A 20-year-old man with a single morphologic left ventricle due to tricuspid atresia and ventricular inversion with transposed great arteries, with transposed great arteries presents with stunted growth and maturation. He initially had a pulmonary artery band (Figure 7-1) placed when he was 2 months old with an atrial septectomy at 9 months. At age 3 years he underwent a surgical Fontan palliation, which involved redirection of the superior vena cava (SVC) and inferior vena cava (IVC), by way of a lateral tunnel, to the right pulmonary artery, confluent with the left. The main pulmonary artery (MPA) was excised. Therefore, systemic venous flow was redirected to passively flow to the right and left pulmonary vascular beds, bypassing the heart entirely (Figure 7-2). He had an uneventful recovery in early childhood until he was diagnosed with protein-losing enteropathy (PLE) at the age of 6 years. Subsequently, at age 10, further surgery was done to remove the lateral tunnel and replace this with an extracardiac conduit between the IVC and pulmonary artery. Despite surgical revision and medical therapy, he continued to have evidence of PLE complicated by a small stroke and evidence of mild liver fibrosis. He was started on warfarin. By age 12, he had evidence of delayed maturation and stunted growth (Figure 7-3), as a complication of PLE. He had chronic hypoproteinemia, hypocalcemia, and hyponatremia. Measured testosterone and growth hormone levels were normal. He was considered for heart transplantation, but instead medical therapy was continued, including subcutaneous heparin, testosterone, and spironolactone.
FIGURE 7-1
Illustration depicting a pulmonary band placed in the patient’s anatomy of tricuspid atresia with transposed great arteries. Ao, aorta; LV, left ventricle; PA, pulmonary artery; PV, pulmonary vein; RA, right atrium; RV, right ventricle. (Copyright © McGraw-Hill Education, Photographer: Dr. Laurie Armsby. Modified with permission from Dr. Laurie Armsby.)

FIGURE 7-2
A. Illustration depicting a lateral tunnel Fontan connecting the inferior vena cava (IVC) and superior vena cava (SVC) to the pulmonary artery which has been disconnected from the pulmonary valve in the patient’s anatomy of tricuspid atresia with transposed great arteries. Ao, aorta; LA, left atrium; LV, left ventricle; PA, pulmonary artery; PV, pulmonary vein; RA, right atrium; RV, right ventricle. B. Transthoracic echocardiogram showing a subcostal view of the inferior vena cava (IVC) being directed into the lateral tunnel through the right atrium. SVC, superior vena cava. (Copyright © McGraw-Hill Education, Photographer: Dr. Laurie Armsby. Modified with permission from Dr. Laurie Armsby.)

Now at age 20, he is asymptomatic with no limitations in exercise capacity or palpitations. He is attending college. His blood pressure is 86/60 mm Hg, heart rate is 87 bpm, and oxygen saturation is 94% on room air. He is 59-in tall and weighs 40 kg. His body surface area was only 1.29 m2, < 3rd percentile. He appears prepubescent. He had a loud systolic murmur at the base of the heart. There was no ascites or edema. The lower extremities were hyperpigmented with varicosities, both visible and palpable. Measured hemoglobin was 17.0 g/dL, total protein was 3.8 g/dL, albumin was 1.6 g/dL. Liver and kidney function tests are normal. He is in normal sinus rhythm. An echocardiogram showed a single ventricle with normal systolic function. There was no mitral valve regurgitation. There was stenosis through the ventricular septal defect (VSD) (in essence subaortic stenosis since this is the flow pathway to the hypoplastic right ventricle and aorta) with a peak gradient of 3.2 m/s. Bone densitometry measured 0.53 g/cm2 of the lumbar region and 0.47 g/cm2 of the femur (T scores severely low, –5.1 and –3.7, respectively). Measured peak oxygen consumption with exercise was 20.4 mL/kg/min (43% of predicted), despite his self-expressed lack of symptoms. Peak heart rate with exercise was 100 bpm, and peak oxygen saturation was 88%. Hepatic ultrasound showed no cirrhosis, but liver biopsy revealed mild centrilobular and portal fibrosis. By catheterization, his systemic venous pressure was 10 mm Hg, pulmonary capillary wedge pressure was 9 mm Hg. There was a 45-mm Hg systolic gradient between the left ventricle and the ascending aorta. His cardiac index was 2.3 L/min/m2 (Figure 7-4).
