A 29-year-old man was born a “blue baby” and found to have d-transposition of the great arteries (D-TGA). A balloon atrial septostomy (BAS) was performed as an infant, followed by the Mustard (atrial baffle) procedure. He did well for several years and did not require further surgical or percutaneous interventions. He was lost to follow-up for several years and ultimately presented to a local emergency room at 24 years of age, with atrial flutter and a rapid ventricular rate. He was electrically cardioverted back to sinus rhythm. At the time, an echocardiogram demonstrated mild systemic (right ventricular) dysfunction and concerns for narrowing of the superior vena cava (SVC) limb of the systemic venous baffle. Cardiac magnetic resonance imaging (MRI) subsequently demonstrated a mildly dilated and hypertrophied systemic right ventricle (RV) with mild global systolic dysfunction (estimated RV ejection fraction [EF] of 43%). The thin-walled left ventricle (LV) had normal size and function (LVEF of 63%). He was taken to the cardiac catheterization laboratory where SVC baffle limb stenosis was confirmed. A 36-mm max LD stent expanded to 34 mm was deployed to relieve the baffle stenosis. An electrophysiology (EP) study was also performed and revealed both atrial flutter and ventricular tachycardia. Given this, a transvenous implantable cardioverter-defibrillator (ICD) was placed for primary prevention of sudden cardiac death (SCD). He did well from 24 to 29 years of age with no major concerns and maintained regular follow-up with the Adult Congenital Heart Disease (ACHD) clinic. He maintained a regular job, got married, had two children, and exercised regularly. Closer to his 29th birthday, he noted that he was becoming “fatigued” with running 2 to 3 miles. He had rare episodes of palpitations associated with lightheadedness though he never had frank syncope or an ICD discharge. A Cardio-Pulmonary Exercise Test (CPET) was done to screen for desaturations and arrhythmias with exercise. This revealed a baseline oxygen saturation of 92% on room air with significant desaturation to 86% while running. There were no rhythm concerns. A repeat echocardiogram was done that showed a systemic venous baffle leak. He underwent cardiac catheterization with removal of the ICD leads, placement of a covered stent to relieve the baffle leak, and ICD lead replacement. Since this procedure, he has been doing well. He remains fully saturated, continues to work, and exercises often with no concerns.
This case highlights several important concepts for the long-term complications and management of adult patients who have had surgical palliation for D-TGA as an infant/child.
These include concerns for both atrial and ventricular arrhythmias including the need for ICD placement to prevent SCD.
These patients can also undergo both baffle leak and stenosis for which directed transcatheter interventions can be successfully performed in the cardiac catheterization laboratory.
Most importantly this case highlights the importance of long-term follow-up and management in patients with complex congenital heart disease like D-TGA, so they may continue to lead active lifestyles as manifested by this patient having a full time job, exercising, and spending quality time with his family.
Transposition of the great arteries (D-TGA) was first described by Scottish pathologist Matthew Baillie in 1797.
It is one of the most common forms of cyanotic congenital heart disease (CHD) and accounts for 5% to 7% of cardiac malformations.
There is a male preponderance of approximately 2:1 and a birth incidence of 20 to 30 per 100,000 live births.1
Inheritance is considered multifactorial. Like many other CHD lesions, there is no known clear genetic association.
Embryology likely involves abnormal persistence of the subaortic conus with resorption or underdevelopment of the subpulmonary conus (infundibulum). This abnormality aligns the aorta anterior and superior with the right ventricle during cardiac development.
D-TGA is an isolated defect in 90% of patients and is rarely associated with syndromes or extracardiac malformations.
Associated cardiac anomalies are common and most frequently include atrial septal defect (ASD), ventricular septal defect (VSD), pulmonary stenosis (PS), and coronary anomalies. While extracardiac anomalies are less common in D-TGA than in other CHD, 30% of neonates die within the first week of life without treatment.2
The definition of D-TGA varies among surgeons and cardiologists alike, however, it is generally agreed that it is a malformation in which the aorta arises from the morphologic right ventricle and the pulmonary artery (PA) arises from the morphologic left ventricle.
In the majority of patients, the anatomy is atrial situs solitus with atrioventricular concordance, d-looping of the ventricles, and ventriculoarterial discordance.2
In 95% of patients, the aorta is positioned anterior and to the right of the pulmonary artery,3 hence, the term “dextro” or “D-TGA” (Figure 5-1). As a result, the circulation is parallel instead of normal in series circulation.
