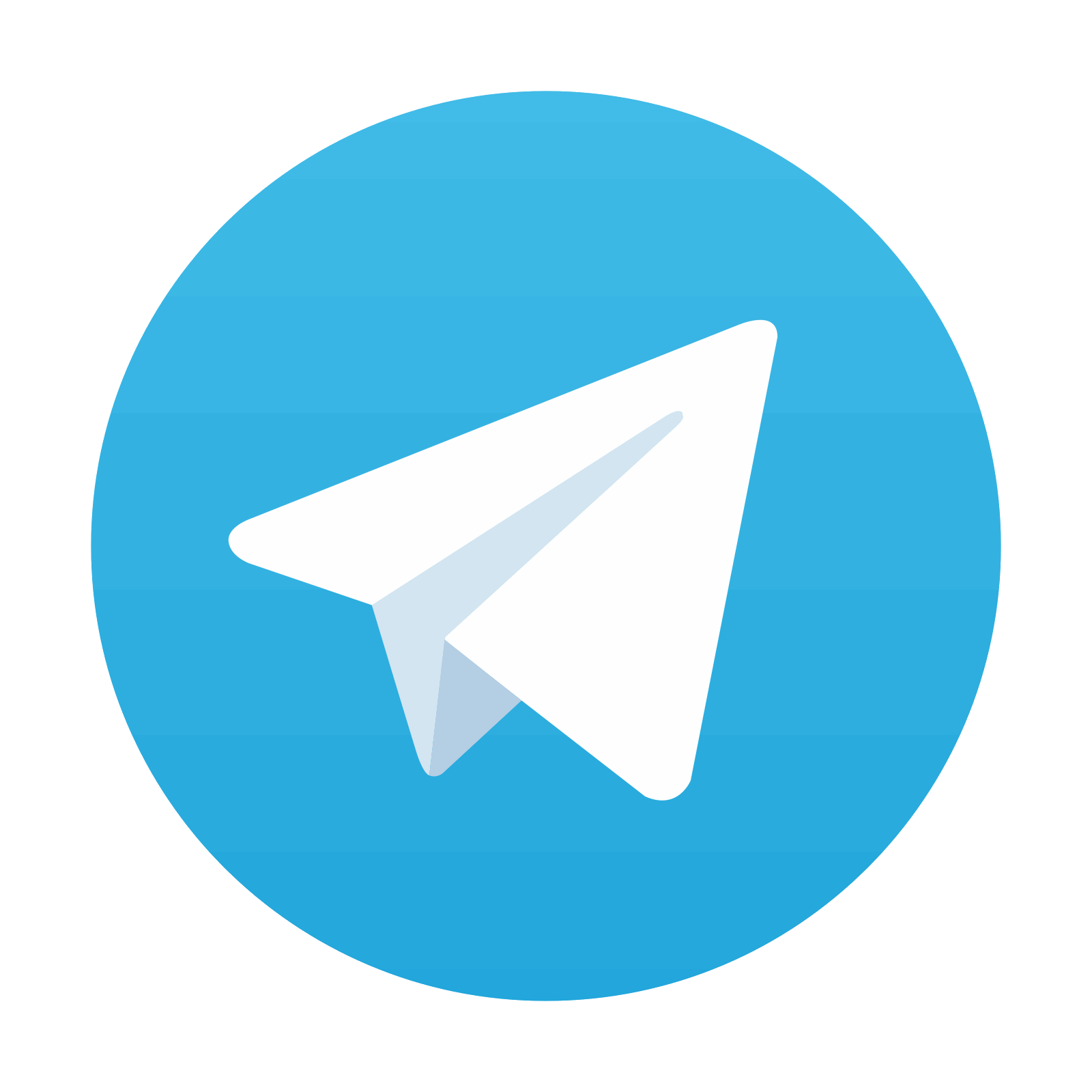
Stay updated, free articles. Join our Telegram channel

Full access? Get Clinical Tree
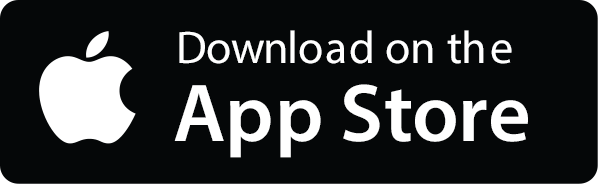
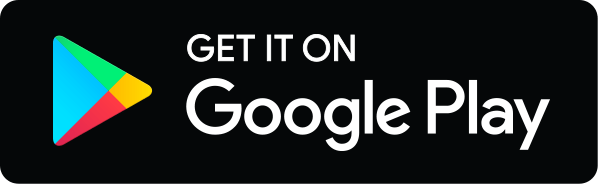