FIGURE 7-4
Diagram illustration hemodynamic measurements obtained at the time of the patient’s cardiac catheterization. Of particular note is the 45-mm Hg systolic gradient between the left ventricle (120/5) and the right ventricle (74/6) demonstrating subaortic stenosis at the level of the bulboventricular foramen and the mildly elevated systemic venous pressure of 10 mm Hg. (Copyright © McGraw-Hill Education, Photographer: Dr. Laurie Armsby. Modified with permission from Dr. Laurie Armsby.)

For individuals born with complex congenital heart defects constituting a single ventricle, such as this patient, the Fontan procedure, a term referring to any variation of procedures used to surgically revise the circulation such that systemic venous drainage is directed to the pulmonary vasculature passively as opposed to via a cardiac pumping chamber, has revolutionized survival since its introduction in 1971. However, survival from the Fontan palliation and its many variations comes at a cost of long-term chronically elevated central venous pressure, with at times drastic multiorgan consequences.
This young adult has what is considered to be a failing Fontan as evidenced by his complications of PLE, arrested growth, poor exercise capacity (objectively measured), and liver disease. The forward flow through his Fontan/pulmonary circuit is inadequate thereby resulting in passive venous congestion which is linked to PLE (enteric venous congestion) and liver disease. Venovenous collaterals form, bringing desaturated blood to the systemic ventricle. Furthermore, inadequate preload to his single ventricle limits cardiac output. Both these factors lower his resting oxygen saturation, and raise his hemoglobin.
Surgical and interventional techniques in his case had been performed to alleviate any obstruction or excess flow through his Fontan circuit and promote forward flow, and medical therapy for PLE has also been extensive. However, these measures have not been able to prevent steady loss of protein with subsequent adverse effects including poor somatic growth, lack of sexual development, and bone disease. He continued to have moderate subvalvar aortic stenosis at the level of the bulboventricular foramen (Figure 7-5) which is difficult to address surgically given a perceived high operative risk given the above comorbidities.
Medical therapies have been aimed at reducing his pulmonary vascular resistance and reducing afterload for his failing ventricle. Future options would include cardiac transplantation, which has a reported high mortality in the perioperative period, but could be curative of his PLE and offer favorable long-term survival.
Such patients are often faced with a clinical dilemma; continue as he is doing in his fairly asymptomatic but limited state, or face high-risk transplantation in the hopes of favorable long-term outcome.
FIGURE 7-5
Illustration highlighting the restrictive bulboventricular foramen (yellow circle) that results in subaortic stenosis and outflow obstruction. Ao, aorta; IVC, inferior vena cava; LA, left atrium; LV, left ventricle; PA, pulmonary artery; RA, right atrium; RV, right ventricle; SVC, superior vena cava. (Copyright © McGraw-Hill Education, Photographer: Dr. Laurie Armsby. Modified with permission from Dr. Laurie Armsby.)

The true prevalence is unknown, but a rate reported in clinical studies was approximately 1.5%.
The incidence of univentricular physiology is thought to be 0.05 to 0.1 per 1000 live births.1
No sex dominance is known: men and women are almost equally affected.
There are many congenital cardiac anomalies that are palliated with multistage repair and ultimately the Fontan operation, which most commonly include (but not limited to) tricuspid atresia (22%), hypoplastic left heart syndrome (21%), and double inlet left ventricle (15%)1, 2, 3 as listed below (Table 7-1).
Anatomy | Incidence Rate (per 1000 live births) |
---|---|
Hypoplastic left heart syndrome | 0.21-0.28 |
Pulmonary atresia | 0.069-0.074 |
Tricuspid atresia | 0.057 |
Unbalanced atrioventricular septal defect | Unknown |
Double outlet right ventricle (unseptatable) | 0.03-0.2 |
Double inlet left ventricle | Unknown |
Heterotaxy | 0.05 |
Miscellaneous unseptatable defects (multiple ventricular septal defects, straddling cords, etc) | Unknown |
In single ventricle physiology, there is complete mixing of pulmonary (desaturated) and systemic (saturated) blood resulting in cyanosis.
Some very rare anatomies truly have 1 ventricle, whereas the majority of patients have 2 morphologically distinct ventricles though there is functionally only one chamber. This may result from 1 ventricle being hypoplastic or from the lack of a functional separation between the ventricles. Ultimately, some anatomies are not amenable to surgical separation (or septation) into 2 ventricles for various reasons and these patients are relegated to the surgical pathway of single ventricle palliation.
There may be inadequate blood flow to the pulmonary or systemic circulations in unbalanced anatomies.
Even with adequate blood flow to both circulations, in the absence of some degree of restriction to pulmonary blood flow, irreversible damage to the pulmonary vasculature with muscularization of pulmonary arterioles can lead to elevated pulmonary vascular resistance and Eisenmenger syndrome.