In the absence of an intercirculatory shunt, especially an ASD, blood entering the systemic circulation remains deoxygenated and the infant will not survive.
In approximately one-third of patients with TGA, the coronary artery anatomy is abnormal, with a left circumflex coronary arising from the right coronary artery (22%), a single right coronary artery (9.5%), a single left coronary artery (3%), or inverted origin of the coronary arteries (3%) representing the most common variants.
Following are the primary anatomic subtypes:
D-TGA with intact ventricular septum
D-TGA with a VSD
D-TGA with VSD and left ventricular outflow tract obstruction
D-TGA with VSD and pulmonary vascular obstructive disease
The postnatal clinical features of D-TGA are based on the degree of mixing between the 2 parallel circulations and the presence of other cardiac anomalies.
Although, most patients present with cyanosis during the newborn period, the severity is based on the amount of intercirculatory mixing, which is particularly affected by the presence and size of an ASD.
The following are 3 common anatomic sites for mixing of oxygenated and deoxygenated blood in D-TGA:
Atrial septal defect (most important)
Ventricular septal defect
Patent ductus arteriosus (PDA)
Oxygen saturations and the degree of hypoxemia are largely based on the amount of this intercirculatory mixing that occurs through the intracardiac and extracardiac connections.
The goal of optimizing intercirculatory mixing is achieved by balancing the effective pulmonary and systemic blood flows. This mixing occurs most efficiently at the level of the atria (eg, ASD) as lower pressure differences allow for bidirectional flow across the atrial septum (eg, flow in both systole and diastole).
Mixing at other sites (eg, VSD or PDA) is more limited due to the presence of larger pressure gradients, which typically direct blood in only one direction. Shunting of blood preferentially toward the systemic or pulmonary circulation tends to result in clinical deterioration.
Rarely, infants with an intact atrial septum can develop cyanosis within the first hours of life. Patients with a VSD or a large PDA, on the other hand, may present between 4 and 8 weeks of life with symptoms of heart failure, such as tachycardia, tachypnea, diaphoresis, and poor feeding. Cyanosis is not affected by exertion (eg, crying or feeding) or the use of supplemental oxygen.
Reverse differential cyanosis with higher postductal saturations than preductal saturations can be found in patients with pulmonary hypertension, coarctation of the aorta, or interruption of the aortic arch. This is due to the right-to-left ductal shunting of blood into the descending aorta that is more fully saturated than that entering the ascending aorta.
A small percentage of infants with D-TGA (often with a VSD) develop accelerated pulmonary vascular obstructive disease and progressive cyanosis despite surgical repair or palliation. Long-term survival in this subgroup is particularly poor.
Without surgical treatment the majority of infants with D-TGA die by 1 year of age. Therefore, adult patients presenting to for long-term care have had corrective surgery in infancy or childhood or have had multiple surgical procedures during the course of their life.
From the time that a diagnosis of D-TGA is suspected, patients are managed with intravenous prostaglandin E1 (PGE1) in an effort to maintain or restore circulation between the 2 circuits.
Side effects of PGE1 include apnea, hyperthermia, muscular twitching, and flushing, especially with higher dosages.
Once the oxygen saturation improves, the dosage of the PGE1 can often be reduced.
The Rashkind procedure (Figures 5-2A and 5-2B) was developed in the 1966 by William Rashkind for the initial management of the neonate with D-TGA and restrictive intra-atrial communication.4 In this procedure, a catheter is introduced via femoral or hepatic venous access and positioned across the atrial septum into the left atrium. A balloon is then inflated and drawn back abruptly into the right atrium to create an atrial septostomy and improved circulation between the 2 circuits.
As the trend toward earlier definitive repair has continued, Rashkind balloon atrial septostomy is now most often performed in infants with profound hypoxemia or in cases in which surgery must be delayed.2, 5
In 1950, American surgeons Alfred Blalock and C. Rollins Hanlon introduced the Blalock-Hanlon atrial septectomy in which an atrial septal defect was created by excising the posterior aspect of the interatrial septum.6 Although a common palliative procedure in the past, it is now rarely performed.