Surgical palliation in the single ventricle is aimed toward separating the pulmonary and systemic circulations through a variety of staged surgeries resulting in what is commonly referred to as “Fontan” physiology.
Most single ventricle anatomies are often readily detected before birth with fetal echocardiography.
With the introduction of routine pulse oximetry of infants in recent years, some otherwise asymptomatic infants have been identified via screening.
Ultimately the diagnosis is confirmed and refined using transthoracic echocardiography and cross-sectional imaging (computed tomography [CT] or magnetic resonance imaging [MRI]), which can provide useful supplemental information.
On occasion, cardiac catheterization is needed to further define anatomy and for surgical planning during the diagnostic period. It is particularly helpful to determine pulmonary blood flow in patients with inadequate antegrade flow through the pulmonary valve or with pulmonary atresia. These hemodynamic data inform decisions about surgical intervention.
Adult patients with single ventricular physiology should be followed by an adult congenital heart disease specialist with periodic visits which should include symptom assessment with functional classification, growth charting when appropriate, weight and muscle mass assessment in adults, blood pressure, oxygen saturations, imaging, cardiac catheterization, and laboratory evaluation when needed.
It can be difficult to determine the important clinical marker of exercise tolerance in a patient chronically compensating for their single ventricle physiology. The case discussed earlier is exemplary—the patient has no subjective symptoms yet his measured oxygen consumption is very low.
Particularly, it is known that patients who have undergone Fontan palliation have decreased exercise tolerance and therefore, exercise testing with measurement of oxygen consumption is a good objective clinical marker to follow in this patient population.5, 6
Transthoracic echocardiography provides good information in this patient population. Key findings are patency and velocity of the Fontan and Glenn circuits, patency of the atrial septal defect, atrial size and presence of intracardiac thrombi, atrioventricular valve regurgitation, outflow tract obstruction, and ventricular size and function among other individual questions. These pathways are not always well visualized.
Imaging often relies on knowledge of the specific surgical interventions performed in the individual being imaged.
Cross-sectional imaging such as cardiac MRI and cardiac CT provides useful information in single ventricle patients, particularly in those with anterior systemic ventricles that are difficult to image with echocardiography.
MRI also provides useful information in quantifying ventricular volume, stroke volume, and ejection fraction (EF). New techniques which may be informative for this patient population in the future include fibrosis index and 4-dimensional flow imaging to determine caval blood flow distribution. As with echo, the best studies will be directed by an experienced imager with knowledge of the patient-specific procedures.
Cardiac catheterization provides hemodynamic assessment that is difficult or impossible to obtain via other techniques, particularly, diastolic pressure measurements, central venous pressures (Fontan circuit pressures), pulmonary vascular resistance, and the presence of right-to-left shunts.
Also, interventions such as coiling of venovenous and aortopulmonary collaterals, dilation of branch pulmonary stenosis, re-coarctations, closure of baffle-leaks, and refenestration or closure of Fontan fenestration can be undertaken during catheterization to optimize the Fontan circuit.
Routine rhythm assessment may be useful to evaluate for the presence of clinically silent or symptomatic atrial or junctional rhythms, resting heart rate, and chronotropic response to exertion.
Fontan patients on amiodarone are at increased risk for thyroid dysfunction. Furthermore, the myocardial depression or tachycardia, associated with hypo- or hyperthyroidism, respectively, are deleterious to single ventricle patients.
Thyroid-stimulating hormone (TSH) and free thyroxine (T4) should be followed intermittently, particularly in those patients who are symptomatic.
Poor forward flow through the Fontan circuit results in hepatic congestion from hepatic venous hypertension, inflammation/transaminitis, fibrosis, and ultimately dysfunction and failure. Therefore, assessing liver inflammation and function should be followed with serial liver profiles and coagulation panels as well as imaging.
Some commercially available biomarker assays for fibrosis have been considered for longitudinal follow-up. Frequency and modality of liver imaging have not been routinely established.
Liver biopsy should also be considered on occasion, and can often be combined in some institutions with cardiac catheterization.
Fontan patients with PLE often have gastrointestinal fluid losses and may have symptoms of diarrhea and weight loss prior to complications of hypoproteinemia.
Fecal α1 antitrypsin is elevated in patients with PLE. Patients may also experience bloating, distention, and abdominal pain secondary to hepatic distention or ascites.
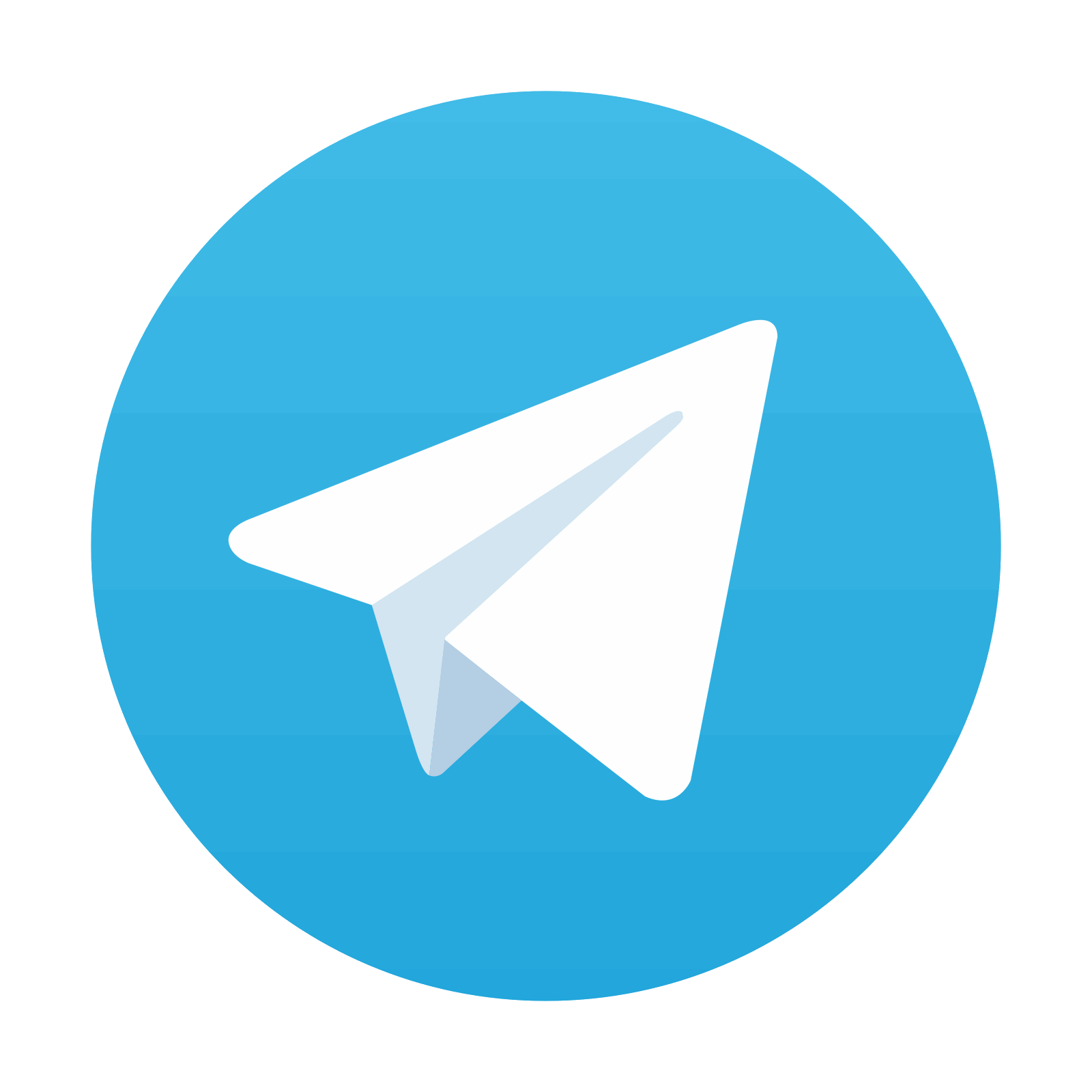
Stay updated, free articles. Join our Telegram channel

Full access? Get Clinical Tree
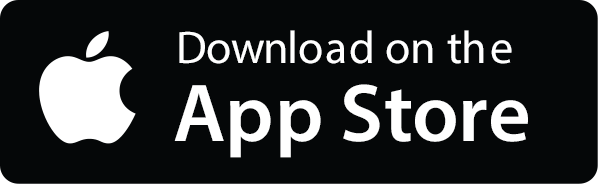
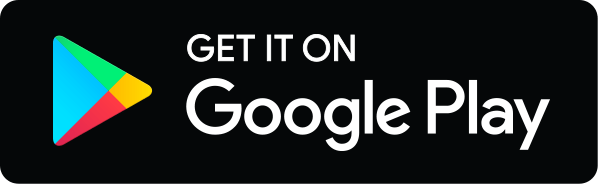