The first definitive surgeries for D-TGA were described by Åke Senning in 1959 and William Mustard in 1964 (Figure 5-3).7, 8
The “Senning” and “Mustard” procedures were considered “atrial switch operations” and allowed for blood to flow in series by baffling systemic venous blood to the left ventricle and pulmonary venous blood to the right ventricle (Figures 5-4A and 5-4B).
The primary difference between the procedures is that the Senning procedure used the right atrial wall to create the baffle whereas the Mustard procedure employed autologous pericardium or synthetic material (eg, Dacron).
These operations were usually performed between 1 month and 1 year of life and, until the 1990s when the arterial switch repair became more widely used, had an operative mortality of as low as 1%.9, 10
FIGURE 5-3
Atrial switch repair (Mustard procedure). Systemic venous blood (blue) returns from body and travels to the lungs (black arrows) via the LV. Pulmonary venous blood (red) returns from the pulmonary bed and travels to the systemic circulation (yellow arrows) via the RV. Ao, aorta; IVC, inferior vena cava; LA, left atrium; LV, left ventricle; MPA, main pulmonary artery; RA, right atrium; RV, right ventricle; SVC, superior vena cava SVB, systemic venous baffle.

FIGURE 5-4A and 5-4B
Atrial switch repair (Mustard procedure). A. The opened pulmonary venous atrium in a heart after a Mustard atrial switch operation. The red curved arrows indicate flow from the right (RPV) and left pulmonary veins toward the tricuspid valve (TV) and right ventricle. The dashed blue curved arrows indicate flow from the superior vena cava (SVC) and inferior vena cava (IVC) behind the limbs of the baffle toward the mitral valve (MV) and left ventricle. B. The opened systemic venous atrium in the same heart showing the other side of the baffle with SVC and IVC flow (blue curved arrows) toward the mitral valve (MV). The left pulmonary veins (LPV) are seen posterior to the systemic venous atrium. (Reproduced with permission from Stephen P. Sanders, MD, Professor of Pediatrics [Cardiology], Harvard Medical School; Director, Cardiac Registry, Departments of Cardiology, Pathology, and Cardiac Surgery, Children’s Hospital Boston, Boston, Massachusetts.)

In 1975, Jatene et al successfully performed the first anatomic repair (arterial switch repair) of D-TGA.11 This procedure involves transecting the aorta and pulmonary arteries at the level above the sinuses and positioning them above the left and right ventricle, respectively. The coronary arteries, along with a small area of surrounding tissue (called a “button”), are also detached from the aorta and sutured into place on the neoaorta (Figures 5-5A and 5-5B).
Though technically challenging, the arterial switch operation (ASO) allows the left ventricle to become the systemic ventricle. Indeed, Mustard first conceived of, and attempted, the arterial switch for D-TGA in the early 1950s. His few attempts were unsuccessful owing to technical difficulties posed by the translocation of the coronary arteries, and the idea was abandoned.
The ASO is now the most widely accepted operation for D-TGA and is typically performed within the first 2 weeks of life.3
FIGURE 5-5A and 5-5B
Arterial switch operation (ASO). A. Left lateral view of the great arteries after an arterial switch operation. The main pulmonary artery (MPA) is anterior to the aorta (Ao). The left pulmonary artery (LPA) has been divided to show the inside of the aorta. The suture line (blue sutures) is evident between the ascending Ao and the pulmonary root (?). The right coronary artery ostium (RCA) with surrounding button of aortic wall is seen on the right side of the aorta. B. A frontal view of the same heart showing the MPA and branches. The right pulmonary artery (RPA) passes posteriorly between the Ao and the superior vena cava (SVC). The divided LPA is to the left of the Ao. (Reproduced with permission from Stephen P. Sanders, MD, Professor of Pediatrics [Cardiology], Harvard Medical School; Director, Cardiac Registry, Departments of Cardiology, Pathology, and Cardiac Surgery, Children’s Hospital Boston, Boston, Massachusetts.)
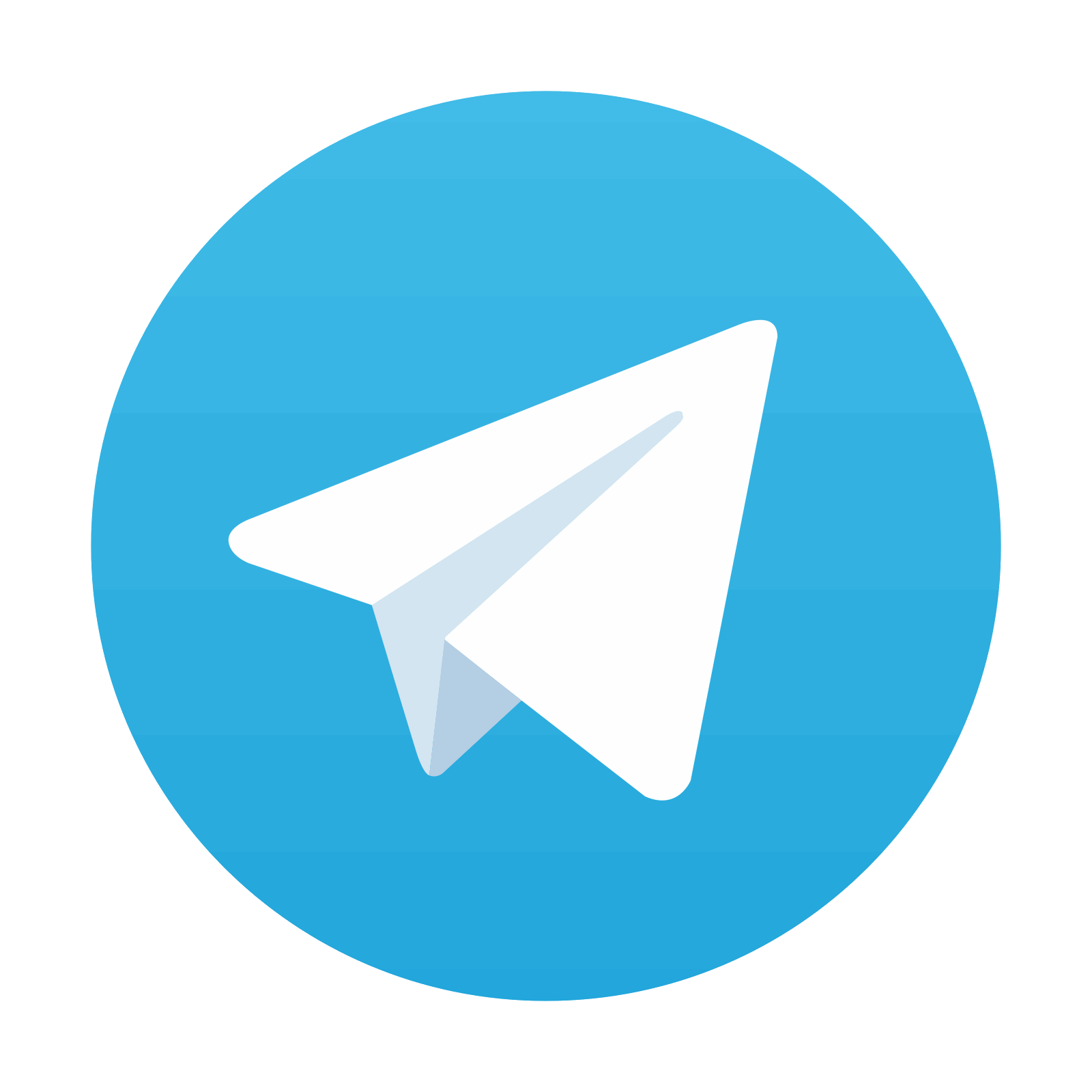
Stay updated, free articles. Join our Telegram channel

Full access? Get Clinical Tree
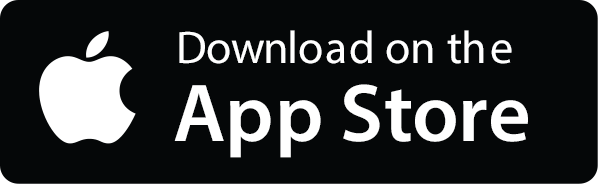
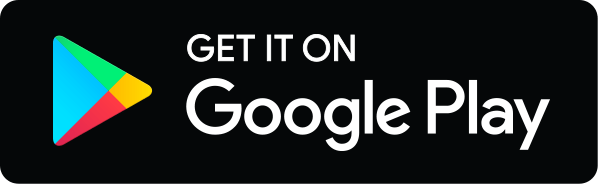
